Relationship between carotid artery stiffness and total serum homocysteine in coronary slow flow phenomenon: a high-resolution echo-tracking study
Introduction
Coronary slow flow phenomenon (CSFP), first described by Tambe et al. (1), is defined as the protracted passage of an angiographic contrast agent to the distal portion of the epicardial coronary arteries in the absence of stenosis (2). Although the etiology of slow coronary flow is not well understood, it may be related to coronary microvascular endothelial dysfunction and elevated serum homocysteine (tHcy) levels (3,4). Barutcu et al. (5) investigated the relationship between tHcy concentration and CSFP and found that in patients with CSFP, the serum tHcy level was significantly higher than that in those with normal coronary flow. Elevated plasma tHcy levels have been reported in symptomatic (6,7) and asymptomatic (8) adults with normal coronary arteries but slow blood flow, confirmed by coronary angiography. Studies have shown that elevated tHcy levels are associated with endothelial dysfunction and oxidative stress, both of which contribute to increased risk of cardiovascular disease regardless of conventional risk factors (9,10). Several epidemiological studies have also shown that elevated total homocysteine is strongly related to atherosclerosis (11-13), a leading cause of stroke (14). Arterial stiffness is now acknowledged as an independent risk predictor of future cardiovascular events (15,16). Therefore, the purpose of our study was to evaluate carotid arterial stiffness in patients with CSFP and to explore the relationship between tHcy concentration and carotid artery stiffness. We present the following article in accordance with the Strengthening the Reporting of Observational studies in Epidemiology (STROBE) reporting checklist (available at https://qims.amegroups.com/article/view/10.21037/qims-21-931/rc).
Methods
Study population
The study was conducted in accordance with the Declaration of Helsinki (as revised in 2013). The study was approved by the Institutional Review Board of Beijing Tiantan Hospital. All participants provided written informed consent before their ultrasound examinations. Participants were consecutively selected from patients undergoing coronary angiography at the Department of Cardiology, Beijing Tiantan Hospital, China, between October 2019 and March 2020. A total of 73 patients with slow coronary flow (mean age 59.30±12.50 years) and 73 controls with normal coronary flow (mean age 60.50±10.20 years) were evaluated. Patients with coronary artery stenosis, a myocardial bridge, cardiomyopathy, left ventricular dysfunction, severe arrhythmia, and valvular heart disease were excluded from the study. Clinical characteristics of all enrolled subjects were recorded.
Definition of slow coronary flow
Coronary angiography was performed by the femoral approach using the standard Judkins technique. During coronary angiography, iohexol (Omnipaque; Nycomed Ireland, Cork, Ireland) was manually injected (6–8 mL of contrast agent at each location). The TIMI frame counting method was used to record the coronary flow rate of all cases. According to the method first described by Gibson et al. (17), the TIMI frames for each major coronary artery of each participant in the study were determined. Then, 2 or 3 experienced cardiologists reviewed the angiographic results of all patients. For the TIMI flow grade, the inter-observer and intra-observer agreement (К-value) were 0.73 and 0.78, respectively. Normal coronary arteries were defined as those without significant lumen stenosis or with diameter stenosis of less than 50%.
Carotid artery stiffness assessment
All participants were examined in the supine position in a temperature-controlled environment with the neck turned to the other side of the examination. The research was performed using a duplex Doppler ultrasound instrument (Prosound α-10; Aloka Co., Ltd., Tokyo, Japan) with a 7.5 MHz linear array probe and a high-resolution echo-tracking system. Specifically, the distal common carotid artery was located 2.0 cm inferior to the carotid bulb, and the carotid artery stiffness parameters and the carotid intima-media thickness were obtained in a longitudinal section of plaque-free artery. The systolic blood pressure (Ps) and the diastolic blood pressure (Pd) were measured with a cuff-type manometer, noninvasively. The systolic diameter (Ds) and the diastolic diameter (Dd) were measured and averaged over 3 cardiac cycles. Arterial stiffness parameters were automatically calculated according to the following formulas (18): β index (β) = ln(Ps/Pd)/(Ds – Dd/Dd); arterial compliance (AC) = π (Ds × Ds – Dd × Dd)/4(Ps – Pd); augmentation index (AIx) = [∆P/(Ps – Pd)] × 100; and pressure-strain elasticity modulus (Ep) = (Ps – Pd)/[(Ds – Dd)/Dd]. The local pulse wave velocity (PWV) was derived from the β index, and the ∆P was the difference between the maximal pressure and the pressure at the first peak on the carotid pressure waveform (Figure 1). All measurements were performed by the same doctor who conducted the ultrasounds throughout the study.
