Rapid quantification of global brain volumetry and relaxometry in patients with multiple sclerosis using synthetic magnetic resonance imaging
Introduction
Multiple sclerosis (MS) is a neurological disease pathologically characterized by demyelination, inflammation, axonal loss, and gliosis scattered throughout the central nervous system (CNS). Clinically, MS is considered primarily a white matter (WM) disease. WM lesion load, as measured by magnetic resonance imaging (MRI), has been used to explain part of the pathological processes (1-3). However, specific cognitive impairments, such as reduced mental processing, attention impairment, and memory deficits, which can be found in 45–65% of MS patients (4-6), might be better explained by physical disability in gray matter (GM) (7,8). Some pathological studies have reported significant foci of GM lesions in the cerebral cortex and other GM regions are of importance (9-11).
Conventional MRI plays an important role in the diagnosis and follow-up of MS patients (12). Quantitative measurements, such as the loss of specific tissue types and global and regional brain atrophy, are gaining increasing attention as critical clinical markers to determine disease severity and prognosis in MS patients (13). Compared with conventional MR imaging techniques, quantitative MR imaging can not only characterize the focal visualized lesions of MS but also detect the hidden abnormalities in normal-appearing gray matter (NAGM) and normal-appearing white matter (NAWM) (14-16).
In the vast majority, only one parameter per scan can be measured using quantitative MR. Synthetic MRI allows simultaneous acquisition of T1, T2 and PD values, and subsequent reconstruction of synthetic images that resemble conventional MR images from a single scan within a clinically time. Importantly, synthetic MRI can automatically segment brain tissue based on the relaxation value and provide volumetry information of different types of brain tissues, including volumes of GM, WM, myelin content, and cerebral spinal fluid (CSF) (17,18). Furthermore, the method offers scanner independence and acceptable scanning times, since a single acquisition can provide data for reconstructing all image sets.
To the best of our knowledge, there are no previous reports of using synthetic MRI to simultaneously explore global and regional GM volumetry and relaxometry information in MS by automatically segmenting brain regions. The purpose of this study was to quantitatively assess the changes of GM volumetry and relaxometry information simultaneously in MS patients using synthetic MRI. We present the following article in accordance with the STROBE reporting checklist (available at https://qims.amegroups.com/article/view/10.21037/qims-21-970/rc)
Methods
Subjects
This study was conducted in accordance with the Declaration of Helsinki (as revised in 2013). The study was approved by the Medical Research Ethics Committee of China Medical University, and informed consent was provided by all participants.
All MS patients and healthy controls (HCs) were recruited at the First Hospital of China Medical University from September 2019 to May 2021. The inclusion criteria for this study were as follows: age between 18 and 65 years; had a clinically definite MS based on revised McDonald’s criteria (19); study exclusion criteria were as follows: (I) had received corticosteroid treatment in the last 2 weeks preceding the study (n=3); (II) had the previous history of other CNS’s diseases such as demyelinating and neurodegenerative diseases, brain tumor or surgery, head injury, cerebrovascular disease (n=1); (III) had poor image quality (n=3). The age-well matched HC subjects had no history of hypertension, neurological or psychiatric diseases, brain malformations, or trauma based on the conventional MRI. The flowchart of this study was shown in Figure 1. Neurological disability was assessed using the Expanded Disability Status Scale (EDSS) within one week after the MRI scan (20).
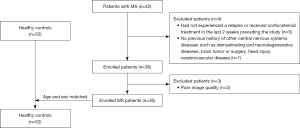
MRI protocol
MRI images were acquired based on a 3.0 Tesla MR scanner (SIGNA Pioneer, GE Healthcare, Milwaukee, WI, USA) equipped with a standard 21-channel phased-array head-neck coil. Conventional axial T1 and T2-weighted images (T1WI and T2WI), sagittal T1WI, and fluid-attenuated inversion recovery (FLAIR) were obtained to exclude participants with brain lesions.
