Assessment of ultrasound shear wave elastography within muscles using different region of interest sizes, manufacturers, probes and acquisition angles: an ex vivo study
Introduction
Shear wave elastography (SWE) is a promising modality for evaluating the elasticity of the musculoskeletal system. Shear wave speed (SWS) is closely related to tissue stiffness, with faster propagating shear waves representing stiffer tissue (1,2). The feasibility of SWE has been confirmed in the diagnosis of breast (3), liver (4), thyroid (5), and prostate (6) lesions. However, the role of SWE in the evaluation of musculoskeletal diseases still requires further research, and more studies are needed to establish a standard protocol. Young’s modulus can be estimated from SWS, assuming a linear isotropic and homogeneous tissue model. The relationship between Young’s modulus and SWS is E = 3ρC2. In this equation, E represents Young’s modulus, 3 is a constant related to Poisson’s ratio for strain, ρ is tissue density (assumed to be 1 g/cm3), and C is the SWS. Therefore, it is recommended that SWS results are recorded in m/s, considering the anisotropy of muscles (7,8). Pathological changes, such as muscular dystrophy (9), spasticity (10), and inflammation (11), can change the mechanical properties of muscles. The technology of SWE can non-invasively evaluate tissue stiffness qualitatively and quantitatively (12-14) and has the potential to assess muscle properties effectively. However, the application of SWE to the musculoskeletal system must overcome various structural and anatomical problems, such as tissue heterogeneity due to the presence of tendon and aponeurotic structures, muscle contraction and relaxation, location variability, and the complexity of adjacent structures (15,16). All of these features have been shown to influence SWE readings (17-19), further complicating in vivo evaluation of muscle elasticity.
Various factors, such as the region of interest (ROI) size, machines from different manufacturers, probes of different frequencies, and probe orientation, all exert influence on SWS muscle measurement. For many machines, there are various ROI sizes for selection. Recently, 2 studies focused on the effect of ROI on muscle SWS and both reported that ROI size (ROI sizes 28–707 mm2 and 15–200 mm2, respectively) had little influence on SWS (20,21). Most studies have focused on whether ROI size affects SWS, and almost no studies have focused on the reproducibility of different ROI sizes, which has particular significance for follow-up evaluation of lesions. As far as we know, only a few studies have evaluated the variability and repeatability of SWS measured with different ultrasound elastography machines (22,23). A phantom study demonstrated SWS variability in machines from different manufacturers, indicating a considerable difference in SWS depending on the type of machine used (24). However, it is not appropriate to extrapolate conclusions obtained from phantom studies to the musculoskeletal system. Furthermore, few studies have evaluated the variability of SWS acquired from different machines using different probes within healthy ex vivo muscles, which is vital for results comparison in clinical practice and future studies. The human body has pennate muscles, such as the gastrocnemius and vastus lateralis, that require particular attention because the angle between the muscle fiber and the long axis of the muscle is variable. Several previous studies have also indicated variabilities regarding probe orientation. These studies showed that the best reproducibility was associated with the longitudinal plane relative to the muscle fibers in normal skeletal muscles (19,21). Miyamoto et al. reported that the reliability of shear modulus measurements remains high if the angle between the probe axis and the orientation of the muscular fibers is below 20° (25). To date, there has been no study revealing the feasible angle range relative to the fascicle direction in the same plane.
Further standardization of the acquisition process is essential for improving measurement accuracy. In this work, 3 professional sonographers acquired the SWS of isolated muscles. The purpose of this study was to evaluate the SWS variations in ex vivo muscles with different ROI sizes, machines, probes, and acquisition angles. Furthermore, the intra- and inter-operator reproducibility of the mean SWS under different conditions was investigated. We present the following article in accordance with the Guidelines for Reporting Reliability and Agreement Studies (GRRAS) reporting checklist (available at https://qims.amegroups.com/article/view/10.21037/qims-21-1072/rc).
Methods
Materials
A total of 80 samples (20 for the ROI comparison, 20 for the machine comparison, 20 for the probe comparison, and 20 for the angle comparison) of fresh, ex vivo pork tenderloin muscle with clear fiber provided by the same slaughterhouse were obtained. These 80 specimens were selected based on our pre-test experience. They were removed from the pig bodies 6–15 h before use and measured at 20 ℃ temperature, with an average size of [10–15] cm × 7 cm × 7 cm.
