Evaluation of liver tumor identification rate of volumetric-interpolated breath-hold images using the compressed sensing method and qualitative evaluation of tumor contrast effect via visual evaluation
Introduction
Among the noninvasive imaging modalities available for the detection of liver tumors, contrast-enhanced magnetic resonance imaging (MRI) using liver-specific contrast agents has superior diagnostic performance as compared with other imaging modalities (1). In addition, low temporal resolution, which has been considered a disadvantage of MRI in the past, is gradually being solved by recent and ever-evolving imaging technique (2,3).
We have already reported the usefulness of the using compressed sensing (CS)-volumetric-interpolated breath-hold examination (VIBE) (CS-VIBE) with improved temporal resolution as compared with computed tomography (CT) for the evaluation of blood flow and circulation in the arterial phase (4). Specifically, we have reported that CS-VIBE can be used to evaluate wash-in/wash-out in the arterial phase, which has been difficult to do using MRI, and that it allows to generate time intensity curve (TIC) very similar to CT. In addition, as compared with the conventional VIBE sequence, CS-VIBE was shown to significantly improve the image contrast ratio of the liver, aorta, portal vein, and pancreas to the erector spinae muscle and its usefulness in MRI contrast studies has been demonstrated. However, the k-space sampling used in CS-VIBE differs significantly from conventional acquisition in its coherence and is combined with a non-linear, sparsity-enforcing reconstruction (5). Therefore, for clinical use, it is not sufficient to simply improve the temporal resolution and contrast ratio. In clinical practice, the detection and evaluation of tumors in abdominal contrast studies, such as liver, are very important (6,7). If we can show improved or equivalent performance in tumor detection as well as improved temporal resolution and image contrast, we can expect our CS-VIBE method to be used clinically.
Several reports have detected liver tumors on MRI using CS. However, most of these used radial sampling or parallel imaging under free-breathing conditions (3,8). This is possibly the first study to evaluate tumor enhancement using the view-sharing sequence with only the CS method in a prospective study using breath-hold. In addition, this study used the body-60ch coil, which is a rare type of abdominal coil with very high sensitivity. Therefore, evaluation using high-spatial-resolution imaging is possible, which is a particularly novel finding. The purpose of this study was to evaluate the possible clinical use of CS-VIBE in patients with liver tumors by assessing tumor contrast enhancement effect by radiologists. We present the following article in accordance with the STARD reporting checklist (available at https://qims.amegroups.com/article/view/10.21037/qims-21-850/rc).
Methods
Image acquisition and subjects
The study was conducted in accordance with the Declaration of Helsinki (as revised in 2013). The Review Board at Tokyo Medical University Hospital approved this prospective study (registration No. SH4087), and all patients provided written informed consent. Study participants consisted of a consecutive series of all patients suspected of a liver disease who had performed liver MRI, including contrast-enhanced dynamic imaging between April 2015 and May 2017. To ensure inclusion of a sufficient number of cases at the time of planning, the duration of this study was 2 years (until the end of April 2017). Twenty-five patients were excluded because of technical failure. Thus, a total of 202 patients were enrolled (116 men and 86 women; mean age, 65.8 years; range, 26–91 years). Among the patients, 118 were excluded because no neoplastic lesions of the liver were detected on clinical imaging. Among the remaining 84 patients, 22 lesions were pathologically confirmed neoplastic lesions in the liver, and 62 lesions were confirmed by imaging and clinical observation. The etiologies of the patients in this study were metastatic (include suspected metastatic tumor) (n=27), hepatocellular carcinoma (include suspected hepatocellular carcinoma) (n=37), and benign (n=20) (as shown in Figure 1 and Table 1). Owing to the fact that the purpose of the present study was to evaluate the effect of contrast enhancement, patients with no confirmed liver tumor or no contrast enhancement effect were excluded from the evaluation. The primary sites of metastatic tumors were 13 from colorectal cancer, 11 from pancreatic cancer, and 3 from other sources. We used gadolinium-ethoxybenzyl-diethylenetriamine penta-acetic acid (Gd-EOB-DTPA) as the contrast agent. We administered Gd-EOB-DTPA as a bolus injection at 0.1 mL/kg and 2 mL/s through the vein of upper limb, followed by a 50-mL saline administered at the same rate. Dynamic multiphase contrast-enhanced MRI under breath-hold was performed for intraindividual comparisons. All patients performed MRI on a 3T system (MAGNETOM Skyra, Siemens Healthcare, Erlangen, Germany). Then we used a 60-channel body array coil. We performed a spoiled three-dimensional gradient echo sequence with CS (prototype sequence provided by Siemens Healthcare; repetition time, 3.28 ms; echo time, 1.3 ms; flip angle, 10°; field of view, 400 mm; matrix, 235×448; voxel size, 0.45×0.45×2.0 mm; thickness, 2.0 mm; acceleration factor of CS, 22; temporal resolution for one phase, 4 s and breath-hold time, 20 s). With regard to the of the imaging, we started signal acquisition after confirming that the contrast agent had returned to the left ventricle and after breath holding. In a patient weighing 60 kg, the duration time of the contrast agent injection was 28 s. The average of scan time was 20±2 s after injection of contrast agent. However, the time needed to provide the breath holding instruction to the patient (about 8 s) and to acquire data for sensitivity correction (about 3 s) were required before actual data acquisition. Thus, in the first phase of both sequences, the time required for filling the center (low frequency) of the k-space that determines the image contrast was more than 30 s after the injection. Dynamic scans in the CS-VIBE were performed five scan at 4 s/scan.