Synthetic MRI [MAGnetic resonance image Compilation (MAGiC)] images (21) were obtained through the whole head for each subject using multiple-delay-multiple-echo (MDME) sequence: field of view (FOV) =240 mm × 192 mm, in-plane resolution =0.8 mm × 1 mm, matrix =320×192, echo time (TE) 1/TE 2 =17.5 ms/87.7 ms, repetition time (TR) =5,669 ms, echo-train length (ETL) =16, number of excitation (NEX) =2, bandwidth =31.25 kHz, phase acceleration factor =2.5, slice thickness/gap =3/0.6 mm, 38 slices for whole brain coverage, total scan time is 7 min 30 s.
High-resolution images were acquired with a three-dimensional brain volume (3D-BRAVO) sequence: matrix =240×240, FOV =240 mm × 240 mm, TR =6.0 ms, TE =2.9 ms, prep time =400, flip angle =12°, bandwidth =35.71 kHz, NEX =2, phase acceleration factor =2, slice thickness/gap =1/0 mm, slice number =176. The scan time for 3D-BRAVO was 3 min 34 s.
Image processing and analysis
Relaxation maps generated from the MAGiC images were selected for quantitative analysis using SyMRI 8.0 software (SyntheticMR AB, Linköping, Sweden). The total volume includes intracranial volume (ICV), brain parenchymal volume (BPV), myelin volume (MYV), cerebral spinal fluid volume (CSFV), white matter volume (WMV), gray matter volume (GMV), myelin fraction (MYF = MYV/BPV), and brain parenchymal fraction (BPF = BPV/ICV) were also acquired by automatic brain segmentation. The T1, T2, and PD maps provide an absolute scale and hence a robust input to brain segmentation for WM, GM, and CSF. The ICV corresponds to the sum of BPV and CSF. BPV was calculated as the sum of WM, GM, and non-WM/GM/CSF. MYV contains the myelin water and myelin sheaths. The short-time component of the observed T2 relaxation represents the presence of water trapped between the myelin sheaths, termed myelin water. MYV can be automatically calculated in the latest version of the SyMRI software.
Global volumetry and relaxometry data were processed using the following steps: Firstly, rigid registration between the 3D-BRAVO images and the relaxation maps was conducted using SPM12 (www.fil.ion.ucl.ac.uk/spm/software/spm12). Secondly, the individual images of tissue probability maps and normalized relaxation maps were obtained from native space to standard Montreal Neurological Institute (MNI) templates (http://www.mni.mcgill.ca/) via segmentation and normalization using the CAT12 toolbox (http://www.neuro.unijena.de/cat/). The global relaxometry in GM was calculated by averaging the relaxation values from voxels with GM partial volume exceeding 95%. Finally, the brain regional GM volumetry and relaxometry in 33 selected regions were extracted according to the anatomical automatic labeling (AAL) template (22). The post-processing pipeline as shown in Figure 2.
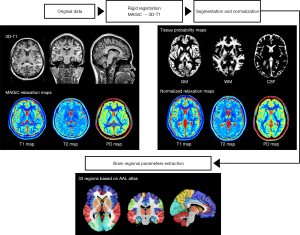
Statistical analysis
All statistical analyses were performed using MATLAB R2020a and SPSS version 22.0 software (SPSS, Chicago, IL, USA). Normal distribution assumption was checked utilizing Kolmogorov-Smirnov and Shapiro-Wilk tests. Student t-tests were used to compare demographic and clinical data (age, education, and ICV) conforming to normal distribution. Chi-square tests were used to examine gender between two groups.
The global brain volumetry, regional brain T1, T2, and PD values between MS patients and HCs were compared using analysis of covariance (ANCOVA). Correlational analyses were performed to determine the correlations of quantitative parameters and clinical variables (EDSS scores, illness duration) using MS patients. The ICV, gender, education, and age were considered as covariates for the following ANCOVA and correlational analyses. Multiple comparisons of all brain regional analyses were controlled by Bonferroni correction according to 33 selected brain regions. A corrected P<0.05 was considered statistically significant. The significant brain subregions were shown by MRIcron software.
Results
Initially, 42 patients were recruited, with 7 subsequently excluded. In the final study cohort, 35 MS patients and 52 age-matched HCs were included. No significant differences in age (P=0.0864) and gender (P=0.5018) were found between the MS patients compared with HCs groups. The illness duration of MS patients ranged from 0.5 to 250 months, with a median duration of 48 months. The mean EDSS score was 3.014±1.358 (see Table 1).