Our research was an exploratory study; it did not require a large sample size, so we chose a sample size of 20. The small number of samples not only saved on staffing, financial resources, and time, but also guaranteed the accuracy and reliability of the research results. To minimize the elastic changes of specimens over time, we selected 20 separate specimens for each of the different item comparisons.
Ultrasound machines
We used 3 two-dimensional (2D) SWE machines from different manufacturers: the Aixplorer system (SuperSonic Imagine, Aix-en-Provence, France; machine I) equipped with SL 10-2 and SL 15-4 probes (probes A and B), the Acuson S3000 (Siemens Healthcare, Erlangen, Germany; machine II) equipped with a 9L4 probe, and the Resona 7 (Mindray, Shenzhen, China; machine III) equipped with an L14-5WU probe.
The Aixplorer system operates with a spatial resolution of 1 mm × 1 mm in elastography (7). The SWE uses the acoustic radiation force induced by ultrasound beams to stimulate the target tissues. A rectangular translucent color window of 2 cm × 2.5 cm with the ROIs placed at the center of the box at a depth of 2 cm with a couplant of 2 mm was chosen as the acquisition method for all machines. No air bubbles were allowed in the couplant. The Aixplorer system penetration (Pen) condition was chosen. The values were presented in units of m/s (26). During imaging, SWS measurement should avoid “fence” artifacts, areas of signal void, and areas of too high or too low signal to minimize inaccurate measurements. To prevent measurement inaccuracy when evaluating the muscle elasticity, we avoided placing the ROI on the edge of the color window. The muscle samples were placed between the 2 fixators during the examination to maintain the shape, but no pressure was applied. Measurements were repeated 3 times for each condition, and the probe was removed and replaced each time.
Operators
The study was conducted by 3 board-certified sonographers (operators A, B, and C) with more than 3 years’ experience in ultrasound elastography. Before the experiment, all operators were trained on the specimens by completing at least 30 successful experimental operations. During the examination, the operator placed the probe along the direction of the muscle fiber. Each operator completed the SWE procedures independently and was blind to the measurement results of the other operators. The measurement order of the 3 operators was random.
ROI
The SWE measurements were acquired using 20 muscle samples with machine I equipped with an SL10-2 linear probe. Circular ROIs of 1, 3, 5, and 10 mm diameter were chosen. Readings were repeated 3 times for each ROI size by each operator, and the probe was removed and replaced each time. The SWE procedures were performed independently by operators A, B, and C.
Different SWE systems
The 3 machines from different manufacturers
We used 3 different machines, including the Aixplorer system equipped with an SL 10-2 probe, the Acuson S3000 equipped with a 9L4 probe, and the Resona 7 equipped with an L14-5WU probe.
A circular ROI with a diameter of 1 mm was selected for machines I and III, while the ROI size was an unchangeable rectangle in machine II (1 mm × 1 mm in size), as shown in Figure 1.
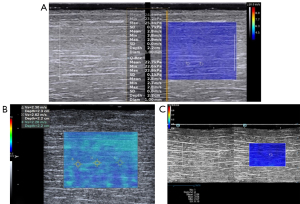
The SWS was acquired from 20 muscle samples using the 3 machines consecutively. The measurement order of the 3 machines for each sample was random. Each sample measurement took about 30 min, and the probe was placed at the same position each time. A marker was used to record the specific measurement location of each sample on the surface of the muscle. Measurements were repeated 3 times. The time interval between the 3 measurements was 8–10 min.
The 2 probes of different frequencies
The SWS was acquired from another 20 muscle samples using 2 probes, SL 10-2 and SL 15-4 (with center frequencies of 6 and 8.5 MHz, respectively) with the SuperSonic Aixplorer, as illustrated in Figure 2. Similarly, each sample measurement took 30 min to complete.
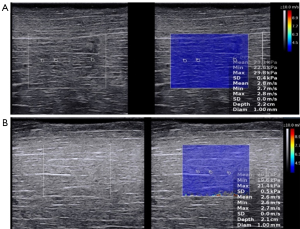
Operator A conducted these measurements.
Angles
A total of 20 isolated muscle samples were selected to assess the influence of the acquisition angle on muscle SWS using machine I equipped with an SL 10-2 probe and a 5 mm ROI diameter. A depth of 2 cm was chosen as the acquisition method for all angles. The total time for each muscle measurement was no more than 60 min. We used “α” to represent the angle between the probe plane and the muscle surface (Figure 3). In the present study, 0° indicated that the probe plane was parallel to the muscle surface. During the examination, the probe was always placed along the direction of the muscle fiber. Measurements were acquired using 9 different angles: 0°, 5°, 10°, 15°, 20°, 25°, 30°, 35°, and 40° at the same position. When the probe was positioned obliquely to the muscle surface, there was a sufficient amount of coupling agent and no bubbles (Figures S1,S2). No pressure or deformation of the muscle tissues occurred during operation. For each angle, measurements were repeated 3 times. Operator A conducted the above measurements.
