Calcifications in diffuse leptomeningeal glioneuronal tumors: a case series
Introduction
Diffuse leptomeningeal glioneuronal tumor (DLGNT) is a new entity recognized in the 2016 revision of the World Health Organization (WHO) classification of tumors of the central nervous system (1,2). Previously known in the literature under a variety of similar terms (such as disseminated oligodendroglial-like leptomeningeal tumor of childhood or diffuse leptomeningeal oligodendrogliomatosis) (3-5), this tumor is a rare entity typically seen in the pediatric population with a median age of 8.4 years (6). The neoplastic cells frequently show histological and immunohistochemical features of both glial, defined oligodendroglioma-like cells, and neuronal differentiation, often with expression of synaptophysin in addition to OLIG2 and S-100 (2-4,6-8). The lesions commonly harbor BRAF fusions (KIAA1549-10 BRAF fusion) (3,4) or duplications (9), as well as deletions of chromosome arm 1p, either alone or 11 occasionally combined with 19q (3,4,7,10,11). In contrast to oligodendrogliomas, DLGNT do not have IDH mutations (2,3,6,7,12).
Although most tumors display a slow progression, aggressive cases have been occasionally described (3,5,13,14). To date there are no therapeutic guidelines for this rare entity. Published reports cite the use of various chemotherapy regimens with or without radiation, showing relatively good clinical outcomes with adequate disease control in most cases (2,3,5,6,8,9,15).
Nonetheless, survival varies widely and data on long-term outcome are still lacking. Some authors reported an average survival of 22 months (12), while other series showed a range of survival that ranged from 19 months to 9 years, with a mean follow-up time of 54 months (5).
The classical magnetic resonance imaging (MRI) appearance of these tumors consists of marked subarachnoid/leptomeningeal enhancement and tiny T2-hyperintense pseudo-cystic lesions along the subpial surface of the neuroaxis, especially in the cerebellum, brainstem, and spinal cord (2,16,17-19). Communicating hydrocephalus is frequently associated (6). Although, by definition, DLGNT should not display significant parenchymal involvement and/or previous parenchymal tumor, few subjects presented an intraparenchymal lesion component, commonly in the spinal cord (12,20). Furthermore, in a recent study by Chiang et al. comprising five patients, leptomeningeal dissemination was not radiologically detected (1,21), suggesting that DLGNT do not necessarily present with gross leptomeningeal dissemination on MRI (21,22).
Here, we report a novel neuroradiological feature, consisting of a peculiar pattern of progressive leptomeningeal calcifications in three young subjects with DLGNT with long-term clinical and neuroradiological follow-up.
We present the following article in accordance with the CARE reporting checklist (available at https://qims.amegroups.com/article/view/10.21037/qims-21-741/rc).
Case presentation
Three patients with histologically proven DLGNT (2 males and 1 female, median age 12.4 years, range, 9.4–25.8 years) were identified from the institutional database of pediatric patients treated for tumors at the Gaslini Children’s Hospital between 2010 and 2020. The median age at diagnosis was 5 years (range, 2–13 years) and mean follow-up time was 9.5 years (range, 8.4–12.8 years). In all our cases an immunohistochemical study of the biopsied pathological tissue using antibodies for IDH1 (R132H), GFAP, Olig2, S100 and synaptophysin was performed, detecting the following pattern: IDH1 negative, GFAP negative, OLIG2 positive, S100 positive, synaptophysin positive (Figure 1).
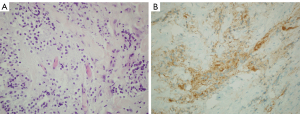
In 2/3 cases clinical presentation at onset included photophobia and vomiting related to a communicating hydrocephalus which was confirmed by brain CT or MRI examinations while the third case had symptoms more nuanced and non-specific, characterized by awakening at night, confusional state, and dysarthria. All patients were evaluated with brain and spinal MRI that in 2/3 cases showed multiple small cysts scattered on the surface of cerebellum, brainstem, and spinal cord (Figure 2A-2C); in the older subject, small cystic lesions were present only on the cerebellar surface. In all cases, contrast-enhanced T1-weighted images revealed diffuse leptomeningeal enhancement mainly at level of cerebellar subarachnoid spaces, basal cisterns, and spinal cord with no evidence of intraparenchymal involvement (Figure 2D-2G).
