The feasibility of 18F-FDG gated positron emission tomography (PET) for left ventricular dyssynchrony assessment in comparison with 99mTc-MIBI gated single-photon emission computed tomography (SPECT) among patients with prior myocardial infarction
Introduction
Left ventricular (LV) asynchrony is associated with severe heart failure, and cardiac resynchronization therapy (CRT) has proven to be an effective treatment (1-4). However, according to current guidelines, nearly 30% of patients with heart failure have not received good treatment results for CRT (5). Research has shown that LV mechanical dyssynchrony, LV motion patterns, amount of viable myocardium and scar burden are the main factors affecting a patient’s response to CRT (6). By evaluating the mechanical dyssynchrony of the left ventricle, it may be helpful to determine which patients will benefit from CRT. Therefore, we selected myocardial infarction (MI) patients with somewhat larger areas of total perfusion defects (TPDs) and scars as the research objects, which is more representative.
Phase analysis of 99mTc-MIBI gated single-photon emission computed tomography (GSPECT) can evaluate LV dyssynchrony, which has been regarded as a standard reference (7). Compared with ungated acquisition, GSPECT does not increase the risk of additional radiation exposure. Compared with GSPECT, 18F-FDG gated positron emission tomography (GPET) has more advantages. For example, GPET is a routinely performed gated acquisition and has a higher spatial resolution. In addition, the myocardium with impaired but viable blood perfusion often shows reduced uptake of 99mTc-MIBI and preserved uptake of 18F-FDG. Whether GPET phase analysis is feasible in evaluating LV dyssynchrony depends on the accuracy of its quantitative determination of histogram bandwidth (BW) and phase standard deviation (SD) values. If PET/CT assessment of LV desynchronization is feasible, then a comprehensive assessment of myocardial vitality, infarct location, and LV dyssynchrony in patients with MI in one scan before CRT without additional acquisition will be more effective and beneficial for patients. Current evidence on the role of LV mechanical dyssynchrony evaluation is mainly available for GSPECT, but 18F-FDG GPET is not often used (8). To date, there is a lack of prospective head-to-head studies to further verify the value of GPET phase analysis on LV dyssynchrony assessment and whether it can be used as a better option. Little information is available on the interchangeability between GSPECT- and GPET-derived LV dyssynchrony.
The aim of this study was to evaluate the feasibility of GPET phase analysis for clinical utilization among MI patients. Furthermore, we evaluated the impacts of viable myocardium, TPDs and scarring on the accuracy of LV mechanical dyssynchrony measured by GPET and used GSPECT phase analysis as the reference standard. We present the following article in accordance with the STARD reporting checklist (available at https://qims.amegroups.com/article/view/10.21037/qims-21-822/rc).
Methods
Study population
We prospectively studied 196 consecutive patients with prior MI who underwent GSPECT and GPET viable myocardial examination at Fu Wai Hospital from July 2016 to October 2017. The 2-day protocol was used to assess myocardial viability. That is, 99mTc-MIBI resting myocardial perfusion imaging was performed on the first day, and 18F-FDG myocardial metabolism imaging was performed on the second day. According to the diagnostic criteria of the European Society of Cardiology (ESC)/American College of Cardiology Foundation (ACCF)/American Heart Association (AHA)/World Heart Federation (WHF) expert consensus, the diagnosis of prior MI was confirmed by increased levels of biomarkers of myocardial necrosis, electrocardiographic (ECG) changes, pathological examination and imaging (9). After excluding patients with left bundle branch block, severe arrhythmia (ventricular tachycardia, or ventricular fibrillation), pregnancy, low GSPECT or GPET imaging quality and inaccurate detection of LV contour, 146 patients were included in this study. Before the start of the study, the first author evaluated all subjects and screened their research eligibility. The study was conducted in accordance with the Declaration of Helsinki (as revised in 2013). The study was approved by the ethics committee of Fu Wai Hospital and Cardiovascular Institute. Informed consent was obtained from all patients.
