Relationship of body mass index and abdominal fat with radiation dose received during preoperative liver CT in potential living liver donors: a cross-sectional study
Introduction
Liver transplantation (LT) is the most effective treatment for end-stage liver disease (1), and living donor LT (LDLT) is frequently performed, due to a shortage of deceased organ donors (2). The main clinical concern with LDLT is the risk to living donors who are generally healthy prior to the LT (1). To reduce the risks of LDLT and ensure donor safety, careful preoperative evaluation of hepatic parenchyma, anatomical variations in the hepatic vasculature, and precise estimation of hepatic volume are crucial for appropriate donor selection and surgical planning (3,4). Contrast-enhanced computed tomography (CT) is currently the most widely-used imaging modality for preoperative evaluation in LDLT because of its superior spatial resolution, easy accessibility, and short acquisition time (3,5,6). However, radiation exposure is a major concern with CT, especially for younger living donors, as cumulative radiation doses throughout a person’s lifetime may increase their potential risk of cancer development (7).
Various strategies have been proposed to reduce the radiation dose to patients, in accordance with the principle of ALARA, which means to keep the radiation dose “as low as reasonably achievable”. Some of these strategies include the following: tube current modulation, lower tube voltage protocols, automatic exposure control (AEC), and iterative reconstruction (8-11). Of these strategies, AEC, which aims to automatically adjusts the tube current to the patient size, is widely used with multidetector CT systems, providing a substantial reduction in radiation dose while maintaining adequate image quality (9,12). Meanwhile, it is already well-known that patients with a larger body habitus receive a significantly higher radiation dose during abdominopelvic CT scans when an AEC system is used (13). Previous studies have investigated the effects of patients’ body weight, cross-sectional area, and anteroposterior diameter on doses of ionizing radiation during abdominopelvic CT scans using AEC (14-17). However, limited studies have evaluated the relationship between the abdominal fat and radiation dose during abdominopelvic CT scans (13,18). To the best of our knowledge, no previous studies have evaluated the relationship of body mass index (BMI) and abdominal fat with the radiation dose during liver CT scans, particularly when focused on potential living donors, who are young and healthy.
Therefore, the purpose of the present study was to determine the relationship of BMI and abdominal fat with the effective dose received during liver CT scans as part of a pre-donation work-up in potential living liver donors. We present the following article in accordance with the STROBE reporting checklist (available at https://qims.amegroups.com/article/view/10.21037/qims-21-977/rc).
Methods
The study was conducted in accordance with the Declaration of Helsinki (as revised in 2013). The present study was approved by the Institutional Review Board of Asan Medical Center. The requirement for written informed consent was waived due to the retrospective nature of the study.
Study population
We retrospectively searched the database from Asan Medical Center to identify living liver donor candidates who had undergone preoperative CT imaging of the liver between January 2017 and December 2018. The potential donors underwent CT imaging of the liver as part of a pre-donation work-up in order to evaluate for hepatic parenchyma and anatomical variations in the hepatic vasculature, and to estimate the liver volume. Subjects who had fully visible abdominal fat at the third lumbar vertebra level on CT imaging were included in this study. Donor candidates who had undergone CT imaging of the liver at outside hospital were excluded due to inconsistency in CT scan parameters.
CT acquisition
Preoperative CT imaging was performed using 64- or 128-multidetector scanners (Somatom series, Siemens, Erlangen, Germany). Unenhanced CT scans were obtained for the quantitative assessment of hepatic steatosis, followed by biphasic (hepatic arterial and portal venous phases) contrast-enhanced CT scans for the anatomical mapping of the hepatic vasculature and CT volumetry. The scan parameters are listed in Table 1. The tube current was controlled using the CARE Dose 4D software package (Siemens), an AEC system which modulates the tube current within different anatomic regions (z-axis) as well as within the section (angular). Based on a single anteroposterior or lateral topogram, CARE Dose 4D determined the adequate tube current level (mAs) for each section of the subject and modulated the tube current to maintain similar image quality throughout the scan length. Thus, the scan length and dose were specific to each subject. The software package then generated the dose length product (DLP) (mGy.cm) and total mAs for each examination, which was also specific to each subject. The effective dose (mSv) was calculated by multiplying the DLP with a conversion factor (k), which was defined as the region-specific normalized effective dose (19).