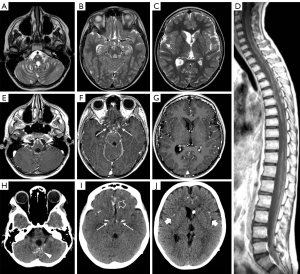
Calcifications were present at clinical onset in the older subject and appeared about 2 years after clinical onset in the other two. They were mainly located in the basal cisterns, sylvian fissures, on the posterior surface of the thalami, and within cerebral/cerebellar hemispheric sulci (Figure 2H-2J). At first, calcifications were mostly visible as slight hypointensities on T2* gradient echo (GE) or susceptibility weighted images (SWI) corresponding to subtle hyperdensities on CT scan. Over time, multiple MRI and CT examinations showed reduction of the leptomeningeal enhancement and a progressive increase in signal and density alterations referable to calcifications (Figure 3). Figure 4 shows progression of calcifications on multiple head CT examinations over time in each patient.
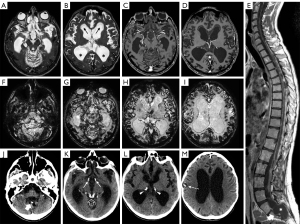
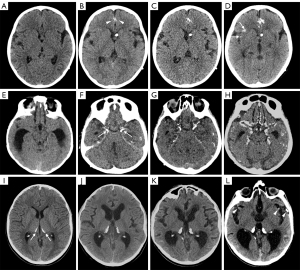
Patients underwent different schemes of chemotherapy, variably associated with craniospinal irradiation and/or bevacizumab, with disease stabilization in one case, disease regression but demise after bilateral cerebrovascular accidents and radiation induced leukoencephalopathy in the second case (Figure 5), and disease progression in the third case. In all cases, calcifications were present before starting craniospinal irradiation and/or treatment with bevacizumab; in one of the three cases subtle calcifications were already present at diagnosis.
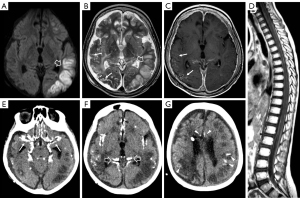
Clinical and neuroradiological features of each patient are described in detail as Supplementary file (Appendix 1). All procedures performed in this study were in accordance with the ethical standards of the institutional and/or national research committee(s) and with the Helsinki Declaration (as revised in 2013). Written informed consent was obtained from the patients’ parents or legal guardians for publication of this case report and accompanying images. A copy of the written consent is available for review by the editorial office of this journal.
Discussion
In this case series, we report a peculiar pattern of intracranial calcifications detected on MRI and CT studies in three patients with typical radiological features and histological diagnosis of DLGNT.
The localization of calcium deposits was similar in all cases and corresponded to the regions classically involved by leptomeningeal contrast enhancement in DLGNT. In particular, calcifications were mainly located in the basal cisterns, sylvian fissures, on the posterior surface of the thalami, and within cerebral hemispheric sulci. We did not perform T2* GE sequences or CT scan at the spinal level. Therefore, we are unaware if calcifications may also be present around the spinal cord, along the spinal roots or at the level of neoplastic tissue in thecal sac, where the biopsies were performed. Interestingly, few nodular calcifications were recently noted by Tiwari et al. along the left sylvian fissure in a 3-year-old girl with a DLGNT on a head CT at clinical onset (23). Unfortunately, in this case, the biopsy was performed along the contralateral sylvian fissure, and histopathological correlation is unavailable also in this case (23). Recently, Lakhani et al. described a case of DLGNT with an intracranial calcified parenchymal mass lesion in the right basal ganglia at the onset (24); in this case, the coarse intraparenchymal calcific deposit seems to differ from those illustrated in our cases in which the calcifications turn out to be a peculiar pattern of leptomeningeal calcifications which seem to be a characteristic of this neoplasm if followed over time. These types of calcifications are different from those we might find in other primary central nervous system tumors that present as partly calcified intraparenchymal masses such as oligodendroglioma or calcifying pseudoneoplasms of neuroaxis (CAPNON), which usually occur as extra- axial, or less often intra-axial, solitary calcified masses.