Table 1
Variables | MS (n=35) | HC (n=52) | χ2/t | P |
---|---|---|---|---|
Gender (male/female) | 11/24 | 20/32 | χ2=0.4517 | 0.5018 |
Age (years), mean ± SD | 32.94±10.073 | 29.31±9.243 | t=1.7349 | 0.0864 |
Illness duration (months), median (IQR; range) | 36 (12–68; 0.5–240) | NA | ||
Education (years) | 13.66±2.300 | 14.29±2.269 | t=1.265 | 0.209 |
EDSS score, mean ± SD | 3.014±1.358 | NA | ||
ICV | 1,378.143±119.443 | 1,453.731±121.389 | t=2.866 | 0.0052 |
MS, multiple sclerosis; HC, healthy control; IQR, interquartile range; EDSS, Expanded Disability Status Scale; ICV, intracranial volume.
Quantification of global volumetry
Results of global volumetry differences between the MS patients and HCs are shown in Table 2. The global volumetry including WMV, MYV, and BPV were all significantly lower than MS patients, as well as a higher CSFV (P<0.001). Similarly, BPF and MYF were significantly lower in MS patients (all P<0.01).
Table 2
Variables | HC | MS | t | P |
---|---|---|---|---|
Global volumetry | ||||
GMV (mL) | 626.175±89.486 | 613.120±65.388 | 1.7459 | 0.1904 |
WMV (mL) | 579.903±68.432 | 472.249±58.505 | 43.9486 | <0.001*** |
CSFV (mL) | 177.017±39.729 | 241.294±81.805 | −38.7301 | <0.001*** |
MYV (mL) | 192.457±27.381 | 151.883±22.766 | 38.1444 | <0.001*** |
BPV (mL) | 1,276.712±107.368 | 1,136.771±106.126 | 38.8223 | <0.001*** |
MYF (MYV/BPV) | 13.231±1.465 | 11.034±1.529 | 6.7395 | <0.001*** |
BPF (BPV/ICV) | 87.85±2.392 | 82.623±5.368 | 5.4115 | <0.001*** |
Global GM relaxometry | ||||
T1 | 1321.565±73.652 | 1420.485±49.207 | −43.7581 | <0.001*** |
T2 | 103.014±4.474 | 113.877±10.819 | −39.6669 | <0.001*** |
PD | 67.55±2.507 | 83.082±0.878 | −10.9375 | 0.0013** |
**, P<0.01; ***, P<0.001. MS, multiple sclerosis; HC, healthy control; GMV, gray matter volume; WMV, white matter volume; CSFV, cerebral spinal fluid volume; MYV, myelin volume; BPV, brain parenchymal volume; MYF, myelin fraction; BPF, brain parenchymal fraction; ICV, intracranial volume; GM, gray matter; PD, proton density.
There was no statistical difference in global GMV between the two groups (P>0.05). For regional GM volume measurements, several regions in the MS patients were significantly demonstrated smaller than those of HCs (P<0.05, corrected)(see Table S1, Figure 3).

Quantification of global relaxometry
For global GM relaxometry, the T1, T2, and PD values were higher in MS patients than those in HCs, respectively (P<0.05) (see Table 2).
For regional GM relaxometry, the T1, T2, and PD values of several regions showed significant differences between MS patients and HCs (P<0.05, corrected) (see Table S2, Figure 4, Figure S1A-S1C).
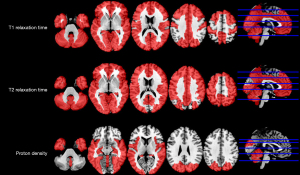
Correlations between quantitative parameters and clinical variables
The global GMV and BPV have shown in Table 3 decreased as the EDSS scores increased (P<0.05). Furthermore, a significant negative correlation was found between regional GM volumetry (olfactory and rectus) and EDSS scores (P<0.05, corrected). But there were no significant correlations between illness duration and quantitative parameters (P>0.05, corrected).