Resting GSPECT
The resting GSPECT image began approximately 60–90 min after intravenous administration of 925 MBq 99mTc-sestamibi. The imaging acquisition was performed with a dual-head SPECT system (e.cam, Siemens Medical Systems), which is carried out in a zoom factor of 1.45, a 128×128 matrix and a total of 32 views at 30 s per view. With a 20% window centered, the cardiac cycle was divided into eight equal fixed intervals over the 140 keV photopeak, and the Butterworth-filtered back-projection method (10) (order 5; cutoff frequency 0.40) was used to reconstruct the cardiac gated transaxial images. GSPECT images were displayed as short-axis slices, vertical long-axis slices and horizontal long-axis slices.
GPET
Under fasting conditions, the patients received a glucose load. After 40 min, regular short-acting insulin was injected intravenously according to their serum glucose level. After the serum glucose level reached the target, 148 MBq 18F-FDG (Chinese Atomic Energy Institute, Beijing, China) was administered intravenously. Imaging acquisition was performed for 10 min after 1 hour. The 18F-FDG myocardial images were obtained by a high spatial resolution PET scanner (Truepoint Biography 64, Siemens Healthcare, Knoxville, TN, USA). With a zoom factor of 2.0, the cardiac cycle was divided into 8 equal intervals and formatted into a 128×128 matrix.
Analysis of GSPECT and GPET images
After gated image acquisition was completed, the gated and nongated datasets of SPECT and PET were transferred to the Siemens e.soft workstation. Quantitative gated SPECT (QGS) (version 3.1, Cedars-Sinai Medical Center, Los Angeles, CA, USA) algorithms were used to analyze SPECT and PET images with the same functional parameters. LV parameters, such as BW, SD, mismatch, scar scores and TPD, were analyzed by QGS and quantitative perfusion SPECT (QPS) (version 3.1, Cedars-Sinai Medical Center, Los Angeles, CA, USA). For patients with a large TPD and inaccurate delineation of the endocardium and epicardium, both PET and SPECT images needed to be corrected manually. The manual correction rate was 8.2%. All data analyses were performed by a senior nuclear medicine doctor who was blinded to the patient data.
Statistical analysis
SPSS v22.0 software (SPSS Inc., Chicago, IL, USA) was used for statistical analyses. Continuous data are expressed as the mean ± SD. For nonnormal distributions, disagreements were analyzed by the Wilcoxon nonparametric test (11), and the strength of agreement was tested using Spearman correlation. The degree of agreement between GPET and GSPECT was evaluated by Bland-Altman analysis (12,13). The agreement limits were evaluated by the mean difference ±1.96 SD of differences. Factors (such as mismatch, scarring and TPD) related to the difference in LV dyssynchrony parameters (BW and SD) between GPET and GSPECT were assessed by linear regression analysis. Only variables with statistical significance in univariate analysis were further included in multivariate analysis. A P value of less than 0.05 was considered statistically significant.
Results
Patient characteristics and phase analysis
The clinical characteristics of the patients are shown in Table 1. One hundred and thirty-one men and 15 women were included. All patients had a history of prior MI. The mean values of BW and SD from GPET were 154° and 46°, respectively, and those from GSPECT were 133° and 36°, respectively.
Table 1
Characteristic | Values |
---|---|
Male sex | 131 [90] |
Age (years) | 58±11 |
Body mass index (kg/m2) | 25.1±3.4 |
Diabetes | 56 [38] |
Hypertension | 91 [62] |
Hyperlipidemia | 115 [79] |
PCI or CABG | 65 [45] |
Coronary angiography | n=96 |
Three-vessel disease | 57 [59] |
Two-vessel disease | 25 [26] |
One-vessel disease | 14 [15] |
New York Heart Association class III–IV | 44 [30] |
TPD (%) | 36±15 |
Mismatch (%) | 17±12 |
Scar (%) | 16±11 |
EF (%, echo) | 39±10 |
Data are number (with percentage in parentheses) or mean ± SD. PCI, percutaneous coronary intervention; CABG, coronary artery bypass grafting; TPD, total perfusion defect; EF, ejection fraction; SD, standard deviation.
With regard to BW and SD, the correlation between GPET and GSPECT was moderate (r=0.554 and 0.537, respectively; both P<0.001) (Figure 1A,1B). Through Bland-Altman analysis, GPET overestimated both BW and SD (20.5° and 9.5°, respectively). The overestimation of GPET had an increasing trend with increasing magnitudes of BW and SD (Figure 1C,1D) compared to GSPECT.