Of note, in the rare cases reported in literature in which a histopathologic evaluation of DLGNT was performed during long-term follow-up or at autopsy, no calcium deposits have been described (3,25). Histologically, DLGNT are described as low- to moderate-cellularity lesions consisting of relatively monomorphous oligodendrocyte-like cells with round to oval nuclei, inconspicuous nucleoli and glioneuronal elements, embedded in a plentiful desmoplastic or myxoid leptomeningeal stroma (1-3,5,22). Progressive deposition of calcium in the context of this desmoplastic tissue might be a possible explanation for leptomeningeal calcifications in DLGNT. Indeed, calcifications have been described in other glioneuronal tumors, particularly solid or solid-cystic tumors like ganglioglioma (30%), desmoplastic infantile ganglioglioma (20%), papillary glioneuronal tumors (75%) and rosette-forming glioneuronal tumor (25%) (26-28).
Since the first report by Harwood-Nash et al. (29), calcifications are considered the most common radiological manifestations of radiation-related brain injury, though the underlying mechanism is not yet completely clear. It has been suggested that post-radiation intracerebral calcifications could be related to mineralizing microangiopathy or dystrophic calcifications developed in the perivascular brain tissue secondary to the leakage of plasma fluid from damaged vessels, and regional ischemia due to microcirculation impairment (30). However, in the present series, only one subject was treated with radiotherapy, and intracranial calcifications appeared well before the onset of radiation treatment. Of note, in this patient, we observed a dramatic evolution with radiation induced leukoencephalopathy associated with right middle cerebral artery infarct that could have been triggered or facilitated by presence of calcifications around the arterial walls in the basal cisterns.
Systemic chemotherapy could also induce the formation of calcifications within tumors. It has been hypothesized that chemotherapy may lead to a pH rise with alkalization of the tumor environment and subsequent degeneration of the neoplastic tissues followed by calcifications of necrotic components (31). However, there is still no absolute certainty about the mechanism and no specific treatment has been clearly indicated as responsible. Indeed, the appearance of calcifications has not been systematically reported in patients with low-grade brain tumors treated with International Society of Paediatric Oncology-Low Grade Glioma Subcommittee (SIOP-LGG) chemotherapy regimens. Therefore, we believe that calcifications in DLGNT are not related to radiotherapy and are unlikely the consequence of chemotherapy. Of note, two patients in this series were treated with bevacizumab. Bevacizumab is a humanized monoclonal antibody that works by inhibiting the action of VEGF, a specific angiogenesis growth factor that binds to receptors on blood vessels and stimulates the formation of new blood vessels.
Bevacizumab is the prototype of an antiangiogenic agent and has broad antitumor activity. It has been used in DLGNT, individually or in combination with radiotherapy, chemotherapy and/or other targeted therapies, with mixed efficacy (4,9,13,14,32,33). Bevacizumab may cause intratumoral calcifications in high-grade gliomas, likely through a vascular mechanism (34). It has been hypothesized that bevacizumab can lead to hypoxia of the neoplastic tissue with consequent breakdown of the normal calcium gradient across the cell membrane and accumulation of calcium inside the cell and in the extracellular space after cell death (31,33). Of note, in the two patients treated with bevacizumab, calcifications were already present before the beginning of this therapy. Therefore, we suppose that bevacizumab might perhaps have had a contributory effect but not a primary role in the development of leptomeningeal calcium deposits.
Interestingly, in our series, the number and extension of calcifications progressively increased, starting from few linear/nodular leptomeningeal depositions and slowly progressing to a more diffuse pattern of leptomeningeal involvement. At the onset, although calcifications could be depicted on brain MRI as faint, subtle hypointensities on T2* GE or susceptibility-weighted images, these were better appreciated as linear or nodular leptomeningeal hyperdensities on head CT examinations. Conversely, in one subject (Appendix 1, case 3), several years after clinical onset and before bevacizumab therapy, we noticed a discrepancy between the evolution of these imaging features on CT and MRI studies. Indeed, leptomeningeal calcifications on head CT were less extensive and conspicuous compared to the linear hypointensities depicted on T2* GE or susceptibility-weighted sequences on brain MRI. Moreover, these hypointensities also involved the cortex of both cerebral hemispheres. This finding suggests that cortical neurodegenerative processes might be associated with leptomeningeal calcium deposits in DLGNT in later stages. Remarkably, the patient presented signs of cognitive impairment supporting a neurodegenerative process. However, we lack cortical histological data, and the nature of these T2*-hypointense cortical lesions in DLGNT remains unknown.