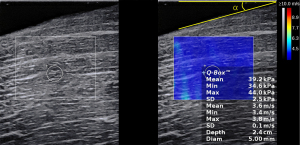
Data and statistical analysis
The software SPSS 22.0 (IBM Corp., Armonk, NY, USA) was used for statistical analysis. All data were presented as the mean ± standard deviation (M ± SD), and P value <0.05 was considered statistically significant. For the comparison of variance in the different measurement conditions, the coefficient of variation (CV) was calculated by applying the equation: CV = standard deviation/mean value (27). The larger the CV value, the lower the reliability and repeatability of each measurement. The Kolmogorov-Smirnov test was used to check if the data were normally distributed. The test for homogeneity of variance was applied to check for differences in variance. Analysis of variance (ANOVA) was used to compare the data from the different ROIs and machines. The Kruskal-Wallis test was used to compare the data from the different acquisition angles. A t-test was performed to compare SWS between the 2 different probes. For the subgroup analysis, Tamhane’s T2 post hoc test was used to compare SWS between the different machines and angles. The repeatability and reproducibility of the measurements were quantified using intra-class correlation coefficients (ICC) (two-way mixed effect model). The ICC values were interpreted as follows: 0.00–0.20 for poor agreement, 0.21–0.40 for fair agreement, 0.41–0.60 for moderate agreement, 0.61–0.80 for substantial agreement, and >0.80 for almost perfect agreement (21). The ICC and 95% confidence interval (CI) were used to evaluate the intra- and inter-operator consistency.
Results
Results obtained with different ROI sizes
The SWS was successfully acquired from 20 muscle samples. The Kolmogorov-Smirnov test showed all P values were >0.05, indicating that the data were normally distributed. The ANOVA results revealed that SWS did not differ substantively by ROI size (Table 1). The degrees of intra- and inter-operator agreement under each ROI size were almost perfect (ICC, >0.80) except for the 1 mm ROI diameter in the inter-operator agreement (Table 2). Figure 4 shows the SWS comparisons under the different ROI sizes by the 3 operators.
Table 1
ROI diameter (mm) | SWS (M ± SD) (m/s) with different ROI diameter | |||
---|---|---|---|---|
A | B | C | P value | |
1 | 4.75±0.58 | 4.35±0.46 | 4.42±0.39 | 0.036 |
3 | 4.60±0.60 | 4.39±0.52 | 4.50±0.36 | 0.464 |
5 | 4.63±0.60 | 4.47±0.54 | 4.45±0.40 | 0.521 |
10 | 4.62±0.58 | 4.51±0.54 | 4.52±0.38 | 0.752 |
P value | 0.874 | 0.778 | 0.865 |
The ANOVA test showed no significant differences among means of any of the analyzed variables; A, B, and C represent the 3 operators. P<0.05 was considered statistically significant. ROI, region of interest; SWS, shear wave speed; M ± SD, mean ± standard deviation; ANOVA, analysis of variance.
Table 2
ROI diameter (mm) | Intra-operator ICC (95% CI) | Inter-operator ICC (95% CI) | ||
---|---|---|---|---|
A | B | C | ||
1 | 0.96 (0.91–0.98) | 0.98 (0.95–0.99) | 0.91 (0.81–0.97) | 0.74 (0.42–0.89) |
3 | 0.92 (0.83–0.97) | 0.98 (0.95–0.99) | 0.91 (0.81–0.97) | 0.88 (0.73–0.95) |
5 | 0.91 (0.81–0.96) | 0.98 (0.95–0.99) | 0.95 (0.89–0.98) | 0.89 (0.75–0.96) |
10 | 0.92 (0.83–0.97) | 0.98 (0.96–0.99) | 0.96 (0.91–0.98) | 0.85 (0.68–0.94) |
A, B, and C represent the 3 operators. ROI, region of interest; ICC, intra-class correlation coefficients; CI, confidence interval.
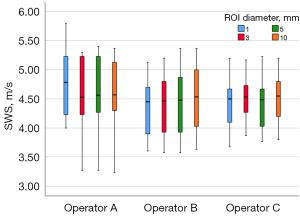
Comparison of different SWE systems
The SWS was successfully acquired from 20 muscle samples for the comparison of SWS of different machines and probes.