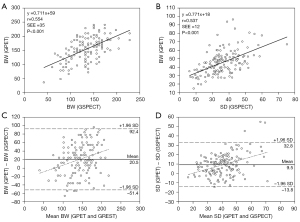
Influence of the correlation factor on the discrepancies between GPET and GSPECT
According to the TPD scores, patients were divided into two groups. A score ≤20% was Group 1, with 22 (15%) patients, and >20% was Group 2, with 124 (85%) patients. Table 2 summarizes the mean values of BW and SD for these patients. The correlation between GPET and GSPECT was moderate with respect to BW and SD (r=0.445–0.561) between the two groups. In comparison with GSPECT, the values of BW and SD were overestimated by GPET in the two groups. P<0.001 when the TPD scores were greater than 20%, and P<0.05 when the TPD scores were less than or equal to 20%.
Table 2
Groups | N | Parameter | GPET | GSPECT | R | P value |
---|---|---|---|---|---|---|
Group 1 | 22 | BW | 142 | 112 | 0.561 | 0.007 |
SD | 42 | 30 | 0.445 | 0.038 | ||
Group 2 | 124 | BW | 156 | 137 | 0.545 | <0.001 |
SD | 46 | 37 | 0.536 | <0.001 |
TPD scores ≤20%, Group 1; TPD scores >20%, Group 2. P value, between GSPECT and GPET; R, intraclass correlation coefficient between GSPECT and GPET. BW, histogram bandwidth; SD, standard deviation; GPET, 18F-FDG gated positron emission tomography; GSPECT, 99mTc-MIBI gated single-photon emission computed tomography; TPD, total perfusion defect.
In addition, according to the mismatch scores, the patients were divided into three groups. A score <10% was assigned to Group 1, which had 57 (39%) patients. A score of 10–19% was assigned to Group 2, which had 49 patients (34%). A score ≥20% was assigned to Group 3, which had 40 (27%) patients. Table 3 summarizes the mean values of BW and SD for these patients. According to the QGS results, the correlation between GPET and GSPECT was moderate for BW and SD (r=0.518–0.612). Compared with GSPECT, GPET overestimated the values of BW and SD in the three groups (P<0.001).
Table 3
Groups | N | Parameter | GPET | GSPECT | R | P value |
---|---|---|---|---|---|---|
Group 1 | 57 | BW | 150 | 127 | 0.537 | <0.001 |
SD | 46 | 34 | 0.519 | <0.001 | ||
Group 2 | 49 | BW | 154 | 131 | 0.518 | <0.001 |
SD | 44 | 35 | 0.532 | <0.001 | ||
Group 3 | 40 | BW | 160 | 146 | 0.612 | <0.001 |
SD | 48 | 40 | 0.558 | <0.001 |
Mismatch scores <10%, Group 1; 10%≤ mismatch scores <20%, Group 2; mismatch scores ≥20%, Group 3. P value, between GSPECT and GPET; R, intraclass correlation coefficient between GSPECT and GPET. BW, histogram bandwidth; SD, standard deviation; GPET, 18F-FDG gated positron emission tomography; GSPECT, 99mTc-MIBI gated single-photon emission computed tomography.
Finally, according to the perfusion/metabolic match, the patients were divided into three groups. Those with perfusion/metabolic match (scar) were assigned to Group 1, which had 21 (14%) patients. Those with perfusion/metabolic partial mismatch (partially viable) were assigned to Group 2, which had 105 (72%) patients. Those with perfusion/metabolic mismatch (viable) were assigned to Group 3, which had 20 (14%) patients. Table 4 summarizes the mean values of BW and SD for these patients. The correlation between GPET and GSPECT was moderate for BW and SD (r=0.504–0.656), as indicated by QGS analysis. Compared with GSPECT, GPET overestimated the values of BW and SD in the three groups (P<0.05).