Table 1
Parameters | Protocols |
---|---|
kV/effective mAs/rotation time, s | 100/200/0.5 |
Detector collimation, mm | 0.6 |
Pitch | 1 |
Scan direction | Craniocaudal |
Scan range | Non-contrast and hepatic arterial phase—from diaphragm to liver lower margin |
Portal venous phase—from diaphragm to symphysis pubis | |
Intravenous contrast | 150 mL of iopromide (Ultravist 370, Bayer Schering Pharma, Berlin, Germany) |
Body composition parameters
A single axial CT image at the level of the inferior endplate of the third lumbar vertebra was selected from each subject for processing. Abdominal CT image analyses were performed with a fully convolutional, network-based, automatic segmentation technique using a deep-learning system (20), and body composition was assessed using an artificial intelligence software (AID-UTM, iAID Inc., Seoul, Republic of Korea) (20). CT images were automatically segmented to generate boundaries and measure the abdominal fat. The visceral fat area (VFA, cm2) and subcutaneous fat area (SFA, cm2) were demarcated using fat tissue thresholds (−190 to −30 Hounsfield units). The total fat area (TFA, cm2) was calculated by adding VFA and SFA.
Data collection
Data on age, sex, and anthropometric measurements (body weight and height) were collected for each subject. BMI was calculated as the body weight (kg) divided by the square of the height (m2). The BMI status of subjects was determined using ethnicity-specific cutoff values, as follows: BMI <23 kg/m2 for lean, 23–24.9 kg/m2 for overweight, and ≥25 kg/m2 for obese.
Statistical analyses
Descriptive data were expressed as mean ± standard deviation. The Shapiro-Wilk test was performed to check normality of distribution. Correlations between the effective dose and other variables were evaluated using Pearson’s correlation coefficient. Statistical significance was set at P<0.05, and statistical analyses were performed using SPSS (version 23.0; IBM, Armonk, NY, USA).
Results
Characteristics of the study population
A total of 695 potential living liver donors (mean age, 30.5±9.7 years; 445 men and 250 women) were included for analysis. The characteristics of the study population are summarized in Table 2. The mean BMI was 23.6±3.3 kg/m2, and in terms of abdominal fat parameters, the mean VFA, SFA, and TFA were 66.2±45.8, 152.5±80.6, and 218.7±110.0 cm2, respectively. The mean DLP was 623.4±218.0 mGy∙cm, and the mean effective dose was 9.4±3.3 mSv.
Table 2
Variables | n=695 |
---|---|
Age, years | 30.5±9.7 |
Sex, men:women | 445:250 |
Body mass index, kg/m2 | 23.6±3.3 |
Visceral fat area, cm2 | 66.2±45.8 |
Subcutaneous fat area, cm2 | 152.5±80.6 |
Total fat area, cm2 | 218.7±110.0 |
Dose length product, mGy∙cm | 623.4±218.0 |
Effective dose, mSv | 9.4±3.3 |
Correlation between effective dose and other variables
The effective dose had a strong positive correlation with BMI (r=0.715; P<0.001), SFA (r=0.770; P<0.001), and TFA (r=0.792; P<0.001), and a moderate positive correlation with VFA (r=0.545; P<0.001) (Table 3).