Tables 3,4 present the SWS results obtained using the 3 different machines and the 2 different frequency probes. Figure 5 shows the SWS distribution using the 3 different machines.
Table 3
Machines | SWS (M ± SD) (m/s) | P value | CV | Intra-system ICC (95% CI) | Inter-system ICC (95% CI) |
---|---|---|---|---|---|
Machine I | 3.24±0.18 | <0.001* | 0.06 | 0.64 (0.29–0.84) | 0.05 (0–0.57) |
Machine II | 2.51±0.16 | 0.06 | 0.69 (0.42–0.84) | ||
Machine III | 3.51±0.27 | 0.08 | 0.61 (0.25–0.82) |
P<0.05 was considered statistically significant. *, denotes a significant difference between groups (P<0.05). Machine I, Aixplorer system, SuperSonic Imagine; machine II, Acuson S3000, Siemens Healthcare; machine III, Resona 7, Mindray. SWS, shear wave speed; ICC, intra-class correlation coefficients; M ± SD, mean ± standard deviation; CV, coefficient of variation; CI, confidence interval.
Table 4
Probes | SWS (M ± SD) (m/s) | P value | CV | Intra-system ICC (95% CI) |
---|---|---|---|---|
Probe A | 2.85±0.22 | 0.053 | 0.08 | 0.84 (0.69–0.93) |
Probe B | 2.98±0.23 | 0.08 | 0.90 (0.81–0.95) |
P<0.05 was considered statistically significant. Probe A, Aixplorer system, SL 10-2; Probe B, Aixplorer system, SL. ICC, intra-class correlation coefficients; SWS, shear wave speed; M ± SD, mean ± standard deviation; CV, coefficient of variation; CI, confidence interval.
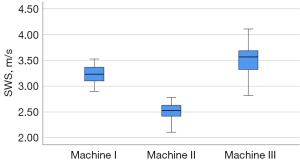
The Kolmogorov-Smirnov test showed all P values =0.20 for the 3 groups of data from the 3 machines. The SWS acquired at a depth of 2 cm was significantly different between the 3 machines (P<0.001). In the subgroup analysis, there were obvious differences between any 2 machines (P<0.001). The CVs were 0.06 in machines I and II, while the CV was 0.08 in machine III. The highest CV and SWS were associated with machine III, and the lowest CV and SWS were associated with machine II. The SWS acquired from all 3 machines produced a substantial intra-system reproducibility (ICC, 0.61–0.80). The SWS acquired from machine II achieved the best intra-system reliability compared to the results acquired from machines I and III. Comparing the results from the 3 machines, the degree of inter-system agreement reproducibility was poor (ICC, <0.20).
For the probe comparison, the test for homogeneity of variance showed P=0.73, which indicated the variances were equal. No significant difference was found between SWS acquired with the 2 linear probes of different frequencies (P>0.05), and both probes had almost perfectly consistent results (ICC, >0.80).
Results obtained under different angles
The SWS was successfully acquired from 15 muscle samples.
The results of SWS with different angles in machine I are shown in Table 5. The SWS measured at different angles (0–40°) was significantly different (P<0.05). When the angle was less than 20°, the CVs were small. There was no significant difference between the SWS measured at angles 5–20° and 0°. When the angle increased to 25° or more, the CVs became large, and the SWS difference between the large angles (≥25°) and 0° occurred. The largest CV and the lowest ICC were related to an angle of 35°. The filling rate of the elastogram was extremely low, and only 2 specimens were successfully measured at an angle of 40°. The SWS acquired at 0–25° achieved the best intra-system reliability compared to the other angles. The comparison of SWS measured at different angles is shown in Figure 6.
Table 5
Parameters | 0° | 5° | 10° | 15° | 20° | 25° | 30° | 35° | 40° |
---|---|---|---|---|---|---|---|---|---|
SWS (M ± SD) (m/s) | 3.97±0.38 | 3.96±0.61 | 3.96±0.57 | 3.87±0.38 | 3.91±0.53 | 4.67±1.09 | 4.95±1.26 | 3.69±1.48 | 5.32±0.92 |
CV | 0.10 | 0.15 | 0.14 | 0.10 | 0.14 | 0.23 | 0.25 | 0.40 | 0.17 |
ICC | 0.96 (0.89–0.99) | 0.90 (0.77–0.97) | 0.92 (0.80–0.97) | 0.86 (0.69–0.95) | 0.85 (0.67–0.95) | 0.89 (0.76–0.96) | 0.45 (0.09–0.77) | 0.29 (0–0.73) | 0.80 (0.08–0.99) |
P value | 0.008* |
P<0.05 was considered statistically significant. *, denotes a significant difference between groups (P<0.05). Machine I, Aixplorer system, SuperSonic Imagine. SWS, shear wave speed; M ± SD, mean ± standard deviation; CV, coefficient of variation; ICC, intra-class correlation coefficients.