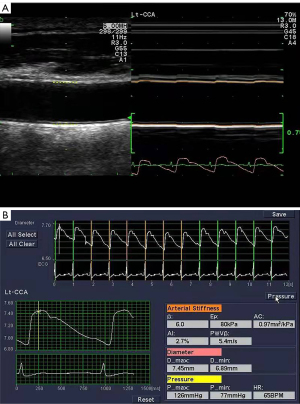
Assessment of clinical characteristics
We collected the following baseline data: age (y), male gender (%), height (cm), body mass index (BMI; kg/m²), systolic blood pressure (SBP; mmHg), diastolic blood pressure (DBP; mmHg), glucose level (Glu, mmol/L), glycated hemoglobin [GHb; % of total hemoglobin (HbA)], creatinine (Cre, mmol/L), low density lipoprotein cholesterol (LDL-C, mmol/L), and tHcy (umol/L). Smoking history was defined as having smoked for more than 10 years; hypertension was defined as SBP ≥90 mmHg, DBP ≥140 mmHg, or the use of antihypertensive drugs; diabetes mellitus (DM) was defined as fasting serum glucose ≥126 mg/dL or the use of antidiabetic medications; and hyperlipidemia was defined as total fasting serum cholesterol >5.72 mmol/L or triglycerides >1.70 mmol/L in adults. Serum creatinine was measured by the enzymatic method, with the reference range of serum creatinine 59–106 µmol/L for males and 45–88 µmol/L for females. The level of GHb was determined by reverse phase cation exchange chromatography, and the reference value range of GHb was 4.0% to 6.0%. In the morning, fasting brachial venous blood samples (10 mL) were collected from all participants, and biochemical parameters were determined by an automatic chemiluminescence analyzer (IMMULITE 2000, Siemens Healthineers, Erlangen, Germany).
Statistical analysis
Statistical analysis was performed using the software SPSS 25.0 (IBM Corp., Armonk NY, USA). The relevant data showed normal distribution. For demographic and clinical information, comparisons of categorical variables were made using the chi-square test while continuous variables were analyzed by the independent two-sample t-test. Univariate correlational analysis was conducted to assess the relationship between arterial stiffness parameters and tHcy concentration in the CSFP group. Logistic regression analysis was used to ascertain independent risk factors for CSFP. A receiver operating characteristic (ROC) curve was adopted to display the classification performance of the detected risk factors for CSFP. For all statistical analyses, a value of P<0.05 was considered significant.
Results
Demographic and clinical characteristics
Table 1 presents the clinical characteristics of participants with coronary normal flow and slow flow phenomena. There were no significant differences in age, gender, height, BMI, heart rate, Ps, Pd, fasting blood glucose, GHb level, Cre level, LDL-C level, intima-media thickness (IMT), smoking history, and DM between the 2 groups (P>0.05 for all baseline data measurements). However, the serum tHcy level in the CSFP group was significantly higher than that in the control group (19.95±4.00 vs. 9.12±2.72; P=0.009). Mean TIMI frame count for the major epicardial coronary artery was 48.60±11.30 in the CSFP group and 24.50±3.80 in the control group (P=0.001).