Table 3
Quartile (Effective dose, mSv) | I (<7.15) | II (7.15–8.75) | III (8.75–10.82) | IV (>10.82) | Correlation coefficient (r) with effective dose | P value |
---|---|---|---|---|---|---|
Body mass index, kg/m2 | 21.0±2.1 | 22.8±2.1 | 23.8±2.5 | 26.6±3.7 | 0.715 | <0.001 |
Visceral fat area, cm2 | 36.3±25.2 | 55.3±36.4 | 70.5±44.3 | 102.5±46.4 | 0.545 | <0.001 |
Subcutaneous fat area, cm2 | 88.1±45.0 | 129.5±46.9 | 159.9±54.6 | 232.2±88.2 | 0.770 | <0.001 |
Total fat area, cm2 | 124.4±60.7 | 184.7±65.6 | 230.4±75.3 | 334.7±105.8 | 0.792 | <0.001 |
Relationship of BMI and abdominal fat on the effective dose
The mean effective dose for a BMI considered to be lean was 7.7±1.9 mSv, 9.2±2.0 mSv for overweight, and 12.3±3.9 mSv for obese, with statistically significant differences (P<0.001). The mean effective dose for a TFA <200 cm2 was 7.5±1.8 mSv, for a TFA 200–400 cm2 was 10.3±2.5 mSv, and for a TFA >400 cm2 was 17.3±4.2 mSv, with statistically significant differences (P<0.001). As BMI and TFA increased, so did the effective dose (Figure 1).
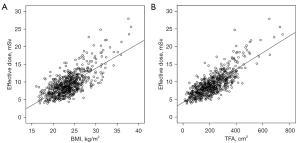
Discussion
In the present study, the effective dose was found to be positively correlated with BMI and the area of abdominal fat. An increased BMI was associated with an increased effective dose from the multidetector CT, performed with an AEC system. An increase in abdominal fat significantly increased the effective dose from the liver CT performed as part of the pre-donation work-up for potential living donors.
To improve the success and reduce the risks of LDLT, the following are needed for appropriate donor selection and surgical planning: careful preoperative assessments of hepatic parenchyma, hepatic vascular anatomy, and liver volume estimation (3,4). Currently, contrast-enhanced multidetector CT is the most widely-used imaging modality for the preoperative evaluation of potential living liver donors, due to its excellent spatial resolution, easy accessibility, and short acquisition time (3,5,6). However, considerable disadvantage of CT imaging is radiation exposure (7). In terms of medical exposure, an effective dose >10 mSv is considered to be a moderate risk for a single exposure (21). In the present study, more than two-thirds of obese subjects (BMI >25 kg/m2) had an effective dose >10 mSv. Considering that the living liver donor population is younger, and that a patient’s cumulative radiation doses over their lifetime can increase the probability of developing cancer (7), alternative imaging modalities such as magnetic resonance imaging (MRI) should be considered, especially for potential living liver donors with a higher BMI. With recent technical advances such as increased gradient strength, refined pulse sequences, and novel contrast agents, MRI has the potential to serve as an “all-in-one” imaging modality, particularly regarding the degree of hepatic steatosis and detailed evaluation of vascular and biliary anatomy in potential living liver donors (22),
In a study by McLaughlin et al. (13), the total abdominal adipose tissue was more predictive of DLP than BMI, which is consistent with the results of the present study. Our study also demonstrated that TFA was more strongly correlated with effective dose than BMI. These results suggest that the estimation of total abdominal adiposity, more than BMI, may guide to reduce the radiation dose and facilitate dose optimization protocols when using AEC. A possible process would be to acquire a representative single-slice abdominal CT section at the level of the third vertebra and obtain an automated quantification of TFA (13). We believe that TFA-based optimization protocols from single-slice CT sections may provide an individualized approach for a more aggressive dose reduction in young and healthy living liver donors.
The present study has several limitations. This was a single-center retrospective study; therefore, the results should be prospectively validated in a multicenter study. Additionally, the mean BMI of our study population was quite low because the study population was Asian. Therefore, further studies in a Western population with a substantial proportion of subjects with a higher BMI would be beneficial. In this study, DLP-based effective dose estimation was used. However, according to a recent publication (23), DLP-based effective dose estimates differ significantly from organ dose-based effective dose estimates and may not be accurate compared to organ dose-based effective dose estimates. Further studies using organ dose-based effective dose estimates may be needed. Lastly, thick layers of subcutaneous fat may shield internal organs and act to reduce effective dose partly mitigating the additional mAs required for imaging. Accordingly, the results reported might be overestimates of the true effect of obesity on radiation dose.