Table 3
Variables | EDSS | |
---|---|---|
r | Significance | |
Global volumetry | ||
GMV | −0.353 | 0.038 |
BPV | −0.357 | 0.035 |
Regional GM volumetry | ||
Olfactory | −0.383 | 0.023 |
Rectus | −0.356 | 0.036 |
EDSS, Expanded Disability Status Scale; GMV, gray matter volume; BPV, brain parenchymal volume; GM, gray matter.
Discussion
Volumetric characteristics
Brain atrophy is considered an important prognostic factor in the assessment of subsequent disability (23-25). Our findings indicated that brain atrophy in MS patients may involve the entire brain, including myelin and WM, suggesting that the pathophysiology of MS patients may be related to the whole brain not only limited to some specific brain regions. The potential neuropathology leads to brain atrophy, and synthetic MRI can monitor these volumetric variations.
The pathology of GM lesions differs from that of WM lesions (26). Some extent axonal transaction can be found in cortical GM lesions in addition to the glial, synaptic, and neuronal deficits (26), which may be necessary for illustrating the consistent findings of cortical thinning and GM atrophy in MS measured by MRI (27).
Different MRI-based techniques (automatic, semi-automatic, and manual) have been applied to measure global and regional brain atrophy. In the current, we have investigated the utility of synthetic MR imaging to determine the atrophic brain regions in MS. Reduced global brain volumetry and increased CSF volume indicated brain atrophy. Significantly lower BPF in patients with MS has been demonstrated by synthetic MRI volumetry. Meanwhile, a reduction of MYV was still found in MS patients, indicating that myelin content decreased. The results are consistent with previous findings (using other methodologies) (24,25,28).
Though we did not find significant global GM atrophy in our present research, which is inconsistent with previous studies (29), multiple significant GM atrophic regions were still detected in MS patients. GM atrophy, which contributes to the multiple atrophic brain regions in patients suffering from MS, may reflect a combination of neurite transaction (30), demyelination, and reduced synapse or glial densities (31,32). Our findings confirm that GM atrophy is not uniform across the brain in MS, and some regions are more vulnerable to atrophy than others (33). These findings are consistent with other histopathological or MR studies, which showed atrophy and demyelinated lesions in GM structures such as the amygdala, pallidum, caudate, thalamus, hypothalamus, hippocampus, and putamen (14,26,34,35). Of course, another possible reason may be the insufficient samples. A larger-sample study should be performed to further investigate the global GM atrophy in the MS.
Relaxometry characteristics
Normal brain tissues have a relatively narrow range of T1, T2, and PD values, while pathological tissues exhibit distinctly deviated values (36). Neuronal and axonal loss or death, water content changes, and demyelination can cause changes in relaxation times even if the tissues appear normal on imaging (37,38). This may speculate about the underlying pathophysiology of MS and have clinical implications in predicting disease progression in MS (i.e., possible resultant neuronal damage and demyelination). Relaxometry is sensitive to the microstructure and composition of the brain and can potentially reveal the alterations of specific brain tissue (13).
We found significantly high T1 values in the selected GM regions in MS patients. These results are similar to the previous studies that showed a prolonged T1 (39,40). Although changes in T1 relaxation time may be related to myelin loss, iron load, amyloid burden, and water content in MS patients, inconsistent results of T1 alterations in GM have been reported (41,42). Previous studies have revealed that hyperintensity of T1-weighted in lesions attributed to T1 shortening, possibly due to remyelination or macrophage activity (13). Thus, the influence of MS on T1 values may be complex (13).
Previous studies have shown that T2 relaxation time was associated with amyloid deposits, myelin density, iron load, and tissue water content (43,44). Our results showed significantly higher T2 values in MS compared to HCs, which is consistent with previous studies. The prolongation of T2 may be due to gliosis, axonal and myelin loss, and increased water content. However, it is difficult to identify which factor plays a dominant role in the changes to T2 value.
PD values can reflect the tissue water content and thus infer the structural damage to the brain. However, there were relatively few studies of PD values in patients with MS. In our work, fewer GM brain regions had PD higher value in MS patients than in normal controls. The reason for this may be because PD is generally less sensitive to pathophysiology than T1 and T2. Future studies are still needed.