Table 4
Groups | N | Parameter | GPET | GSPECT | R | P value |
---|---|---|---|---|---|---|
Group 1 | 21 | BW | 152 | 140 | 0.643 | 0.002 |
SD | 47 | 38 | 0.589 | 0.005 | ||
Group 2 | 105 | BW | 160 | 134 | 0.526 | <0.001 |
SD | 47 | 36 | 0.531 | <0.001 | ||
Group 3 | 20 | BW | 125 | 125 | 0.656 | 0.002 |
SD | 38 | 34 | 0.504 | 0.023 |
Perfusion/metabolic match (scar), Group 1; perfusion/metabolic partial mismatch (partially viable), Group 2; perfusion/metabolic mismatch (viable), Group 3. P value, between GSPECT and GPET; R, intraclass correlation coefficient between GSPECT and GPET. BW, histogram bandwidth; SD, standard deviation; GPET, 18F-FDG gated positron emission tomography; GSPECT, 99mTc-MIBI gated single-photon emission computed tomography.
Univariate and multivariate regression analysis
Table 5 shows the corresponding factors related to BW and SD measurements. The results of univariate regression analysis demonstrated that several factors were significantly related to the discrepancy in BW and SD measurements between GPET and GSPECT. Furthermore, according to the results of multivariate regression analysis, TPD was related to the difference in BW measurement (P<0.01), and mismatch was associated with the discrepancy in SD measurement (P<0.01) between GPET and SPECT.
Table 5
Variables | Univariate analysis | Multivariate analysis | ||||
---|---|---|---|---|---|---|
Correlation coefficient | P value | Coefficient | Standard error beta | P value | ||
Variables related to the differences in BW (ΔBW) | −0.254 | 0.260 | 0.001 | |||
TPD | −0.254 | 0.001 | ||||
Mismatch | −0.160 | 0.038 | ||||
Scar | −0.179 | 0.021 | ||||
Variables related to the differences in SD (ΔSD) | −0.210 | 0.117 | 0.006 | |||
TPD | −0.202 | 0.009 | ||||
Mismatch | −0.210 | 0.006 | ||||
Scar | −0.084 | 0.281 |
BW, histogram bandwidth; SD, standard deviation; GPET, 18F-FDG gated positron emission tomography; GSPECT, 99mTc-MIBI gated single-photon emission computed tomography; TPD, total perfusion defect.
Discussion
In this study, our main findings were as follows: (I) The correlation between GPET and GSPECT was moderate for BW and SD in patients with MI. GPET overestimated both BW and SD. The mean differences in BW and SD were 21° and 10°, respectively. (II) Subgroup analysis showed that BW and SD measured by GPET were overestimated (3). Between GPET and GSPECT, TPD was correlated with the difference in BW measurement, and mismatch was related to the difference in SD measurement.
The two-day protocol of gated 99mTC-MIBI SPECT combined with 18F-FDG PET can simultaneously evaluate myocardial ischemia, viability, LV function parameters and dyssynchrony (14-18). Current evidence on the role of LV mechanical dyssynchrony evaluation is mainly available for GSPECT, and the feasibility of 18F-FDG GPET is uncertain. Compared with GSPECT, GPET has a higher temporal and spatial resolution, so it should be more accurate in theory. In addition, gated acquisition of GPET is routinely performed. Whether GPET phase analysis is feasible in evaluating LV dyssynchrony depends on the accuracy of its quantitative determination of BW and SD values.
However, GPET moderately correlated with GSPECT in assessing LV dyssynchrony in this study. The results here are generally similar to those of previous work. Wang et al. reported that GPET and GSPECT were moderately correlated in the evaluation of LV dyssynchrony in patients with coronary artery disease (CAD) (rBW =0.58 and rSD =0.60) (19). The results of Tian et al. showed that there was a moderate correlation for BW (r=0.65) and SD (r=0.63) between GSPECT and GPET in patients with ischemic cardiomyopathy (20). However, there are also some results with high correlations. For example, Shao et al. (6) found that BW and SD obtained in GPET and GSPECT showed a good correlation in pigs with MI (rBW =0.74 and rSD =0.84). The reasons for the small difference may be that the parameters of the experimental animal pigs were measured under anesthesia and the blood glucose was not strictly regulated, which could have affected the results of dyssynchrony. Ali et al. also confirmed that there was a statistically significant difference in SD and BW between resting and stress states (21). In addition, the value of phase analysis may vary depending on the software program, even when using the same software (22,23). Additionally, the phase parameter has great variability because the phase histogram is affected by many factors, such as sex, cardiovascular risk factors, including hypertension, diabetes, and kidney disease, and imaging protocol (7,24,25).