In conclusion, the effective dose had a significant positive correlation with both BMI and abdominal fat during preoperative CT; therefore, increased BMI and abdominal fat significantly increased the radiation dose received during liver CT in potential living liver donors.
Acknowledgments
Funding: This research was supported by the Basic Science Research Program through the National Research Foundation (NRF) of Korea, funded by the Ministry of Science, ICT, and Future Planning (No. 2017R1E1A1A03070961).
Footnote
Reporting Checklist: The authors have completed the STROBE reporting checklist. Available at https://qims.amegroups.com/article/view/10.21037/qims-21-977/rc
Conflicts of Interest: All authors have completed the ICMJE uniform disclosure form (available at https://qims.amegroups.com/article/view/10.21037/qims-21-977/coif). The authors have no conflicts of interest to declare.
Ethical Statement: The authors are accountable for all aspects of the work in ensuring that questions related to the accuracy or integrity of any part of the work are appropriately investigated and resolved. The study was conducted in accordance with the Declaration of Helsinki (as revised in 2013). The present study was approved by the Institutional Review Board of Asan Medical Center. The requirement for written informed consent was waived due to the retrospective nature of the study.
Open Access Statement: This is an Open Access article distributed in accordance with the Creative Commons Attribution-NonCommercial-NoDerivs 4.0 International License (CC BY-NC-ND 4.0), which permits the non-commercial replication and distribution of the article with the strict proviso that no changes or edits are made and the original work is properly cited (including links to both the formal publication through the relevant DOI and the license). See: https://creativecommons.org/licenses/by-nc-nd/4.0/.
References
- EASL Clinical Practice Guidelines. Liver transplantation. J Hepatol 2016;64:433-85. [Crossref] [PubMed]
- Lee SG. A complete treatment of adult living donor liver transplantation: a review of surgical technique and current challenges to expand indication of patients. Am J Transplant 2015;15:17-38. [Crossref] [PubMed]
- Schroeder T, Malagó M, Debatin JF, Goyen M, Nadalin S, Ruehm SG. "All-in-one" imaging protocols for the evaluation of potential living liver donors: comparison of magnetic resonance imaging and multidetector computed tomography. Liver Transpl 2005;11:776-87. [Crossref] [PubMed]
- Schroeder T, Radtke A, Kuehl H, Debatin JF, Malagó M, Ruehm SG. Evaluation of living liver donors with an all-inclusive 3D multi-detector row CT protocol. Radiology 2006;238:900-10. [Crossref] [PubMed]
- Lee MW, Lee JM, Lee JY, Kim SH, Park EA, Han JK, Kim YJ, Shin KS, Suh KS, Choi BI. Preoperative evaluation of the hepatic vascular anatomy in living liver donors: comparison of CT angiography and MR angiography. J Magn Reson Imaging 2006;24:1081-7. [Crossref] [PubMed]
- Kwon HJ, Kim KW, Jang JK, Lee J, Song GW, Lee SG. Reproducibility and reliability of computed tomography volumetry in estimation of the right-lobe graft weight in adult-to-adult living donor liver transplantation: Cantlie's line vs portal vein territorialization. J Hepatobiliary Pancreat Sci 2020;27:541-7. [Crossref] [PubMed]
- Mathews JD, Forsythe AV, Brady Z, Butler MW, Goergen SK, Byrnes GB, Giles GG, Wallace AB, Anderson PR, Guiver TA, McGale P, Cain TM, Dowty JG, Bickerstaffe AC, Darby SC. Cancer risk in 680,000 people exposed to computed tomography scans in childhood or adolescence: data linkage study of 11 million Australians. BMJ 2013;346:f2360. [Crossref] [PubMed]