Table 1
Variables | Control group (n=73) | CSFP group (n=73) | P value |
---|---|---|---|
Male gender (%) | 56% | 59% | 0.84 |
Age (years) | 60.50±10.20 | 59.30±12.50 | 0.50 |
Height (cm) | 167.76±6.18 | 167.85±7.03 | 0.85 |
BMI (kg/m2) | 24.63±2.33 | 25.22±3.09 | 0.63 |
SBP (mmHg) | 128.40±12.36 | 131.86±11.14 | 0.45 |
DBP (mmHg) | 75.66±10.11 | 76.87±11.24 | 0.59 |
Glucose (mmol/L) | 6.21±2.33 | 6.29±2.16 | 0.45 |
GHb (%) | 5.78±0.68 | 5.92±0.61 | 0.86 |
Creatinine (mmol/L) | 71.21±14.08 | 71.08±15.11 | 0.87 |
LDL-C (mg/dL) | 2.40±0.68 | 2.55±0.54 | 0.57 |
IMT (mm) | 1.10±0.20 | 1.23±0.14 | 0.12 |
Mean TIMI frame count | 24.50±3.80 | 48.60±11.30 | 0.001 |
tHcy (μmol/L) | 9.12±2.72 | 19.95±4.00 | 0.009 |
Hypertension (%) | 63% | 66% | 0.82 |
DM (%) | 18% | 22% | 0.32 |
Hyperlipidemia (%) | 12% | 15% | 0.76 |
History of smoking (%) | 19% | 24% | 0.47 |
BMI, body mass index; SBP, systolic blood pressure; DBP, diastolic blood pressure; GHb, glycated hemoglobin; LDL-C, low density lipoprotein; IMT, intima-media thickness; tHcy, total serum homocysteine; TIMI, thrombolysis in myocardial infarction; DM, diabetes mellitus.
Comparison of carotid artery stiffness parameters between CSFP group and control group
Table 2 shows the quantitative parameters of carotid artery stiffness. The values of β index (10.75±2.16 vs. 9.02±2.11; P=0.007), Ep (147.41±41.22 vs. 116.21±39.21; P=0.004), and PWV (7.45±1.23 vs. 6.16±1.20; P=0.003) in the CSFP group were significantly higher than those in the control group, but AC (0.52±0.11 vs. 0.69±0.24; P=0.008) was lower in the CSFP group than in the control group.
Table 2
Parameters | Control group (n=73) | CSFP group (n=73) | P value |
---|---|---|---|
β index | 9.02±2.11 | 10.75±2.16 | 0.007 |
Ep (kPa) | 116.21±39.21 | 147.41±41.22 | 0.004 |
AC (mm2/kPa) | 0.69±0.24 | 0.52±0.11 | 0.008 |
Aix (%) | 17.06±13.00 | 15.03±9.29 | 0.235 |
PWV (m/s) | 6.16±1.20 | 7.45±1.23 | 0.003 |
D-min (mm) | 7.12±0.65 | 7.42±0.14 | 0.622 |
D-max (mm) | 7.87±0.33 | 7.54±0.83 | 0.833 |
β index, stiffness parameter; Ep, pressure-strain elasticity modulus; AC, arterial compliance; AIx, augmentation index; PWV, local pulse wave velocity; D-min, minimal diameter; D-max, maximal diameter; CSFP, coronary slow flow phenomenon.
Relationship between carotid artery stiffness parameters and tHcy levels in patients with CSFP
Carotid artery stiffness correlated with serum tHcy concentration in CSFP patients (Figure 2). Correlation analysis revealed a significant positive correlation between serum tHcy levels and β (R value =0.494; P<0.0001), Ep (R value =0.469; P<0.0001) and PWV (R value =0.436; P<0.0001) However, there was a significant negative correlation between serum tHcy levels and AC (R value =−0.230; P=0.022).