Discussion
The SWE is a valuable and promising tool for disease diagnosis of the musculoskeletal system, and the accurate evaluation of muscle elasticity is of great significance for clinical diagnosis (28). To the best of our knowledge, few studies have explored the same variables using ex vivo muscle samples. There is also limited information on the performance of the Mindray SWE system. The present study focused on the effects of different ROI sizes, various probe orientations, probes of different frequencies, and popular machines from 3 different manufacturers on muscle SWS that may be important for the future standardization of muscle evaluation.
The objective of the first part of our study was to evaluate the influence of various ROI sizes on SWS and the reproducibility of SWS under different ROI sizes. Kot et al. (29) acquired muscle elasticity with 3 different ROI sizes (8, 10, and 12 mm diameter for the right rectus femoris and 2, 3, and 4 mm diameter for the patella tendon) and reported no significant difference in the mean value of the elasticity modulus (29). Alfuraih et al. evaluated the elasticity of the rectus femoris muscle and reported that SWS did not differ substantively by ROI size (15, 75, and 200 mm2), but reliability indices indicated that the smaller ROI did not perform quite as well as the larger sizes (21). Our study demonstrated that the mean value did not differ with various ROI sizes, and the intra-and inter-operator ICC was almost perfect except for the inter-operator ICC for an ROI of 1 mm. When evaluating the elasticity of muscles with a relatively uniform elastic distribution, the SWS values were the average value of the entire circular ROI, which may explain why no significant difference in the mean value of the SWS was found. Therefore, it is acceptable to report the mean SWS value of the muscle when using different ROI sizes. Our study demonstrated that using an ROI of 1–10 mm produced low variability and high reproducibility. In routine examinations, the ROI size might be tailored to the specific circumstances. A larger ROI may be necessary for diseases affecting a large muscle area, and a smaller ROI may be more suitable for small focal lesions (21).
The second part of this study investigated the variations in SWS of ex vivo muscles when using different machines and probes. Shin et al. investigated consistency of SWS in ultrasound elastography using different machines and probes (a high-frequency linear probe and a low-frequency convex probe) and found a considerable difference in SWS (24). However, their research focused on a phantom study, and the results may not apply to muscle tissues. Alfuraih et al. compared the reliability between 2 different machines (LOGIQ E9 and Aixplorer) within muscles (21). They reported that the LOGIQ E9 system produced reliable results that were comparable with the Aixplorer system; however, their study was an in vivo experiment that was susceptible to muscle state and body posture, and they did not evaluate the influence of different frequency probes. Another study compared SWS using the ACUSON S2000 and ElastPQ machines and revealed significant differences in liver stiffness between the 2 machines (30). However, to our knowledge, only a few studies have compared SWS obtained from 3 different machines of different manufacturers and tested the systems’ reliability and consistency on ex vivo musculoskeletal imaging. The present study showed a considerable difference in SWS on ultrasound elastography obtained from the machines supplied by different manufacturers. This is likely because machines from different manufacturers are somewhat different in the way they generate and calculate SWS in the tissues. Also, this study briefly compared the degree of agreement between 3 repeated acquisitions using the 3 machines. The results showed that the intra-system consistency of each machine was satisfactory, whereas the inter-system consistency of the 3 machines was unsatisfactory. Therefore, care should be taken in clinical practice or scientific studies when comparing results from different elastography machines for patient follow-up. The CVs of the present study were small, ranging from 0.06 to 0.08, and similar to the results of a previous phantom study, which showed CVs of 0–0.09 at depths of 2–5 cm (24).