Interestingly, there were no significant microstructure alterations common to the MS patients in the deep GM nuclei (caudate, putamen, pallidum), while the atrophy of deep GM nuclei existed. The pathological process of patients with MS is complex. We can speculate that atrophy in the cortical GM in patients with MS is relatively more sensitive and extensive.
Correlations between quantitative parameters and clinical variables
Moreover, there was a strong correlation between global volumetry (GMV and BPV) and EDSS scores. Previous studies have demonstrated the strongest correlations in the advanced stages of MS, and cortical atrophy occurs even before clinical symptoms become apparent (45). Another report has also confirmed that a higher EDSS score was correlated with a higher degree of atrophy (25,39).
We found a statistically significant negative correlation in two out of 33 selected GM regions in MS patients. There were no correlations in other regions. None of the measured GM volumetric or relaxometric metrics showed any association with the illness duration. There are two possible reasons: first, the pathophysiological alterations in brain GM may be global during pathology rather than restricted to a single region. Second, the relatively small sample size may have limited the significance of altered regional GM relaxometry, especially when the number of brain regions to be analyzed was relatively large.
The present study still has some limitations. (I) This study was limited to exploring MS disease and normal controls and did not provide an in-depth comparative analysis of subtypes. Future studies should focus on the characteristics of subtypes of MS. (II) We did not analyze the focal MS plaques characteristics in the present study. The plaque characteristics should also be further explored in the future. (III) We did not evaluate the cognitive function in MS patients. More studies will be explored the association between cognitive function and image characteristics.
Conclusions
These findings suggest thatMS patients had global and regional brain volumetry and relaxometry alterations, and the synthetic MRI-derived parameters may be potentially used as specific quantitative markers for the clinic to improve the understanding of MS.
Acknowledgments
The authors thank Dr. Yongnan Mu (Department of Medicine, Englewood Hospital, Englewood, NJ, USA) for polishing the language, and Lizhi Xie from GE healthcare for expert technical assistance with MRI.
Funding: None.
Footnote
Reporting Checklist: The authors have completed the STROBE reporting checklist. Available at https://qims.amegroups.com/article/view/10.21037/qims-21-970/rc
Conflicts of Interest: All authors have completed the ICMJE uniform disclosure form (available at https://qims.amegroups.com/article/view/10.21037/qims-21-970/coif). PW reports that he is the employee of GE Healthcare, which developed the data post-processing method used in this paper.The otherauthors have no conflicts of interest to declare.
Ethical Statement:
Open Access Statement: This is an Open Access article distributed in accordance with the Creative Commons Attribution-NonCommercial-NoDerivs 4.0 International License (CC BY-NC-ND 4.0), which permits the non-commercial replication and distribution of the article with the strict proviso that no changes or edits are made and the original work is properly cited (including links to both the formal publication through the relevant DOI and the license). See: https://creativecommons.org/licenses/by-nc-nd/4.0/.
References