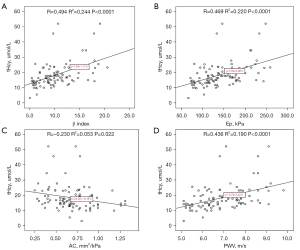
Independent predictors of CSFP
Logistic regression analysis showed that tHcy concentration, left PWV, right PWV, left β index, and right β index were all predictors of CSFP (Table 3). Among them, the left β index and right β index had the best prediction effect on CSFP, and the left PWV and right PWV also had better performance (Figure 3). The cutoff values for these factors were 9.3, 9.3, 6.7, and 6.6, respectively (Table 3).
Table 3
Variables | AUC | Sensitivity | Specificity | Cutoff | 95% CI | Youden index |
---|---|---|---|---|---|---|
Left β index | 0.95 | 94.5% | 89.0% | 9.3 | 0.91–0.98 | 0.83 |
Right β index | 0.95 | 98.6% | 86.3% | 9.3 | 0.91–0.98 | 0.84 |
Left PWV | 0.92 | 89.0% | 84.9% | 6.7 | 0.86–0.95 | 0.74 |
Right PWV | 0.91 | 93.2% | 78.1% | 6.6 | 0.85–0.95 | 0.71 |
tHcy | 0.79 | 64.4% | 85.9% | 17.8 | 0.71–0.85 | 0.50 |
tHcy, total serum homocysteine; β index, stiffness parameter; PWV, local pulse wave velocity; AUC, area under the curve; CI, confidence interval; CSFP, coronary slow flow phenomenon.
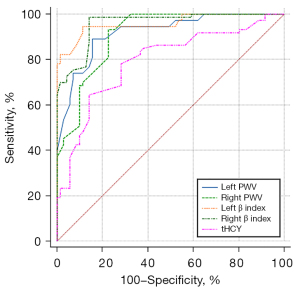
Discussion
Our study found that serum tHcy level in the CSFP group was significantly higher than that in the control group. High serum tHcy concentration is associated with the slow flow phenomenon in nonstenotic coronary arteries. Evrengul et al. found elevated tHcy levels in patients with slow coronary flow but otherwise normal coronary arteries, compared to those with normal coronary flow (19). They concluded that elevated tHcy levels might be related to endothelial dysfunction. A study performed by Barutcu et al. (5) on the relationship between tHcy concentration and CSFP found that tHcy levels increased in cases with slow coronary blood flow. Yurtdaş et al. (20) investigated plasma tHcy concentrations in patients with CSFP before and at the end of an exercise trial and compared them with healthy controls. The results showed that the tHcy values of the CSFP patients were higher than those of the control group, and this difference persisted after the exercise test. This is consistent with previous research. These results allow consideration of a necessary pathophysiologic association between elevated plasma tHcy levels and CSFP. Serum tHcy is the intermediate metabolite produced in vivo in essential amino acids after methionine demethylation, which binds to the N-methyl-D-aspartate receptor and plays a role in oxidative stress, apoptosis, and endothelial dysfunction (21,22). Histopathologic studies have shown the existence of capillary and endothelial damage in CSFP patients (23,24). In adult hyperhomocysteinemia, high tHcy concentrations are associated with impaired, blood flow-mediated, endothelium-dependent vasodilatation (9,25). Endothelial dysfunction and oxidative stress caused by elevated tHcy may be responsible for coronary slow flow (26).