In conclusion, we reported a novel peculiar pattern of progressive leptomeningeal calcifications in DLGNT, thus expanding the imaging phenotype of this rare neoplasm. Taking into consideration the onset, evolution of this neuroimaging feature and the absence of direct relationship with treatments, we hypothesize that calcifications in DLGNT might be the effect of natural changes in the tumor and its environment. However, larger longitudinal studies with histopathological correlation are needed to understand the real nature of this neuroimaging finding, the underlying mechanisms, and the potential contributing role of oncology treatment.
Acknowledgments
We acknowledge all the members of the pediatric brain tumors multidisciplinary team of the IRCCS Istituto Giannina Gaslini, who were involved in the care of these patients.
Funding: This study was funded by from “Associazione per la Ricerca sui Tumori Cerebrali del Bambino” and from “Fondazione Berlucchi” to MLG (Neuroncology Unit, IRCCS Istituto Giannina Gaslini).
Footnote
Reporting Checklist: The authors have completed the CARE reporting checklist. Available at https://qims.amegroups.com/article/view/10.21037/qims-21-741/rc
Conflicts of Interest: All authors have completed the ICMJE uniform disclosure form (available at https://qims.amegroups.com/article/view/10.21037/qims-21-741/coif). The authors have no conflicts of interest to declare.
Ethical Statement: The authors are accountable for all aspects of the work in ensuring that questions related to the accuracy or integrity of any part of the work are appropriately investigated and resolved. All procedures performed in this study were in accordance with the ethical standards of the institutional and/or national research committee(s) and with the Helsinki Declaration (as revised in 2013). Written informed consent was obtained from the patients’ parents or legal guardians for publication of this case report and accompanying images. A copy of the written consent is available for review by the editorial office of this journal.
Open Access Statement: This is an Open Access article distributed in accordance with the Creative Commons Attribution-NonCommercial-NoDerivs 4.0 International License (CC BY-NC-ND 4.0), which permits the non-commercial replication and distribution of the article with the strict proviso that no changes or edits are made and the original work is properly cited (including links to both the formal publication through the relevant DOI and the license). See: https://creativecommons.org/licenses/by-nc-nd/4.0/.
References
- Louis DN, Perry A, Wesseling P, Brat DJ, Cree IA, Figarella-Branger D, Hawkins C, Ng HK, Pfister SM, Reifenberger G, Soffietti R, von Deimling A, Ellison DW. The 2021 WHO classification of tumors of the central nervous system: a summary. Neuro Oncol 2021;23:1231-51. [Crossref] [PubMed]
- Gardiman MP, Fassan M, Orvieto E, D'Avella D, Denaro L, Calderone M, Severino M, Scarsello G, Viscardi E, Perilongo G. Diffuse leptomeningeal glioneuronal tumors: a new entity? Brain Pathol 2010;20:361-6. [Crossref] [PubMed]
- Rodriguez FJ, Perry A, Rosenblum MK, Krawitz S, Cohen KJ, Lin D, Mosier S, Lin MT, Eberhart CG, Burger PC. Disseminated oligodendroglial-like leptomeningeal tumor of childhood: a distinctive clinicopathologic entity. Acta Neuropathol 2012;124:627-41. [Crossref] [PubMed]