Table 1
Patient characteristics | Data |
---|---|
Neoplastic lesions of the liver | 84 |
Median (range) age, years | 70 [26–91] |
Average age, years | 66.1 |
Women | 32 (38.1%) |
Patients with hypervascularized tumors | 26 (30.9%) |
Median (range) age, years | 65 [26–70] |
Average age, years | 61.1 |
Women | 7 (26.9%) |
Metastatic tumor | 27 (32.1%) |
Hepatocellular carcinoma | 37 (44.0%) |
Benign | 20 (23.8%) |
Image analysis
The images in CS-VIBE were anonymized and assigned random patient names. Three radiologists (31, 12, and 10 years of experience in abdominal imaging, respectively) assessed the image quality. These radiologists were blinded to the imaging sequence and patient identity and independently evaluated the images. In the imaging diagnosis for liver tumor evaluation, the degree of portal vein staining in the arterial phase is considered very important for diagnosis (9). Thus, the gradual contrast enhancement effect of the portal vein was first evaluated on a four-point scale to evaluate whether a decent arterial phase could be obtained. The subjective criteria details for image quality were as follows:
- Nonenhancement or faint enhancement of the portal vein;
- Minimal enhancement (about one-third part) of the portal vein;
- Strong enhancement (about two-thirds part) of the portal vein;
- Homogeneous enhancement of portal vein.
Figure 2 shows reference images used to evaluate image quality on a four-point scale. For the contrast enhancement effect of the portal vein, the mean value of each phase for each reader was calculated, and the change was evaluated. Interobserver agreement was assessed using the Fleiss kappa for the evaluation of image quality between readers.

In addition, we reported that the contrast ratio in abdominal images of this sequence is different from that of the conventional sequence. In contrast studies, the detection of the tumor and the evaluation of the tumor itself by its enhancement effect are very important. In this study, we evaluated the contrast enhancement effect of the tumors on a three-point scale (1= nonenhancement; 2= minimal enhancement; 3= substantial enhancement) in 26 patients who were already known to have hypervascularized tumors as assessed by ultrasound or CT by radiologists experienced (31 and 12 years of experience in abdominal imaging, respectively). Of the 84 patients, 58 were excluded because they had no findings of hypervascularized tumors in the liver on ultrasound or CT, and no contrast studies were performed except for MRI. The mean time between CT and MRI examination was 33.1 days (range, 1–84 days). Figure 3 presents reference images used for the evaluation of image quality as assessed on a three-point scale. Furthermore, we assessed interobserver agreement using the weighted Cohen’s kappa for the evaluation of image quality between readers.
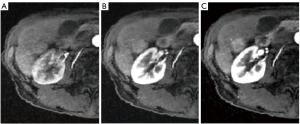
Statistical analysis
All statistical analyses software was JMP (version 14.0 for Macintosh, SAS Inc., Cary, NC, USA). We calculated the Fleiss kappa and weighted Cohen kappa to estimate the interreader agreement and interpreted the results as follows: kappa <0.20, poor agreement; 0.20≤ kappa <0.40, fair agreement; 0.40≤ kappa <0.60, moderate agreement; 0.60≤ kappa <0.80, substantial agreement; kappa >0.80, excellent agreement.