The patients were further divided into different subgroups according to TPD scores, viable myocardial range and perfusion metabolism matching degree. The results showed that GPET overestimated the values of BW and SD in the different subgroups. In an MI patient with large perfusion defects but viable myocardium, the myocardial area detected by GPET was larger than that detected by GSPECT, and GPET overestimated the BW and SD values (Figure 2). In addition, according to the results of multivariate regression analysis, TPD was related to the difference in BW measurement, and the extent of viable myocardium was related to the difference in SD measurement between GPET and GSPECT. Similarly, Wang et al. reported that the degree of myocardial perfusion defect is an independent predictor of LV systolic dyssynchrony (26). They suggested that for coronary heart disease patients with obvious LV remodeling, poor myocardial 18F-FDG uptake or severe functional impairment, gated GPET phase analysis should be carefully applied. However, although most LV mechanical dyssynchrony is currently evaluated by GSPECT, its accuracy still needs to be further studied. MI patients with viable myocardium in the myocardial perfusion defect site usually show preserved uptake of 18F-FDG. Compared with SPECT perfusion imaging, PET detects a larger area of myocardium. In this study, we selected MI patients with a larger TPD score and scar area as the research objects, which is more representative. Taken together, in patients with MI, BW and SD measured by GPET differ from those determined by GSPECT, and thus, these results should not be interchanged in individual patients. Therefore, whether GPET can be used to evaluate LV mechanical dyssynchrony in patients with MI still needs further investigation in large-scale studies.
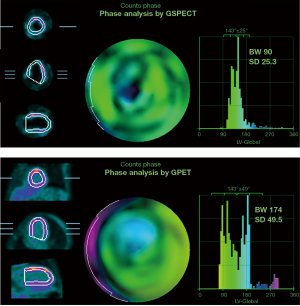
This study has several limitations. First, GSPECT does not perform attenuation correction, so it may underestimate the extent of viable myocardium, especially in the posterior and inferior walls of mild perfusion defects. Second, for patients with a large area of myocardial perfusion defects, the accuracy of the software to detect the boundaries of the outer and inner membranes will be reduced. In addition, the QGS algorithm is mainly designed for GSPECT research, and for PET imaging, a more suitable algorithm should be used for analysis to obtain more accurate results. Another limitation to consider is that the study was a single center study. Possible future work in a multicenter study to evaluate the accuracy of PET phase analysis through the results of clinical cardiac resynchronization therapy could confirm our findings. Since entropy and machine-learning approaches may help improve the estimation of CRT candidates, they can be introduced later.
Conclusions
In conclusion, there was a certain deviation between BW and SD measured by GPET and GSPECT in MI patients, and thus, they should not be interchangeable in individual patients.
Acknowledgments
Funding: This work was supported by grants from the China Postdoctoral Science Foundation (No. 2020M673079 to YY), Shenzhen Science Technology Project (No. JCYJ20210324114005015 to YY), and grant from the innovation and cultivation fund of the sixth medical center of PLA General Hospital (No. CXPY202005 to DWW).
Footnote
Reporting Checklist: The authors have completed the STARD reporting checklist. Available at https://qims.amegroups.com/article/view/10.21037/qims-21-822/rc
Conflicts of Interest: All authors have completed the ICMJE uniform disclosure form (available at https://qims.amegroups.com/article/view/10.21037/qims-21-822/coif). The authors have no conflicts of interest to declare
Ethical Statement: The authors are accountable for all aspects of the work in ensuring that questions related to the accuracy or integrity of any part of the work are appropriately investigated and resolved. The study was conducted in accordance with the Declaration of Helsinki (as revised in 2013). The study was approved by the ethics committee of Fu Wai Hospital and Cardiovascular Institute. Written informed consent was obtained from the patient.
Open Access Statement: This is an Open Access article distributed in accordance with the Creative Commons Attribution-NonCommercial-NoDerivs 4.0 International License (CC BY-NC-ND 4.0), which permits the non-commercial replication and distribution of the article with the strict proviso that no changes or edits are made and the original work is properly cited (including links to both the formal publication through the relevant DOI and the license). See: https://creativecommons.org/licenses/by-nc-nd/4.0/.