- McCollough CH, Primak AN, Braun N, Kofler J, Yu L, Christner J. Strategies for reducing radiation dose in CT. Radiol Clin North Am 2009;47:27-40. [Crossref] [PubMed]
- Yu L, Liu X, Leng S, Kofler JM, Ramirez-Giraldo JC, Qu M, Christner J, Fletcher JG, McCollough CH. Radiation dose reduction in computed tomography: techniques and future perspective. Imaging Med 2009;1:65-84. [Crossref] [PubMed]
- Kordolaimi SD, Argentos S, Pantos I, Kelekis NL, Efstathopoulos EP. A new era in computed tomographic dose optimization: the impact of iterative reconstruction on image quality and radiation dose. J Comput Assist Tomogr 2013;37:924-31. [Crossref] [PubMed]
- Huber S, Cornfeld D, Emre SH, Israel GM. Comparison of multidetector computed tomography angiography and cholangiography performed at 80 and 120 kVp in live liver donors. J Comput Assist Tomogr 2013;37:443-9. [Crossref] [PubMed]
- Kalra MK, Naz N, Rizzo SM, Blake MA. Computed tomography radiation dose optimization: scanning protocols and clinical applications of automatic exposure control. Curr Probl Diagn Radiol 2005;34:171-81. [Crossref] [PubMed]
- McLaughlin PD, Chawke L, Twomey M, Murphy KP, O'Neill SB, McWilliams SR, James K, Kavanagh RG, Sullivan C, Chan FE, Moore N, O'Connor OJ, Eustace JA, Maher MM. Body composition determinants of radiation dose during abdominopelvic CT. Insights Imaging 2018;9:9-16. [Crossref] [PubMed]
- Israel GM, Cicchiello L, Brink J, Huda W. Patient size and radiation exposure in thoracic, pelvic, and abdominal CT examinations performed with automatic exposure control. AJR Am J Roentgenol 2010;195:1342-6. [Crossref] [PubMed]
- Schindera ST, Nelson RC, Toth TL, Nguyen GT, Toncheva GI, DeLong DM, Yoshizumi TT. Effect of patient size on radiation dose for abdominal MDCT with automatic tube current modulation: phantom study. AJR Am J Roentgenol 2008;190:W100-5. [Crossref] [PubMed]
- Meeson S, Alvey CM, Golding SJ. The in vivo relationship between cross-sectional area and CT dose index in abdominal multidetector CT with automatic exposure control. J Radiol Prot 2010;30:139-47. [Crossref] [PubMed]
- Zarb F, Rainford L, McEntee MF. AP diameter shows the strongest correlation with CTDI and DLP in abdominal and chest CT. Radiat Prot Dosimetry 2010;140:266-73. [Crossref] [PubMed]
- Chan VO, McDermott S, Buckley O, Allen S, Casey M, O'Laoide R, Torreggiani WC. The relationship of body mass index and abdominal fat on the radiation dose received during routine computed tomographic imaging of the abdomen and pelvis. Can Assoc Radiol J 2012;63:260-6. [Crossref] [PubMed]
- Huda W, Magill D, He W. CT effective dose per dose length product using ICRP 103 weighting factors. Med Phys 2011;38:1261-5. [Crossref] [PubMed]
- Park HJ, Shin Y, Park J, Kim H, Lee IS, Seo DW, Huh J, Lee TY, Park T, Lee J, Kim KW. Development and Validation of a Deep Learning System for Segmentation of Abdominal Muscle and Fat on Computed Tomography. Korean J Radiol 2020;21:88-100. [Crossref] [PubMed]
- The 2007 Recommendations of the International Commission on Radiological Protection. ICRP publication 103. Ann ICRP 2007;37:1-332. [PubMed]
- Kim B, Kim SY, Kim KW, Jang HY, Jang JK, Song GW, Lee SG. MRI in donor candidates for living donor liver transplant: Technical and practical considerations. J Magn Reson Imaging 2018;48:1453-67. [Crossref] [PubMed]
- Fu W, Ria F, Segars WP, Choudhury KR, Wilson JM, Kapadia AJ, Samei E. Patient-Informed Organ Dose Estimation in Clinical CT: Implementation and Effective Dose Assessment in 1048 Clinical Patients. AJR Am J Roentgenol 2021;216:824-34. [Crossref] [PubMed]