Results
For the contrast enhancement effects of the portal vein, Figure 4 shows the box plots of each phase for each reader. The mean value of the readers was 1.85 in the first phase, 2.07 in the second phase, 2.66 in the third phase, 3.05 in the fourth phase, and 3.24 in the fifth phase. Moreover, for all evaluated scores, the interreader agreement was fair to substantial (kappa 0.400–0.502; Table 2). Figure 5 shows examples of the portal vein images by the five phases. Specifically, in the signal of the portal vein, the score of second arterial phase increased gradually, and in the third arterial phase, the mean score varied from 2 to 3 (Figure 4). For tumors that already had findings of hypervascularity on ultrasound or CT, CS-VIBE identified 92.3% of tumors as hypervascular (24 of 26 tumors had findings of hypervascularity). For the contrast enhancement effect of the tumors, Table 3 presents the results of the scores for each reader and the degree of agreement. From the results, the interreader agreement was found to be fair to moderate (kappa 0.414–0.521). No significant adverse events occurred as a result of MRI scan.
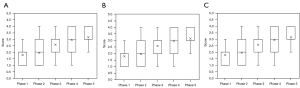
Table 2
Variables | Phase 1, mean (SD) | Phase 2, mean (SD) | Phase 3, mean (SD) | Phase 4, mean (SD) | Phase 5, mean (SD) |
---|---|---|---|---|---|
Reader 1 | 1.89 (0.993) | 2.08 (1.07) | 2.617 (1.10) | 2.99 (1.09) | 3.14 (1.02) |
Reader 2 | 1.88 (0.869) | 2.16 (0.956) | 2.785 (0.992) | 3.185 (0.957) | 3.427 (0.784) |
Reader 3 | 1.78 (0.824) | 1.96 (0.880) | 2.57 (0.947) | 2.96 (0.943) | 3.14 (0.861) |
κ (95% CI) | 0.502 (0.448–0.556) | 0.446 (0.397–0.496) | 0.475 (0.427–0.522) | 0.438 (0.388–0.488) | 0.400 (0.323–0.472) |
Mean (SD): mean and standard deviation of the tumor contrast enhancement effect scores [score of 1, nonenhancement or faint enhancement of the portal vein; score of 2, minimal enhancement (about one-third part) of the portal vein; score of 3, mostly enhancement (about two-thirds part) of the portal vein; score of 4, homogeneous enhancement of portal vein] on a 4-point scale. κ: Fleiss kappa coefficient.
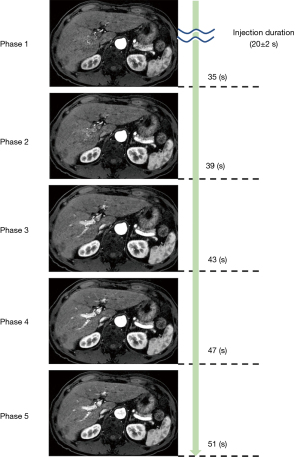
Table 3
Variables | Phase 1, mean (SD) | Phase 2, mean (SD) | Phase 3, mean (SD) | Phase 4, mean (SD) | Phase 5, mean (SD) |
---|---|---|---|---|---|
Reader 1 | 2.15 (0.61) | 2.19 (0.57) | 2.19 (0.57) | 2.19 (0.57) | 2.15 (0.56) |
Reader 2 | 2.00 (0.40) | 2.04 (0.45) | 2.04 (0.45) | 2.04 (0.45) | 2.15 (0.56) |
κ (95% CI) | 0.487 (0.194–0.780) | 0.521 (0.211–0.830) | 0.461 (0.142–0.780) | 0.461 (0.142–0.780) | 0.414 (−0.067–0.90) |
Mean (SD): mean and standard deviation of the tumor contrast enhancement effect scores (score of 1, nonenhancement; score of 2, minimal enhancement; score of 3, substantial enhancement) on a 3-point scale. κ: weighted kappa coefficient.