- Rodriguez FJ, Schniederjan MJ, Nicolaides T, Tihan T, Burger PC, Perry A. High rate of concurrent BRAF-KIAA1549 gene fusion and 1p deletion in disseminated oligodendroglioma-like leptomeningeal neoplasms (DOLN). Acta Neuropathol 2015;129:609-10. [Crossref] [PubMed]
- Preuss M, Christiansen H, Merkenschlager A, Hirsch FW, Kiess W, Müller W, Kästner S, Henssler A, Pekrun A, Hauch H, Nathrath M, Meixensberger J, Pietsch T, Kuchelmeister K. Disseminated oligodendroglial-like leptomeningeal tumors: preliminary diagnostic and therapeutic results for a novel tumor entity corrected. J Neurooncol 2015;124:65-74. [Crossref] [PubMed]
- Chen W, Kong Z, Fu J, Zhao D, Wang R, Ma W, Wang Y. Diffuse leptomeningeal glioneuronal tumour (DLGNT) with hydrocephalus as an initial symptom: a case-based update. Childs Nerv Syst 2020;36:459-68. [Crossref] [PubMed]
- Schniederjan MJ, Alghamdi S, Castellano-Sanchez A, Mazewski C, Brahma B, Brat DJ, Brathwaite CD, Janss AJ. Diffuse leptomeningeal neuroepithelial tumor: 9 pediatric cases with chromosome 1p/19q deletion status and IDH1 (R132H) immunohistochemistry. Am J Surg Pathol 2013;37:763-71. [Crossref] [PubMed]
- Aguilera D, Castellino RC, Janss A, Schniederjan M, McNall R, MacDonald T, Mazewski C. Clinical responses of patients with diffuse leptomeningeal glioneuronal tumors to chemotherapy. Childs Nerv Syst 2018;34:329-34. [Crossref] [PubMed]
- Dodgshun AJ, SantaCruz N, Hwang J, Ramkissoon SH, Malkin H, Bergthold G, Manley P, Chi S, MacGregor D, Goumnerova L, Sullivan M, Ligon K, Beroukhim R, Herrington B, Kieran MW, Hansford JR, Bandopadhayay P. Disseminated glioneuronal tumors occurring in childhood: treatment outcomes and BRAF alterations including V600E mutation. J Neurooncol 2016;128:293-302. [Crossref] [PubMed]
- Deng MY, Sill M, Chiang J, Schittenhelm J, Ebinger M, Schuhmann MU, et al. Molecularly defined diffuse leptomeningeal glioneuronal tumor (DLGNT) comprises two subgroups with distinct clinical and genetic features. Acta Neuropathol 2018;136:239-53. [Crossref] [PubMed]
- Nambirajan A, Suri V, Kedia S, Goyal K, Malgulwar PB, Khanna G, Panda PK, Gulati S, Garg A, Sharma MC. Paediatric diffuse leptomeningeal tumor with glial and neuronal differentiation harbouring chromosome 1p/19q co-deletion and H3.3 K27M mutation: unusual molecular profile and its therapeutic implications. Brain Tumor Pathol 2018;35:186-91. [Crossref] [PubMed]
- Cho HJ, Myung JK, Kim H, Park CK, Kim SK, Chung CK, Choi SH, Park SH. Primary diffuse leptomeningeal glioneuronal tumors. Brain Tumor Pathol 2015;32:49-55. [Crossref] [PubMed]
- Yamasaki T, Sakai N, Shinmura K, Kawaji H, Koizumi S, Samashima T, Namba H. Anaplastic changes of diffuse leptomeningeal glioneuronal tumor with polar spongioblastoma pattern. Brain Tumor Pathol 2018;35:209-16. [Crossref] [PubMed]
- Kessler BA, Bookhout C, Jaikumar S, Hipps J, Lee YZ. Disseminated oligodendroglial-like leptomeningeal tumor with anaplastic progression and presumed extraneural disease: case report. Clin Imaging 2015;39:300-4. [Crossref] [PubMed]
- Lyle MR, Dolia JN, Fratkin J, Nichols TA, Herrington BL. Newly identified characteristics and suggestions for diagnosis and treatment of diffuse leptomeningeal glioneuronal/neuroepithelial tumors: a case report and review of the literature. Child Neurol Open 2015;2:2329048X14567531.