- Rovaris M, Filippi M, Minicucci L, Iannucci G, Santuccio G, Possa F, Comi G. Cortical/subcortical disease burden and cognitive impairment in patients with multiple sclerosis. AJNR Am J Neuroradiol 2000;21:402-8. [PubMed]
- Feinstein A, Roy P, Lobaugh N, Feinstein K, O'Connor P, Black S. Structural brain abnormalities in multiple sclerosis patients with major depression. Neurology 2004;62:586-90. [Crossref] [PubMed]
- Moriarty DM, Blackshaw AJ, Talbot PR, Griffiths HL, Snowden JS, Hillier VF, Capener S, Laitt RD, Jackson A. Memory dysfunction in multiple sclerosis corresponds to juxtacortical lesion load on fast fluid-attenuated inversion-recovery MR images. AJNR Am J Neuroradiol 1999;20:1956-62. [PubMed]
- Calabrese P. Neuropsychology of multiple sclerosis--an overview. J Neurol 2006;253:I10-5. [Crossref] [PubMed]
- Benedict RHB, DeLuca J, Enzinger C, Geurts JJG, Krupp LB, Rao SM. Neuropsychology of Multiple Sclerosis: Looking Back and Moving Forward. J Int Neuropsychol Soc 2017;23:832-42. [Crossref] [PubMed]
- Rao SM. Neuropsychology of multiple sclerosis. Curr Opin Neurol 1995;8:216-20. [Crossref] [PubMed]
- Amato MP, Portaccio E, Goretti B, Zipoli V, Battaglini M, Bartolozzi ML, Stromillo ML, Guidi L, Siracusa G, Sorbi S, Federico A, De Stefano N. Association of neocortical volume changes with cognitive deterioration in relapsing-remitting multiple sclerosis. Arch Neurol 2007;64:1157-61. [Crossref] [PubMed]
- Barkhof F. The clinico-radiological paradox in multiple sclerosis revisited. Curr Opin Neurol 2002;15:239-45. [Crossref] [PubMed]
- Hagiwara A, Kamagata K, Shimoji K, Yokoyama K, Andica C, Hori M, Fujita S, Maekawa T, Irie R, Akashi T, Wada A, Suzuki M, Abe O, Hattori N, Aoki S. White Matter Abnormalities in Multiple Sclerosis Evaluated by Quantitative Synthetic MRI, Diffusion Tensor Imaging, and Neurite Orientation Dispersion and Density Imaging. AJNR Am J Neuroradiol 2019;40:1642-8. [Crossref] [PubMed]
- Honce JM. Gray Matter Pathology in MS: Neuroimaging and Clinical Correlations. Mult Scler Int 2013;2013:627870. [Crossref] [PubMed]
- Andica C, Hagiwara A, Kamagata K, Yokoyama K, Shimoji K, Saito A, Takenaka Y, Nakazawa M, Hori M, Cohen-Adad J, Takemura MY, Hattori N, Aoki S. Gray Matter Alterations in Early and Late Relapsing-Remitting Multiple Sclerosis Evaluated with Synthetic Quantitative Magnetic Resonance Imaging. Sci Rep 2019;9:8147. [Crossref] [PubMed]
- Polman CH, Reingold SC, Banwell B, Clanet M, Cohen JA, Filippi M, Fujihara K, Havrdova E, Hutchinson M, Kappos L, Lublin FD, Montalban X, O'Connor P, Sandberg-Wollheim M, Thompson AJ, Waubant E, Weinshenker B, Wolinsky JS. Diagnostic criteria for multiple sclerosis: 2010 revisions to the McDonald criteria. Ann Neurol 2011;69:292-302. [Crossref] [PubMed]
- Does MD. Inferring brain tissue composition and microstructure via MR relaxometry. Neuroimage 2018;182:136-48. [Crossref] [PubMed]
- Filippi M, Rocca MA, Barkhof F, Brück W, Chen JT, Comi G, DeLuca G, De Stefano N, Erickson BJ, Evangelou N, Fazekas F, Geurts JJ, Lucchinetti C, Miller DH, Pelletier D, Popescu BF, Lassmann H. Attendees of the Correlation between Pathological MRI findings in MS workshop. Association between pathological and MRI findings in multiple sclerosis. Lancet Neurol 2012;11:349-60. [Crossref] [PubMed]
- Davies GR, Altmann DR, Hadjiprocopis A, Rashid W, Chard DT, Griffin CM, Tofts PS, Barker GJ, Kapoor R, Thompson AJ, Miller DH. Increasing normal-appearing grey and white matter magnetisation transfer ratio abnormality in early relapsing-remitting multiple sclerosis. J Neurol 2005;252:1037-44. [Crossref] [PubMed]