Discussion
In the visual assessment of the contrast enhancement effects of the portal vein, the mean score for the third or fourth arterial phase was 2 or 3, specifically 1/3–2/3 enhancement of the portal vein. This result was the same for both readers. In general, liver tumors have no enhancement or only weak dense staining in the arterial phase of only the arterial circulation, and a prior study reported that 15–20 s from the peak of the abdominal aorta is good for the tumor diagnosis (9). This phase is earlier than the peak signal of the portal phase, specifically the timing when the portal vein is faintly stained. The score was 2 or 3 for this visual evaluation, and in the arterial phase, the 3rd or 4th phase corresponded to this time phase. In this method, the required phase, as described above, is sufficiently included in the imaging time, and the images of the phases before and after the phase are also acquired. Therefore, we believe that we can obtain more information, such as dynamic changes in the enhancement characteristics of a tumor. In addition, we expect to improve the contrast ratio, which we have already reported; thus, we believe this sequence is suitable for the evaluation of tumors.
Next, we visually evaluated the tumor enhancement and determined that most of the scores were greater than 2. This was consistent with the results assessed using ultrasound or CT, because the subject was already a tumor known to be hypervascular.
This enhancement was slightly higher in phase 2 than in phase 1 of the artery, which is consistent with the results reported in a previous paper (9). However, both readers assigned the two cases a score of 1 (no contrast enhancement) for a tumor known to be hypervascular. This was probably because of the slightly higher signal in the liver parenchyma from the precontrast images and faint body motion artifacts. Consequently, we used a postprocessing sequence with CS, which we believe in itself does not reduce the tumor signal or affect detectability. However, as we have already reported, the joint reconstruction of all phases with a temporal regularization in the image domain leads to the fact that motion artifacts, such as breathing, can propagate between all phases (4). In our previous study, the effect of motion artifacts was about 45% as compared with the conventional method (4). Therefore, it is extremely important to control the factors related to motion, such as breathing. However, if this disadvantage can be controlled, the sequence provides sufficient contrast and detectability. In fact, TICs, including the arterial layer, play an important role in the classification of liver lesions (10). Because TICs are a specific feature of liver lesions, machine learning has been reported in recent years to improve diagnostic performance and to evaluate and classify malignancy (11). Among the studies on this topic, Alksas et al. suggested the possibility of Computer Assisted Diagnosis (CAD) for LI-RADS using more detailed analysis (i.e., functional markers, texture analysis, and morphological markers). Their CAD system distinguished between benign, intermediate, and malignant hepatocellular carcinoma and also allowed for the classification of subtypes, which was previously considered difficult (12). In this study, we obtained higher-contrast, multitemporal TICs in the prototype sequence, which enabled us to perform detailed analysis. Therefore, future research should use machine learning and deep learning and apply the obtained features to CAD and other applications to improve the diagnostic capability. As a result, we believe that this may facilitate the early detection of tumors.
This study had several limitations. Because this study focused only on the liver, results in other organs might not follow. In addition, this is a prototype sequence, so the results may differ when the commercial version is released. In addition, although the imaging parameters were fixed in this study, the results may not necessarily be the same if the degree of variable-density sampling, which is unique to CS, or other parameters are changed. Owing to the fact that the present study was performed using a 60ch coil for imaging, the imaging parameters may be dependent on this coil. Finally, the sample size of this study was not sufficiently large. However, we believe that we were able to evaluate the characteristics of this prototype sequence for tumors.
In conclusion, using this method, we were able to obtain multiphase images, including the phase, which is useful for evaluating liver tumors. Furthermore, the radiologist could detect the tumor as earlier. Therefore, CS-VIBE is clinically useful for EOB liver studies.
Acknowledgments
Funding: None.
Footnote
Reporting Checklist: The authors have completed the STARD reporting checklist. Available at https://qims.amegroups.com/article/view/10.21037/qims-21-850/rc
Conflicts of Interest: All authors have completed the ICMJE uniform disclosure form (available at https://qims.amegroups.com/article/view/10.21037/qims-21-850/coif). DY, YA, CM, NS, YT, SS and KS report that they were provided work in progress sequence from Siemens. KS reports grants were provided by Eisai, Bayer, GE pharmaceutical, Gerbet, FUJIFILM Toyama Chemical, Nihon Medi-physics and Daiichi-Sankyo, and personal fees were provided by Eisai, Bayer, GE pharmaceutical, Gerbet, FUJIFILM Toyama Chemical and Nihon Medi-physics. KM and DN are current employees of Siemens Healthcare. The authors have no other conflicts of interest to declare.
Ethical Statement: The authors are accountable for all aspects of the work in ensuring that questions related to the accuracy or integrity of any part of the work are appropriately investigated and resolved. The trial was conducted in accordance with the Declaration of Helsinki (as revised in 2013). The study was approved by the Institutional Review Board of Tokyo Medical University Hospital (registration No. SH4087), and informed consent was obtained from all individual participants.