- Karlowee V, Kolakshyapati M, Amatya VJ, Takayasu T, Nosaka R, Sugiyama K, Kurisu K, Yamasaki F. Diffuse leptomeningeal glioneuronal tumor (DLGNT) mimicking Whipple's disease: a case report and literature review. Childs Nerv Syst 2017;33:1411-4. [Crossref] [PubMed]
- Lee JK, Ko HC, Choi JG, Lee YS, Son BC. A case of diffuse leptomeningeal glioneuronal tumor misdiagnosed as chronic tuberculous meningitis without brain biopsy. Case Rep Neurol Med 2018;2018:1391943. [Crossref] [PubMed]
- Ghoniya R, Raheja A. Imaging in diffuse leptomeningeal glioneuronal tumor. Neurol India 2019;67:615-6. [Crossref] [PubMed]
- Demir MK, Yapıcıer O, Yılmaz B, Kılıç T. Magnetic resonance imaging findings of mixed neuronal-glial tumors with pathologic correlation: a review. Acta Neurol Belg 2018;118:379-86. [Crossref] [PubMed]
- Xu H, Chen F, Zhu H, Luo L, Zhang R. Diffuse leptomeningeal glioneuronal tumor in a Chinese adult: a novel case report and review of literature. Acta Neurol Belg 2020;120:247-56. [Crossref] [PubMed]
- Chiang JCH, Harreld JH, Orr BA, Sharma S, Ismail A, Segura AD, Ellison DW. Low-grade spinal glioneuronal tumors with BRAF gene fusion and 1p deletion but without leptomeningeal dissemination. Acta Neuropathol 2017;134:159-62. [Crossref] [PubMed]
- Kang JH, Buckley AF, Nagpal S, Fischbein N, Peters KB. A diffuse leptomeningeal glioneuronal tumor without diffuse leptomeningeal involvement: detailed molecular and clinical characterization. J Neuropathol Exp Neurol 2018;77:751-6. [Crossref] [PubMed]
- Tiwari S, Yadav T, Pamnani J, Mathew JM, Elhence P, Praneeth K, Vedant D, Khera PS, Garg P, Vyas V. Diffuse leptomeningeal glioneuronal tumor: a unique leptomeningeal tumor entity. World Neurosurg 2020;135:297-300. [Crossref] [PubMed]
- Lakhani DA, Mankad K, Chhabda S, Feizi P, Patel R, Sarma A, Pruthi S. Diffuse Leptomeningeal Glioneuronal Tumor of Childhood. AJNR Am J Neuroradiol 2020;41:2155-9. [Crossref] [PubMed]
- Gardiman MP, Fassan M, Nozza P, Orvieto E, Garrè ML, Milanaccio C, Severino M, Perilongo G, Giangaspero F. Diffuse leptomeningeal glioneuronal tumours: clinico-pathological follow-up. Pathologica 2012;104:428-31. [PubMed]
- Shin JH, Lee HK, Khang SK, Kim DW, Jeong AK, Ahn KJ, Choi CG, Suh DC. Neuronal tumors of the central nervous system: radiologic findings and pathologic correlation. Radiographics 2002;22:1177-89. [Crossref] [PubMed]
- Yadav N, Rao S, Saini J, Prasad C, Mahadevan A, Sadashiva N. Papillary glioneuronal tumors: a radiopathologic correlation. Eur J Radiol 2017;97:44-52. [Crossref] [PubMed]
- Hsu C, Kwan G, Lau Q, Bhuta S. Rosette-forming glioneuronal tumour: imaging features, histopathological correlation and a comprehensive review of literature. Br J Neurosurg 2012;26:668-73. [Crossref] [PubMed]
- Harwood-Nash DC, Reilly BJ. Calcification of the basal ganglia following radiation therapy. Am J Roentgenol Radium Ther Nucl Med 1970;108:392-5. [Crossref] [PubMed]
- Suzuki S, Nishio S, Takata K, Morioka T, Fukui M. Radiation-induced brain calcification: paradoxical high signal intensity in T1-weighted MR images. Acta Neurochir (Wien) 2000;142:801-4. [Crossref] [PubMed]
- Halpin S, Kingsley D. Disappearance of cerebral calcification as a sign of tumor growth. AJNR Am J Neuroradiol 1993;14:119-22. [PubMed]
- Schwetye KE, Kansagra AP, McEachern J, Schmidt RE, Gauvain K, Dahiya S. Unusual high-grade features in pediatric diffuse leptomeningeal glioneuronal tumor: comparison with a typical low-grade example. Hum Pathol 2017;70:105-12. [Crossref] [PubMed]
- Blumenthal DT, Aisenstein O, Ben-Horin I, Ben Bashat D, Artzi M, Corn BW, Kanner AA, Ram Z, Bokstein F. Calcification in high grade gliomas treated with bevacizumab. J Neurooncol 2015;123:283-8. [Crossref] [PubMed]
- Pellerino A, Rudà R, Bertero L, Magistrello M, Franchino F, Cassoni P, Pasqualetti F, Pinessi L, Giangaspero F, Soffietti R. Successful use of bevacizumab in an adult primary diffuse leptomeningeal glioneuronal tumor. J Neurosurg Sci 2018;62:229-32. [Crossref] [PubMed]