Several studies have shown that probes with different frequencies can affect SWS measurement results. Chang et al. acquired the SWS of an elasticity phantom and normal liver with convex and linear probes and showed that the SWS with a low-frequency probe was higher at the same depth using the ACUSON S2000 (31). Another study investigated the normal liver stiffness values of healthy children using acoustic radiation force impulse (ARFI) technology with the ACUSON S2000 equipped with convex and linear probes and found that the SWS obtained using a linear probe was lower than that obtained using a convex probe (32). These studies suggested that these differences might be caused by different frequencies and spatial resolutions. Dillman et al. used linear probes of 2–10 and 4–15 MHz to evaluate superficial soft phantoms at 1.0, 2.5, and 4.0 cm depths using the Aixplorer system and reported a non-significant effect of the probes on SWS measurements (23). The present study compared 2 linear probes with different frequencies (SL 10-2 and SL 15-4) on ex vivo muscles at a 2 cm depth and found no significantly different effects on muscle SWS. This result is probably due to the similar working performance of the 2 probes, and the frequency of the push pulse may have been identical given that the bandwidth of both probes overlaps significantly (2–10 and 4–15 MHz). Also, the acquisition depth was not deep enough to make the sound attenuation obvious.
The last part of this study evaluated the effect of the angle between the probe plane and the muscle surface on SWS. Our results suggest that the acceptable detection angle range is between 0–20° when scanning muscles. Alfuraih et al. tested the elasticity of the rectus femoris in vivo in the longitudinal (along the muscle fiber direction), oblique, and lateral directions, revealing that the longitudinal direction was the best probe orientation (21). Miyamoto et al. studied the effect of probe angle on shear wave modulus by placing the probe parallel or 20° obliquely to the fascicle using B-mode images and found that the reproducibility of the shear modulus measurements was high in both the parallel and oblique conditions (25). However, they only assessed 2 angles, and our study applied a larger angle range and explored an acceptable range of angles. Our study found that when the angle was ≤20°, there was no obvious difference in the SWS compared with 0°, along with high reliability and small CVs. However, when the angle was >20°, there was a significant difference in SWS compared with 0°, with larger CVs and suddenly increased or decreased SWS. The heterogeneous high or low tissue elastogram observed in the larger acquisition angles (>20°) may be due to the poor propagation of shear waves in a more anisotropic structure. Larger angles produced a higher level of anisotropy than smaller angles. Therefore, when defining the probe orientation in anisotropic structures, the orientation of the fibers must be considered. An angle of no more than 20° between the muscle fiber and probe will result in better reproducibility and higher reliability, and larger angles (≥25°) will produce inaccurate SWS measurements.
Our study had some limitations. Firstly, isolated porcine muscles were used and successively measured by different operators; therefore, the tissue may have decomposed over time. Secondly, the temperature and perfusion of the isolated muscles were different from the living body temperature, which may have led to elasticity changes in the tissues. Thirdly, for SWS comparison of different angles, the thickness of the couplant applied to the muscle surface was different; larger angles needed more couplant on the muscle surface. This may have caused differences in sound attenuation, further affecting the SWS measurement. Lastly, for better evaluation of the intra-observer agreement, a longer time interval was needed. However, in the present study, 3 measurements were carried out with a time interval between the 3 measurements of only 8–10 min to avoid elasticity changes over time.
Conclusions
Machines from different manufacturers have a significant effect on muscle SWS measurements. Attention should be paid during patient follow-ups when comparing results from different machines. There was no difference in muscle SWS between the 2 linear probes with the Aixplorer system. Under the present study conditions, the size of the ROI had no significant effect on muscle SWS measurements. Therefore, the size of the ROI could be adjusted according to different situations. When evaluating muscle elasticity, the recommended acquisition angle should be no more than 20°, as an angle greater than 25° will lead to inaccurate SWS measurements.
Acknowledgments
Funding: This work was supported by the National Natural Science Foundation of China (No. 82071930).
Footnote
Reporting Checklist: The authors have completed the GRRAS reporting checklist. Available at https://qims.amegroups.com/article/view/10.21037/qims-21-1072/rc
Conflicts of Interest: All authors have completed the ICMJE uniform disclosure form (available at https://qims.amegroups.com/article/view/10.21037/qims-21-1072/coif). The authors have no conflicts of interest to declare.
Ethical Statement: The authors are accountable for all aspects of the work in ensuring that questions related to the accuracy or integrity of any part of the work are appropriately investigated and resolved.
Open Access Statement: This is an Open Access article distributed in accordance with the Creative Commons Attribution-NonCommercial-NoDerivs 4.0 International License (CC BY-NC-ND 4.0), which permits the non-commercial replication and distribution of the article with the strict proviso that no changes or edits are made and the original work is properly cited (including links to both the formal publication through the relevant DOI and the license). See: https://creativecommons.org/licenses/by-nc-nd/4.0/.