- Stevenson VL, Parker GJ, Barker GJ, Birnie K, Tofts PS, Miller DH, Thompson AJ. Variations in T1 and T2 relaxation times of normal appearing white matter and lesions in multiple sclerosis. J Neurol Sci 2000;178:81-7. [Crossref] [PubMed]
- Li C, Chen Y, Wu PY, Wu B, Gong T, Wang H, Chen M. Associations between brain volumetry and relaxometry signatures and the Edmonton Frail Scale in frailty. Quant Imaging Med Surg 2021;11:2560-71. [Crossref] [PubMed]
- Hagiwara A, Warntjes M, Hori M, Andica C, Nakazawa M, Kumamaru KK, Abe O, Aoki S. SyMRI of the Brain: Rapid Quantification of Relaxation Rates and Proton Density, With Synthetic MRI, Automatic Brain Segmentation, and Myelin Measurement. Invest Radiol 2017;52:647-57. [Crossref] [PubMed]
- Thompson AJ, Banwell BL, Barkhof F, Carroll WM, Coetzee T, Comi G, et al. Diagnosis of multiple sclerosis: 2017 revisions of the McDonald criteria. Lancet Neurol 2018;17:162-73. [Crossref] [PubMed]
- Kurtzke JF. Rating neurologic impairment in multiple sclerosis: an expanded disability status scale (EDSS). Neurology 1983;33:1444-52. [Crossref] [PubMed]
- Tanenbaum LN, Tsiouris AJ, Johnson AN, Naidich TP, DeLano MC, Melhem ER, Quarterman P, Parameswaran SX, Shankaranarayanan A, Goyen M, Field AS. Synthetic MRI for Clinical Neuroimaging: Results of the Magnetic Resonance Image Compilation (MAGiC) Prospective, Multicenter, Multireader Trial. AJNR Am J Neuroradiol 2017;38:1103-10. [Crossref] [PubMed]
- Tzourio-Mazoyer N, Landeau B, Papathanassiou D, Crivello F, Etard O, Delcroix N, Mazoyer B, Joliot M. Automated anatomical labeling of activations in SPM using a macroscopic anatomical parcellation of the MNI MRI single-subject brain. Neuroimage 2002;15:273-89. [Crossref] [PubMed]
- Shattuck DW, Sandor-Leahy SR, Schaper KA, Rottenberg DA, Leahy RM. Magnetic resonance image tissue classification using a partial volume model. Neuroimage 2001;13:856-76. [Crossref] [PubMed]
- Vågberg M, Lindqvist T, Ambarki K, Warntjes JB, Sundström P, Birgander R, Svenningsson A. Automated determination of brain parenchymal fraction in multiple sclerosis. AJNR Am J Neuroradiol 2013;34:498-504. [Crossref] [PubMed]
- Kassubek J, Tumani H, Ecker D, Kurt A, Ludolph AC, Juengling FD. Age-related brain parenchymal fraction is significantly decreased in young multiple sclerosis patients: a quantitative MRI study. Neuroreport 2003;14:427-30. [Crossref] [PubMed]
- Geurts JJ, Barkhof F. Grey matter pathology in multiple sclerosis. Lancet Neurol 2008;7:841-51. [Crossref] [PubMed]
- Prinster A, Quarantelli M, Orefice G, Lanzillo R, Brunetti A, Mollica C, Salvatore E, Morra VB, Coppola G, Vacca G, Alfano B, Salvatore M. Grey matter loss in relapsing-remitting multiple sclerosis: a voxel-based morphometry study. Neuroimage 2006;29:859-67. [Crossref] [PubMed]
- Saccenti L, Hagiwara A, Andica C, Yokoyama K, Fujita S, Kato S, Maekawa T, Kamagata K, Le Berre A, Hori M, Wada A, Tateishi U, Hattori N, Aoki S. Myelin Measurement Using Quantitative Magnetic Resonance Imaging: A Correlation Study Comparing Various Imaging Techniques in Patients with Multiple Sclerosis. Cells 2020;9:393. [Crossref] [PubMed]
- Fisher E, Lee JC, Nakamura K, Rudick RA. Gray matter atrophy in multiple sclerosis: a longitudinal study. Ann Neurol 2008;64:255-65. [Crossref] [PubMed]
- Peterson JW, Bö L, Mörk S, Chang A, Trapp BD. Transected neurites, apoptotic neurons, and reduced inflammation in cortical multiple sclerosis lesions. Ann Neurol 2001;50:389-400. [Crossref] [PubMed]