Open Access Statement: This is an Open Access article distributed in accordance with the Creative Commons Attribution-NonCommercial-NoDerivs 4.0 International License (CC BY-NC-ND 4.0), which permits the non-commercial replication and distribution of the article with the strict proviso that no changes or edits are made and the original work is properly cited (including links to both the formal publication through the relevant DOI and the license). See: https://creativecommons.org/licenses/by-nc-nd/4.0/.
References
- Elsayes KM, Hooker JC, Agrons MM, Kielar AZ, Tang A, Fowler KJ, Chernyak V, Bashir MR, Kono Y, Do RK, Mitchell DG, Kamaya A, Hecht EM, Sirlin CB. 2017 Version of LI-RADS for CT and MR Imaging: An Update. Radiographics 2017;37:1994-2017. [Crossref] [PubMed]
- Hamilton J, Franson D, Seiberlich N. Recent advances in parallel imaging for MRI. Prog Nucl Magn Reson Spectrosc 2017;101:71-95. [Crossref] [PubMed]
- Yoon JK, Kim MJ, Lee S. Compressed Sensing and Parallel Imaging for Double Hepatic Arterial Phase Acquisition in Gadoxetate-Enhanced Dynamic Liver Magnetic Resonance Imaging. Invest Radiol 2019;54:374-82. [Crossref] [PubMed]
- Yoshimaru D, Araki Y, Maruyama C, Shirota N, Tajima Y, Murata K, Nickel D, Saito K. Evaluation of abdominal hemodynamics through compressed sensing accelerated functional imaging. Magn Reson Imaging 2020;73:186-91. [Crossref] [PubMed]
- Craven D, McGinley B, Kilmartin L, Glavin M, Jones E. Compressed sensing for bioelectric signals: a review. IEEE J Biomed Health Inform 2015;19:529-40. [Crossref] [PubMed]
- Lee SE, An C, Hwang SH, Choi JY, Han K, Kim MJ. Extracellular contrast agent-enhanced MRI: 15-min delayed phase may improve the diagnostic performance for hepatocellular carcinoma in patients with chronic liver disease. Eur Radiol 2018;28:1551-9. [Crossref] [PubMed]
- Wang F, Numata K, Okada M, Chuma M, Nihonmatsu H, Moriya S, Nozaki A, Ogushi K, Luo W, Ruan L, Nakano M, Otani M, Inayama Y, Maeda S. Comparison of Sonazoid contrast-enhanced ultrasound and gadolinium-ethoxybenzyl-diethylenetriamine pentaacetic acid MRI for the histological diagnosis of hepatocellular carcinoma. Quant Imaging Med Surg 2021;11:2521-40. [Crossref] [PubMed]
- Feng L, Axel L, Chandarana H, Block KT, Sodickson DK, Otazo R. XD-GRASP: Golden-angle radial MRI with reconstruction of extra motion-state dimensions using compressed sensing. Magn Reson Med 2016;75:775-88. [Crossref] [PubMed]
- Kagawa Y, Okada M, Kumano S, Katsube T, Imaoka I, Tanigawa N, Ishii K, Kudo M, Murakami T. Optimal scanning protocol of arterial dominant phase for hypervascular hepatocellular carcinoma with gadolinium-ethoxybenzyl-diethylenetriamine pentaacetic acid-enhanced MR. J Magn Reson Imaging 2011;33:864-72. [Crossref] [PubMed]
- Tsuboyama T, Jost G, Pietsch H, Tomiyama N. Effect of Gadoxetic Acid Injection Duration on Tumor Enhancement in Arterial Phase Liver MRI. Acad Radiol 2020;27:e216-23. [Crossref] [PubMed]
- Wu Y, White GM, Cornelius T, Gowdar I, Ansari MH, Supanich MP, Deng J. Deep learning LI-RADS grading system based on contrast enhanced multiphase MRI for differentiation between LR-3 and LR-4/LR-5 liver tumors. Ann Transl Med 2020;8:701. [Crossref] [PubMed]
- Alksas A, Shehata M, Saleh GA, Shaffie A, Soliman A, Ghazal M, Khelifi A, Khalifeh HA, Razek AA, Giridharan GA, El-Baz A. A novel computer-aided diagnostic system for accurate detection and grading of liver tumors. Sci Rep 2021;11:13148. [Crossref] [PubMed]