Our results showed the stiffness parameters of β index, Ep, and PWV in the CSFP group were significantly higher than those in the control group and AC was lower in the CSFP group than in the control group. It was suggested that patients with CSFP showed signs of carotid arteriosclerosis, namely, decreased arterial compliance and increased arterial stiffness, although there was no coronary artery stenosis (27). As far as we know, this was the first time the relationship between tHcy concentration and carotid artery stiffness parameters had been evaluated. The results of our study showed that serum tHcy concentration was positively correlated with β, Ep, and PWV and negatively correlated with AC in the CSFP group. Several studies have found that changes in arterial stiffness play an important role in the occurrence and development of cerebrovascular disease; furthermore, arterial stiffness is an important factor affecting the myocardial ischemic threshold, and decreased arterial stiffness can reduce artery flow (28,29). Atherosclerosis is caused by the accumulation of tHcy and lipoprotein with microorganisms that obstruct the formation of blood vessels during the formation of vulnerable plaques (30). It has been shown that increasing the tHcy concentration prompts the production of oxygen free radicals, injury to vascular endothelial cells, reduction of nitric oxide release, and attenuation of vascular diastolic function (31). Studies have shown that tHcy can promote the oxidation of LDL-C and stimulate the valuable addition of vascular smooth muscle cells (32). Other researchers have also found (33) that increased tHcy concentrations and other risk factors play a coordinating role in the promotion of cardiovascular disease. Increased tHcy levels significantly promote blood vessel damage caused by risk factors, such as smoking, a high cholesterol diet, and hypertension, and double the risk of cardiovascular disease (34). It is recognized that tHcy is a risk factor for the development of atherosclerosis and thrombotic complications. Endothelial injury may contribute to the early onset of atherogenic events and may also be involved in a mechanism of tHcy-induced vascular disease (35).
Our study found that the tHcy level, left PWV, right PWV, left β index, and right β index were independently associated with CSFP. Moreover, the best predictors of CSFP were the left β index and right β index, and the left PWV and right PWV were also relatively good predictors of CSFP. The cutoff values of these factors were 9.3, 9.3, 6.7, and 6.6, respectively. Beltrame et al. (36) conducted a study of the Australian population and found that male gender and smoking were independent risk factors for CSFP. A study of the Iranian population found that diabetes, hypertension, and opioid abuse were independent risk factors associated with coronary slow flow (37). In our study, however, none of these factors were found to be predictors of CSFP. The possible explanation is that the prevalence of diabetes and hypertension was similar in both groups and there was no statistical difference in GHb, LDL-C, creatinine, or hyperlipidemia; therefore, these factors could not be included in the logistic regression analysis. It is hypothesized that any one or more comorbidities that cause endothelial dysfunction may contribute to the presentation of CSFP.
Limitations
This study had several limitations. First, a history of antianginal or antiplatelet therapy might have affected the frame count results of coronary angiography. Second, information on some factors that affect tHcy levels, such as dietary patterns, food folic acid fortification, and vitamin supplements, was lacking in our study. This might have caused the strength of any association to be underestimated (38). In addition, we only studied the relationship between tHcy level and carotid artery stiffness parameters in CSFP patients. No subgroup analysis was performed for the sampled arteries with and without stenosis. This was due to the insufficient number of subgroup cases. Conversely, patients with moderate and/or severe arterial stenosis were excluded from the study.
Conclusions
In summary, we have shown that patients with CSFP have increased serum tHcy levels compared with controls who have normal coronary flow. In addition, by using high-resolution ultrasonographic technology, our study highlighted the relationship between tHcy and carotid artery stiffness parameters in patients with CSFP, providing information both on tHcy and local carotid artery stiffness parameters. The tHcy concentration was positively correlated with β, Ep, and PWV, but negatively correlated with AC. Moreover, we found that tHcy concentration, left PWV, right PWV, left β index, and right β index were all predictors of CSFP. Among them, the left β index and right β index were the best indicators for predicting CSFP, and these cutoff values were 9.3 and 9.3, respectively. These findings might contribute to a better comprehension of CSFP and the emerging evidence supporting its role in the development of atherosclerosis.
Acknowledgments
Funding: The study was supported by the National Natural Science Foundation of China (No. 81730050).
Footnote
Reporting Checklist: The authors have completed the STROBE reporting checklist. Available at https://qims.amegroups.com/article/view/10.21037/qims-21-931/rc
Conflicts of Interest: All authors have completed the ICMJE uniform disclosure form (available at https://qims.amegroups.com/article/view/10.21037/qims-21-931/coif). The authors have no conflicts of interest to declare.