References
- Aguadé-Bruix S, Romero-Farina G, Candell-Riera J, Pizzi MN, García-Dorado D. Mechanical dyssynchrony according to validated cut-off values using gated SPECT myocardial perfusion imaging. J Nucl Cardiol 2018;25:999-1008. [Crossref] [PubMed]
- Ferrando-Castagnetto F, Ricca-Mallada R, Vidal A, Ferrando R. Effects of prolonged endocardial stimulation on left ventricular mechanical synchrony. A pilot study applying gated-SPECT phase analysis. Endocardial stimulation and dyssynchrony. Arch Cardiol Mex 2017;87:307-15. [Crossref] [PubMed]
- Hatta T, Yoda S, Hayase M, Monno K, Hori Y, Fujito H, Suzuki Y, Matsumoto N, Okumura Y. Prognostic Value of Left Ventricular Dyssynchrony Assessed with Nuclear Cardiology in Patients with Known or Suspected Stable Coronary Artery Disease with Preserved Left Ventricular Ejection Fraction. Int Heart J 2020;61:685-94. [Crossref] [PubMed]
- Luo T, Wang Z, Chen Z, Yu E, Fang C. Layer-specific strain and dyssynchrony index alteration in new-onset systemic lupus erythematosus patients without cardiac symptoms. Quant Imaging Med Surg 2021;11:1271-83. [Crossref] [PubMed]
- Abraham WT, Fisher WG, Smith AL, Delurgio DB, Leon AR, Loh E, Kocovic DZ, Packer M, Clavell AL, Hayes DL, Ellestad M, Trupp RJ, Underwood J, Pickering F, Truex C, McAtee P, Messenger JMIRACLE Study Group. Multicenter InSync Randomized Clinical Evaluation. Cardiac resynchronization in chronic heart failure. N Engl J Med 2002;346:1845-53. [Crossref] [PubMed]
- Shao X, Wang J, Tian Y, Fan S, Zhang F, Yang W, Xin W, Wang Y. Role of Gated Myocardial Glucose Metabolic Imaging in Assessing Left Ventricular Systolic Dyssynchrony after Myocardial Infarction and the Influential Factors. Sci Rep 2018;8:11178. [Crossref] [PubMed]
- Zafrir N. Left ventricular mechanical dyssynchrony graduation of myocardial perfusion gated SPECT phase analysis: What next. J Nucl Cardiol 2018;25:1009-11. [Crossref] [PubMed]
- Juarez-Orozco LE, Monroy-Gonzalez A, Prakken NHJ, Noordzij W, Knuuti J, deKemp RA, Slart RHJA. Phase analysis of gated PET in the evaluation of mechanical ventricular synchrony: A narrative overview. J Nucl Cardiol 2019;26:1904-13. [Crossref] [PubMed]
- Thygesen K, Alpert JS, Jaffe AS, Simoons ML, Chaitman BR, White HD, et al. Third universal definition of myocardial infarction. Circulation 2012;126:2020-35. [Crossref] [PubMed]
- Pretorius PH, Xia W, King MA, Tsui BM, Pan TS, Villegas BJ. Evaluation of right and left ventricular volume and ejection fraction using a mathematical cardiac torso phantom. J Nucl Med 1997;38:1528-35. [PubMed]
- WILCOXIN F. Probability tables for individual comparisons by ranking methods. Biometrics 1947;3:119-22. [Crossref] [PubMed]
- Altman DG, Bland JM. Measurement in medicine: the Analysis of Method Comparison Studies. Statistician 1983;32:307-17. [Crossref]
- Bland JM, Altman DG. Statistical methods for assessing agreement between two methods of clinical measurement. Lancet 1986;1:307-10. [Crossref] [PubMed]
- Yamakawa Y, Takahashi N, Ishikawa T, Uchino K, Mochida Y, Ebina T, Kobayashi T, Matsushita K, Matsumoto K, Kawasaki N, Shimura M, Ohkusu Y, Sumita S, Kimura K, Inoue T, Umemura S. Clinical usefulness of ECG-gated 18F-FDG PET combined with 99mTC-MIBI gated SPECT for evaluating myocardial viability and function. Ann Nucl Med 2004;18:375-83. [Crossref] [PubMed]