- Wegner C, Esiri MM, Chance SA, Palace J, Matthews PM. Neocortical neuronal, synaptic, and glial loss in multiple sclerosis. Neurology 2006;67:960-7. [Crossref] [PubMed]
- Dutta R, Chang A, Doud MK, Kidd GJ, Ribaudo MV, Young EA, Fox RJ, Staugaitis SM, Trapp BD. Demyelination causes synaptic alterations in hippocampi from multiple sclerosis patients. Ann Neurol 2011;69:445-54. [Crossref] [PubMed]
- Steenwijk MD, Geurts JJ, Daams M, Tijms BM, Wink AM, Balk LJ, Tewarie PK, Uitdehaag BM, Barkhof F, Vrenken H, Pouwels PJ. Cortical atrophy patterns in multiple sclerosis are non-random and clinically relevant. Brain 2016;139:115-26. [Crossref] [PubMed]
- Datta S, Staewen TD, Cofield SS, Cutter GR, Lublin FD, Wolinsky JS, Narayana PA. CombiRx Investigators Group. Regional gray matter atrophy in relapsing remitting multiple sclerosis: baseline analysis of multi-center data. Mult Scler Relat Disord 2015;4:124-36. [Crossref] [PubMed]
- Al-Radaideh A, Athamneh I, Alabadi H, Hbahbih M. Cortical and Subcortical Morphometric and Iron Changes in Relapsing-Remitting Multiple Sclerosis and Their Association with White Matter T2 Lesion Load: A 3-Tesla Magnetic Resonance Imaging Study. Clin Neuroradiol 2019;29:51-64. [Crossref] [PubMed]
- Alfano B, Brunetti A, Covelli EM, Quarantelli M, Panico MR, Ciarmiello A, Salvatore M. Unsupervised, automated segmentation of the normal brain using a multispectral relaxometric magnetic resonance approach. Magn Reson Med 1997;37:84-93. [Crossref] [PubMed]
- Fatouros PP, Marmarou A, Kraft KA, Inao S, Schwarz FP. In vivo brain water determination by T1 measurements: effect of total water content, hydration fraction, and field strength. Magn Reson Med 1991;17:402-13. [Crossref] [PubMed]
- Rooney WD, Johnson G, Li X, Cohen ER, Kim SG, Ugurbil K, Springer CS Jr. Magnetic field and tissue dependencies of human brain longitudinal 1H2O relaxation in vivo. Magn Reson Med 2007;57:308-18. [Crossref] [PubMed]
- Vrenken H, Geurts JJ, Knol DL, van Dijk LN, Dattola V, Jasperse B, van Schijndel RA, Polman CH, Castelijns JA, Barkhof F, Pouwels PJ. Whole-brain T1 mapping in multiple sclerosis: global changes of normal-appearing gray and white matter. Radiology 2006;240:811-20. [Crossref] [PubMed]
- Niepel G, Tench ChR, Morgan PS, Evangelou N, Auer DP, Constantinescu CS. Deep gray matter and fatigue in MS: a T1 relaxation time study. J Neurol 2006;253:896-902. [Crossref] [PubMed]
- Krauss W, Gunnarsson M, Andersson T, Thunberg P. Accuracy and reproducibility of a quantitative magnetic resonance imaging method for concurrent measurements of tissue relaxation times and proton density. Magn Reson Imaging 2015;33:584-91. [Crossref] [PubMed]
- Parry A, Clare S, Jenkinson M, Smith S, Palace J, Matthews PM. MRI brain T1 relaxation time changes in MS patients increase over time in both the white matter and the cortex. J Neuroimaging 2003;13:234-9. [Crossref] [PubMed]
- Gracien RM, Reitz SC, Hof SM, Fleischer V, Zimmermann H, Droby A, Steinmetz H, Zipp F, Deichmann R, Klein JC. Assessment of cortical damage in early multiple sclerosis with quantitative T2 relaxometry. NMR Biomed 2016;29:444-50. [Crossref] [PubMed]
- Schenker C, Meier D, Wichmann W, Boesiger P, Valavanis A. Age distribution and iron dependency of the T2 relaxation time in the globus pallidus and putamen. Neuroradiology 1993;35:119-24. [Crossref] [PubMed]
- Amato MP, Hakiki B, Goretti B, Rossi F, Stromillo ML, Giorgio A, Roscio M, Ghezzi A, Guidi L, Bartolozzi ML, Portaccio E, De Stefano NItalian RIS/MS Study Group. Association of MRI metrics and cognitive impairment in radiologically isolated syndromes. Neurology 2012;78:309-14. [Crossref] [PubMed]