References
- Yin L, Cheng L, Wang F, Zhu X, Hua Y, He W. Application of intraoperative B-mode ultrasound and shear wave elastography for glioma grading. Quant Imaging Med Surg 2021;11:2733-43. [Crossref] [PubMed]
- Winn N, Lalam R, Cassar-Pullicino V. Sonoelastography in the musculoskeletal system: Current role and future directions. World J Radiol 2016;8:868-79. [Crossref] [PubMed]
- Barr RG, Nakashima K, Amy D, Cosgrove D, Farrokh A, Schafer F, et al. WFUMB guidelines and recommendations for clinical use of ultrasound elastography: Part 2: breast. Ultrasound Med Biol 2015;41:1148-60. [Crossref] [PubMed]
- Ferraioli G, Filice C, Castera L, Choi BI, Sporea I, Wilson SR, et al. WFUMB guidelines and recommendations for clinical use of ultrasound elastography: Part 3: liver. Ultrasound Med Biol 2015;41:1161-79. [Crossref] [PubMed]
- Cosgrove D, Barr R, Bojunga J, Cantisani V, Chammas MC, Dighe M, Vinayak S, Xu JM, Dietrich CF. WFUMB Guidelines and Recommendations on the Clinical Use of Ultrasound Elastography: Part 4. Thyroid. Ultrasound Med Biol 2017;43:4-26. [Crossref] [PubMed]
- Barr RG, Cosgrove D, Brock M, Cantisani V, Correas JM, Postema AW, Salomon G, Tsutsumi M, Xu HX, Dietrich CF. WFUMB Guidelines and Recommendations on the Clinical Use of Ultrasound Elastography: Part 5. Prostate. Ultrasound Med Biol 2017;43:27-48. [Crossref] [PubMed]
- Wang X, Hu Y, Zhu J, Gao J, Chen S, Liu F, Li W, Liu Y, Ariun B. Effect of acquisition depth and precompression from probe and couplant on shear wave elastography in soft tissue: an in vitro and in vivo study. Quant Imaging Med Surg 2020;10:754-65. [Crossref] [PubMed]
- Tang X, Wang L, Guo R, Huang S, Tang Y, Qiu L. Application of ultrasound elastography in the evaluation of muscle strength in a healthy population. Quant Imaging Med Surg 2020;10:1961-72. [Crossref] [PubMed]
- Cornu C, Goubel F, Fardeau M. Muscle and joint elastic properties during elbow flexion in Duchenne muscular dystrophy. J Physiol 2001;533:605-16. [Crossref] [PubMed]
- Fridén J, Lieber RL. Spastic muscle cells are shorter and stiffer than normal cells. Muscle Nerve 2003;27:157-64. [Crossref] [PubMed]
- Song Y, Lee S, Yoo DH, Jang KS, Bae J. Strain sonoelastography of inflammatory myopathies: comparison with clinical examination, magnetic resonance imaging and pathologic findings. Br J Radiol 2016;89:20160283. [Crossref] [PubMed]
- Gennisson JL, Deffieux T, Fink M, Tanter M. Ultrasound elastography: principles and techniques. Diagn Interv Imaging 2013;94:487-95. [Crossref] [PubMed]
- Jeong WK, Lim HK, Lee HK, Jo JM, Kim Y. Principles and clinical application of ultrasound elastography for diffuse liver disease. Ultrasonography 2014;33:149-60. [Crossref] [PubMed]
- Ophir J, Céspedes I, Ponnekanti H, Yazdi Y, Li X. Elastography: a quantitative method for imaging the elasticity of biological tissues. Ultrason Imaging 1991;13:111-34. [Crossref] [PubMed]
- Ruby L, Mutschler T, Martini K, Klingmüller V, Frauenfelder T, Rominger MB, Sanabria SJ. Which Confounders Have the Largest Impact in Shear Wave Elastography of Muscle and How Can They be Minimized? An Elasticity Phantom, Ex Vivo Porcine Muscle and Volunteer Study Using a Commercially Available System. Ultrasound Med Biol 2019;45:2591-611. [Crossref] [PubMed]
- Romano A, Staber D, Grimm A, Kronlage C, Marquetand J. Limitations of Muscle Ultrasound Shear Wave Elastography for Clinical Routine-Positioning and Muscle Selection. Sensors (Basel) 2021;21:8490. [Crossref] [PubMed]
- Taljanovic MS, Gimber LH, Becker GW, Latt LD, Klauser AS, Melville DM, Gao L, Witte RS. Shear-Wave Elastography: Basic Physics and Musculoskeletal Applications. Radiographics 2017;37:855-70. [Crossref] [PubMed]