- Wei H, Tian C, Schindler TH, Qiu M, Lu M, Shen R, Tian Y, Zhao SH, Zhang X. The impacts of severe perfusion defects, akinetic/dyskinetic segments, and viable myocardium on the accuracy of volumes and LVEF measured by gated 99mTc-MIBI SPECT and gated 18F-FDG PET in patients with left ventricular aneurysm: cardiac magnetic resonance imaging as the reference. J Nucl Cardiol 2014;21:1230-44. [Crossref] [PubMed]
- Tsuboi K, Fuse H, Sasaki M. Evaluation of left ventricular volumes and ejection fraction by gated myocardial perfusion SPECT versus cardiac MRI. Nihon Hoshasen Gijutsu Gakkai Zasshi 2011;67:1304-13. [Crossref] [PubMed]
- Li Y, Wang L, Zhao SH, He ZX, Wang DY, Guo F, Fang W, Yang MF. Gated F-18 FDG PET for assessment of left ventricular volumes and ejection fraction using QGS and 4D-MSPECT in patients with heart failure: a comparison with cardiac MRI. PLoS One 2014;9:e80227. [Crossref] [PubMed]
- Raja S, Mittal BR, Santhosh S, Bhattacharya A, Rohit MK. Comparison of LVEF assessed by 2D echocardiography, gated blood pool SPECT, 99mTc tetrofosmin gated SPECT, and 18F-FDG gated PET with ERNV in patients with CAD and severe LV dysfunction. Nucl Med Commun 2014;35:1156-61. [Crossref] [PubMed]
- Wang L, Wei HX, Yang MF, Guo J, Wang JF, Fang W, Wang YT. Phase analysis by gated F-18 FDG PET/CT for left ventricular dyssynchrony assessment: a comparison with gated Tc-99m sestamibi SPECT. Ann Nucl Med 2013;27:325-34. [Crossref] [PubMed]
- Tian Y, Zhao M, Li W, Zhu Z, Mi H, Li X, Zhang X. Left ventricular mechanical dyssynchrony analzyed by Tc-99m sestamibi SPECT and F-18 FDG PET in patients with ischemic cardiomyopathy and the prognostic value. Int J Cardiovasc Imaging 2020;36:2063-71. [Crossref] [PubMed]
- Ali O, Shenoy M, Alani A, Alani M, Williams K. Are SPECT MPI measures of dyssynchrony dyssynchronous? J Nucl Cardiol 2021;28:1128-35. [Crossref] [PubMed]
- Marques de Souza Filho E, Tinoco Mesquita C, Altenburg Gismondi R, de Amorim Fernandes F, Jan Verberne H. Are there normal values of phase analysis parameters for left ventricular dyssynchrony in patients with no structural cardiomyopathy?: a systematic review. Nucl Med Commun 2019;40:980-5. [Crossref] [PubMed]
- Malik D, Sood A, Parmar M, Mittal BR. Comparison of Left Ventricular Phase Parameters Analysis between Two Software Programs in Patients with Normal Gated Single-photon Emission Computed Tomography-Myocardial Perfusion Imaging. Indian J Nucl Med 2018;33:14-9. [PubMed]
- Misaka T, Hosono M, Kudo T, Ito T, Syomura T, Uemura M, Okajima K. Influence of acquisition orbit on phase analysis of gated single photon emission computed tomography myocardial perfusion imaging for assessment of left ventricular mechanical dyssynchrony. Ann Nucl Med 2017;31:235-44. [Crossref] [PubMed]
- Kortelainen MJ, Koivumäki TM, Vauhkonen MJ, Hakulinen MA. Time-modified OSEM algorithm for more robust assessment of left ventricular dyssynchrony with phase analysis in ECG-gated myocardial perfusion SPECT. EJNMMI Phys 2019;6:30. [Crossref] [PubMed]
- Wang J, Wang Y, Zhang X, Zhou R, Niu R, Lu P. Left ventricular systolic synchrony assessed by phase analysis of gated myocardial perfusion imaging in patients with old myocardial infarction. Zhonghua Xin Xue Guan Bing Za Zhi 2015;43:599-604. [PubMed]