Ethical Statement: The authors are accountable for all aspects of the work in ensuring that questions related to the accuracy or integrity of any part of the work are appropriately investigated and resolved. The study was conducted in accordance with the Declaration of Helsinki (as revised in 2013). The study was approved by the Institutional Review Board of Beijing Tiantan Hospital. All participants gave written informed consent before ultrasound examinations.
Open Access Statement: This is an Open Access article distributed in accordance with the Creative Commons Attribution-NonCommercial-NoDerivs 4.0 International License (CC BY-NC-ND 4.0), which permits the non-commercial replication and distribution of the article with the strict proviso that no changes or edits are made and the original work is properly cited (including links to both the formal publication through the relevant DOI and the license). See: https://creativecommons.org/licenses/by-nc-nd/4.0/.
References
- Tambe AA, Demany MA, Zimmerman HA, Mascarenhas E. Angina pectoris and slow flow velocity of dye in coronary arteries--a new angiographic finding. Am Heart J 1972;84:66-71. [Crossref] [PubMed]
- Kopetz V, Kennedy J, Heresztyn T, Stafford I, Willoughby SR, Beltrame JF. Endothelial function, oxidative stress and inflammatory studies in chronic coronary slow flow phenomenon patients. Cardiology 2012;121:197-203. [Crossref] [PubMed]
- Riza Erbay A, Turhan H, Yasar AS, Ayaz S, Sahin O, Senen K, Sasmaz H, Yetkin E. Elevated level of plasma homocysteine in patients with slow coronary flow. Int J Cardiol 2005;102:419-23. [Crossref] [PubMed]
- Esse R, Barroso M, Tavares de Almeida I, Castro R. The Contribution of Homocysteine Metabolism Disruption to Endothelial Dysfunction: State-of-the-Art. Int J Mol Sci 2019;20:867. [Crossref] [PubMed]
- Barutcu I, Sezgin AT, Sezgin N, Gullu H, Esen AM, Topal E, Ozdemir R. Elevated plasma homocysteine level in slow coronary flow. Int J Cardiol 2005;101:143-5. [Crossref] [PubMed]
- Evrengul H, Tanriverdi H, Kuru O, Enli Y, Yuksel D, Kilic A, Kaftan A, Kirac S, Kilic M. Elevated homocysteine levels in patients with slow coronary flow: relationship with Helicobacter pylori infection. Helicobacter 2007;12:298-305. [Crossref] [PubMed]
- Tanriverdi H, Evrengul H, Tanriverdi S, Kuru O, Seleci D, Enli Y, Kaftan A, Kilic M. Carotid intima-media thickness in coronary slow flow: relationship with plasma homocysteine levels. Coron Artery Dis 2006;17:331-7. [Crossref] [PubMed]
- Ascione L, De Michele M, Accadia M, Rumolo S, Sacra C, Alberta Ortali V, Inserviente L, Petti M, Russo G, Tuccillo B. Effect of acute hyperhomocysteinemia on coronary flow reserve in healthy adults. J Am Soc Echocardiogr 2004;17:1281-5. [Crossref] [PubMed]
- Woo KS, Chook P, Lolin YI, Cheung AS, Chan LT, Sun YY, Sanderson JE, Metreweli C, Celermajer DS. Hyperhomocyst(e)inemia is a risk factor for arterial endothelial dysfunction in humans. Circulation 1997;96:2542-4. [Crossref] [PubMed]
- Cahill M, Karabatzaki M, Meleady R, Refsum H, Ueland P, Shields D, Mooney D, Graham I. Raised plasma homocysteine as a risk factor for retinal vascular occlusive disease. Br J Ophthalmol 2000;84:154-7. [Crossref] [PubMed]