- Dubois G, Kheireddine W, Vergari C, Bonneau D, Thoreux P, Rouch P, Tanter M, Gennisson JL, Skalli W. Reliable protocol for shear wave elastography of lower limb muscles at rest and during passive stretching. Ultrasound Med Biol 2015;41:2284-91. [Crossref] [PubMed]
- Cortez CD, Hermitte L, Ramain A, Mesmann C, Lefort T, Pialat JB. Ultrasound shear wave velocity in skeletal muscle: A reproducibility study. Diagn Interv Imaging 2016;97:71-9. [Crossref] [PubMed]
- Rominger MB, Kälin P, Mastalerz M, Martini K, Klingmüller V, Sanabria S, Frauenfelder T. Influencing Factors of 2D Shear Wave Elastography of the Muscle - An Ex Vivo Animal Study. Ultrasound Int Open 2018;4:E54-60. [Crossref] [PubMed]
- Alfuraih AM, O'Connor P, Tan AL, Hensor E, Emery P, Wakefield RJ. An investigation into the variability between different shear wave elastography systems in muscle. Med Ultrason 2017;19:392-400. [Crossref] [PubMed]
- Hall TJ, Milkowski A, Garra B, Carson P, Palmeri M, Nightingale K, et al. RSNA/QIBA: Shear wave speed as a biomarker for liver fibrosis staging. In 2013 IEEE International Ultrasonics Symposium (IUS). IEEE, 2013:397-400.
- Dillman JR, Chen S, Davenport MS, Zhao H, Urban MW, Song P, Watcharotone K, Carson PL. Superficial ultrasound shear wave speed measurements in soft and hard elasticity phantoms: repeatability and reproducibility using two ultrasound systems. Pediatr Radiol 2015;45:376-85. [Crossref] [PubMed]
- Shin HJ, Kim MJ, Kim HY, Roh YH, Lee MJ. Comparison of shear wave velocities on ultrasound elastography between different machines, transducers, and acquisition depths: a phantom study. Eur Radiol 2016;26:3361-7. [Crossref] [PubMed]
- Miyamoto N, Hirata K, Kanehisa H, Yoshitake Y. Validity of measurement of shear modulus by ultrasound shear wave elastography in human pennate muscle. PLoS One 2015;10:e0124311. [Crossref] [PubMed]
- Creze M, Nordez A, Soubeyrand M, Rocher L, Maître X, Bellin MF. Shear wave sonoelastography of skeletal muscle: basic principles, biomechanical concepts, clinical applications, and future perspectives. Skeletal Radiol 2018;47:457-71. [Crossref] [PubMed]
- Yamanaka N, Kaminuma C, Taketomi-Takahashi A, Tsushima Y. Reliable measurement by virtual touch tissue quantification with acoustic radiation force impulse imaging: phantom study. J Ultrasound Med 2012;31:1239-44. [Crossref] [PubMed]
- Ryu J, Jeong WK. Current status of musculoskeletal application of shear wave elastography. Ultrasonography 2017;36:185-97. [Crossref] [PubMed]
- Kot BC, Zhang ZJ, Lee AW, Leung VY, Fu SN. Elastic modulus of muscle and tendon with shear wave ultrasound elastography: variations with different technical settings. PLoS One 2012;7:e44348. [Crossref] [PubMed]
- Sporea I, Bota S, Grădinaru-Taşcău O, Şirli R, Popescu A. Comparative study between two point Shear Wave Elastographic techniques: Acoustic Radiation Force Impulse (ARFI) elastography and ElastPQ. Med Ultrason 2014;16:309-14. [PubMed]
- Chang S, Kim MJ, Kim J, Lee MJ. Variability of shear wave velocity using different frequencies in acoustic radiation force impulse (ARFI) elastography: a phantom and normal liver study. Ultraschall Med 2013;34:260-5. [PubMed]
- Fontanilla T, Cañas T, Macia A, Alfageme M, Gutierrez Junquera C, Malalana A, Luz Cilleruelo M, Roman E, Miralles M. Normal values of liver shear wave velocity in healthy children assessed by acoustic radiation force impulse imaging using a convex probe and a linear probe. Ultrasound Med Biol 2014;40:470-7. [Crossref] [PubMed]