- Dietrich M, Jacques PF, Polak JF, Keyes MJ, Pencina MJ, Evans JC, Wolf PA, Selhub J, Vasan RS, D'Agostino RB. Segment-specific association between plasma homocysteine level and carotid artery intima-media thickness in the Framingham Offspring Study. J Stroke Cerebrovasc Dis 2011;20:155-61. [Crossref] [PubMed]
- Malinow MR, Nieto FJ, Szklo M, Chambless LE, Bond G. Carotid artery intimal-medial wall thickening and plasma homocyst(e)ine in asymptomatic adults. The Atherosclerosis Risk in Communities Study. Circulation 1993;87:1107-13. [Crossref] [PubMed]
- Sasaki T, Watanabe M, Nagai Y, Hoshi T, Takasawa M, Nukata M, Taguchi A, Kitagawa K, Kinoshita N, Matsumoto M. Association of plasma homocysteine concentration with atherosclerotic carotid plaques and lacunar infarction. Stroke 2002;33:1493-6. [Crossref] [PubMed]
- Larsson SC, Traylor M, Markus HS. Homocysteine and small vessel stroke: A mendelian randomization analysis. Ann Neurol 2019;85:495-501. [Crossref] [PubMed]
- Willum-Hansen T, Staessen JA, Torp-Pedersen C, Rasmussen S, Thijs L, Ibsen H, Jeppesen J. Prognostic value of aortic pulse wave velocity as index of arterial stiffness in the general population. Circulation 2006;113:664-70. [Crossref] [PubMed]
- Boutouyrie P, Tropeano AI, Asmar R, Gautier I, Benetos A, Lacolley P, Laurent S. Aortic stiffness is an independent predictor of primary coronary events in hypertensive patients: a longitudinal study. Hypertension 2002;39:10-5. [Crossref] [PubMed]
- Gibson CM, Cannon CP, Daley WL, Dodge JT Jr, Alexander B Jr, Marble SJ, McCabe CH, Raymond L, Fortin T, Poole WK, Braunwald E. TIMI frame count: a quantitative method of assessing coronary artery flow. Circulation 1996;93:879-88. [Crossref] [PubMed]
- O'Rourke MF, Staessen JA, Vlachopoulos C, Duprez D, Plante GE. Clinical applications of arterial stiffness; definitions and reference values. Am J Hypertens 2002;15:426-44. [Crossref] [PubMed]
- Evrengul H, Tanriverdi H, Enli Y, Kuru O, Seleci D, Bastemir M, Kilic A, Kaftan A, Kilic M. Interaction of plasma homocysteine and thyroid hormone concentrations in the pathogenesis of the slow coronary flow phenomenon. Cardiology 2007;108:186-92. [Crossref] [PubMed]
- Yurtdaş M, Özcan IT, Seyis AS, Çamsarı A, Ciçek D. Plasma homocysteine is associated with ischemic findings without organic stenosis in patients with slow coronary flow. J Cardiol 2013;61:138-43. [Crossref] [PubMed]
- McCully KS. Chemical pathology of homocysteine. IV. Excitotoxicity, oxidative stress, endothelial dysfunction, and inflammation. Ann Clin Lab Sci 2009;39:219-32. [PubMed]
- Shah H, Jan MU, Altaf A, Salahudin M. Correlation of hyper-homocysteinemia with coronary artery disease in absence of conventional risk factors among young adults. J Saudi Heart Assoc 2018;30:305-10. [Crossref] [PubMed]
- Mosseri M, Yarom R, Gotsman MS, Hasin Y. Histologic evidence for small-vessel coronary artery disease in patients with angina pectoris and patent large coronary arteries. Circulation 1986;74:964-72. [Crossref] [PubMed]
- Mangieri E, Macchiarelli G, Ciavolella M, Barillà F, Avella A, Martinotti A, Dell'Italia LJ, Scibilia G, Motta P, Campa PP. Slow coronary flow: clinical and histopathological features in patients with otherwise normal epicardial coronary arteries. Cathet Cardiovasc Diagn 1996;37:375-81. [Crossref] [PubMed]
- Kanani PM, Sinkey CA, Browning RL, Allaman M, Knapp HR, Haynes WG. Role of oxidant stress in endothelial dysfunction produced by experimental hyperhomocyst(e)inemia in humans. Circulation 1999;100:1161-8. [Crossref] [PubMed]
- Yamashita K, Tasaki H, Nagai Y, Suzuka H, Nihei S, Kobayashi K, Horiuchi M, Nakashima Y, Adachi T. Experimental hyperhomocysteinemia impairs coronary flow velocity reserve. Int J Cardiol 2005;104:163-9. [Crossref] [PubMed]
- Okazaki S, Furukado S, Abe Y, Tanaka M, Miwa K, Yamagami H, Sakaguchi M, Sakoda S, Kitagawa K. Association of inflammatory markers and carotid intima-media thickness with the risk of cardiovascular events in high-risk patients. Cerebrovasc Dis 2010;30:180-7. [Crossref] [PubMed]
- Cooper LL, Palmisano JN, Benjamin EJ, Larson MG, Vasan RS, Mitchell GF, Hamburg NM. Microvascular Function Contributes to the Relation Between Aortic Stiffness and Cardiovascular Events: The Framingham Heart Study. Circ Cardiovasc Imaging 2016;9:e004979. [Crossref] [PubMed]
- Adams MR, Nakagomi A, Keech A, Robinson J, McCredie R, Bailey BP, Freedman SB, Celermajer DS. Carotid intima-media thickness is only weakly correlated with the extent and severity of coronary artery disease. Circulation 1995;92:2127-34. [Crossref] [PubMed]
- Memişoğullari R, Yüksel H, Coskun A, Yüksel HK, Yazgan O, Bilgin C. High serum homocysteine levels correlate with a decrease in the blood flow velocity of the ophthalmic artery in highway toll collectors. Tohoku J Exp Med 2007;212:247-52. [Crossref] [PubMed]
- Akintunde AA. Epidemiology of conventional cardiovascular risk factors among hypertensive subjects with normal and impaired fasting glucose. S Afr Med J 2010;100:594-7. [Crossref] [PubMed]
- Schaffer A, Verdoia M, Barbieri L, Cassetti E, Suryapranata H, De Luca G. Impact of Diabetes on Homocysteine Levels and Its Relationship with Coronary Artery Disease: A Single-Centre Cohort Study. Ann Nutr Metab 2016;68:180-8. [Crossref] [PubMed]
- Kennedy BP, Farag NH, Ziegler MG, Mills PJ. Relationship of systolic blood pressure with plasma homocysteine: importance of smoking status. J Hypertens 2003;21:1307-12. [Crossref] [PubMed]
- McCully KS. Homocysteine, Infections, Polyamines, Oxidative Metabolism, and the Pathogenesis of Dementia and Atherosclerosis. J Alzheimers Dis 2016;54:1283-90. [Crossref] [PubMed]
- Tawakol A, Omland T, Gerhard M, Wu JT, Creager MA. Hyperhomocyst(e)inemia is associated with impaired endothelium-dependent vasodilation in humans. Circulation 1997;95:1119-21. [Crossref] [PubMed]
- Beltrame JF, Limaye SB, Horowitz JD. The coronary slow flow phenomenon--a new coronary microvascular disorder. Cardiology 2002;97:197-202. [Crossref] [PubMed]
- Moazenzadeh M, Azimzadeh BS, Zare J, Shokouhi M, Sheikhvatan M. Clinical features and main determinants of coronary slow flow phenomenon in Iranian patients. Eur J Cardiovasc Med 2010;1:2042.
- Clarke R, Lewington S, Donald A, Johnston C, Refsum H, Stratton I, Jacques P, Breteler MM, Holman R. Underestimation of the importance of homocysteine as a risk factor for cardiovascular disease in epidemiological studies. J Cardiovasc Risk 2001;8:363-9. [Crossref] [PubMed]