Patient-specific 3D printed pulmonary artery model with simulation of peripheral pulmonary embolism for developing optimal computed tomography pulmonary angiography protocols
Introduction
Computed tomography pulmonary angiography (CTPA) is increasingly used in the diagnosis of patients with suspected pulmonary embolism (PE) due to its high sensitivity and specificity for detecting segmental and subsegmental PE (1-5). However, the high radiation dose associated with the increased use of CTPA examinations has raised concerns leading to the paradigm shift of developing optimal CTPA protocols to lower radiation dose while still achieving diagnostic images. Low-dose CTPA protocols have been reported in some studies showing the feasibility of reducing tube voltage to 80 and 70 kVp with use of high pitch values with resultant effective dose of less than 2 mSv (6-13). The main disadvantage of lowering tube voltage during CTPA is associated with increased image noise, with noise further increased when a high pitch protocol is used. Use of iterative reconstruction (IR) algorithms have been shown to compensate for increased image noise arising from low kVp protocols, thus improving image quality for diagnosis of PE (14-17). This has created potential opportunities for developing low-dose CT protocols through combining low kVp and high pitch protocols with IR.
Testing different CT protocols on a 3D printed realistic anatomy model represents a new research direction for investigation of optimal CT angiography protocols as 3D printed models accurately replicate both normal anatomical structures and pathologies (18-23). In our previous papers, we reported how we developed a patient-specific 3D printed pulmonary artery model with high accuracy, and tested different CTPA protocols on the model with simulation of thrombus in the main pulmonary arteries (24,25). Through quantitative assessment of image quality, we concluded that low-dose CTPA is achievable with tube voltage lowering to 100 and 80 kVp and use of high pitch 3.2 with more than 80% radiation dose reduction without compromising image quality. In this study we extended our previous research by simulating thrombus in the peripheral pulmonary arteries and scanning the model with different parameters using the latest CT scanner, the 3rd generation dual-source CT, Siemens Force. Further, advanced modelled iterative reconstruction (ADMIRE) available with the Siemens Force system is the latest IR algorithm which offers higher radiation dose reduction while reducing image noise and minimizing artifacts. The purpose of this study was to determine optimal CTPA protocols for detection of small and peripheral thrombus in the pulmonary arteries with resulting low radiation dose and acceptable diagnostic images.
Methods
3D printed pulmonary artery model
This study used the same 3D printed pulmonary artery model as reported in our previous papers (24,25). The model was confirmed to be highly accurate in delineating anatomical structures of pulmonary arteries with successful simulation of PE in the main pulmonary arteries.
Simulation of thrombus in the peripheral pulmonary arteries
Animal blood clots were obtained from a local butcher with small amounts inserted into the peripheral pulmonary arteries to mimic PE. Figure 1 shows selection of small thrombus for insertion into the distal pulmonary artery branches prior to CT scans.
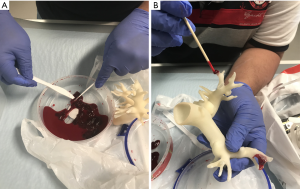
CTPA scanning protocols
Similar to our previous papers, the 3D printed model with peripheral thrombus in the pulmonary arteries was immersed in a plastic container which was filled with diluted contrast medium to create a CT attenuation of 200 HU which is similar to that of CTPA examinations (Figure 2). CTPA scans were performed on a 3rd generation dual-source 192-slice CT scanner (Siemens Force, Siemens Healthcare, Forchheim, Germany) with beam collimation of 192 mm × 0.6 mm and gantry rotation of 250 ms. The CTPA scanning protocols were as follows: 70, 80, 100 and 120 kVp, pitch of 0.9, 2.2 and 3.2, resulting in a total of 12 datasets. The tube current was adjusted for CTPA protocols with pitch of 0.9 based on the kVp, with mAs of 121, 35, 42 and 52 corresponding to 70, 80, 100 and 120 kVp, respectively. For higher pitch values of 2.2 and 3.2, 80 mAs were used for the remaining protocols, regardless of the kVp values. All images were acquired with a slice thickness of 1.0 and 0.5 mm reconstruction interval, resulting in the voxel size of 0.31×0.31×0.31 mm3 for volumetric data. All images were reconstructed with ADMIRE (Siemens Medical Solutions, Forchheim, Germany) at a strength level of 3, and a tissue convolution kernel of Br40d.
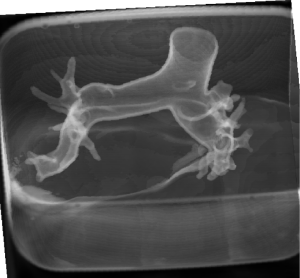
Qualitative assessment of image quality
Images were presented to two experienced thoracic radiologists (each with more than 5 years of experience in interpreting chest CT images) in a random order without showing any information about the scanning parameters. The two assessors were blinded to the scanning protocols and they assessed image quality independently using a 5-point Likert scale with a score of 3 or above indicating that image quality is diagnostic:
- 5: excellent visualization of thrombus with high confidence;
- 4: good visualization of thrombus with good confidence;
- 3: average visualization of thrombus with moderate confidence;
- 2: suboptimal visualization of thrombus with low confidence, and;
- 1: poor visualization of thrombus with no confidence.
Quantitative assessment of image quality
Quantitative assessment of image quality was determined by measuring the image noise in the main pulmonary arteries in terms of signal-to-noise ratio (SNR). A region of interest (ROI) with an area of >0.5 cm2 (containing minimum 500 voxels) was placed in both main pulmonary arteries to measure the SNR. Due to the presence of air bubbles in the pulmonary arteries, ROI was placed in the central part of the main pulmonary arteries to avoid inclusion of any air bubbles which could affect the measurements. Figure 3 shows SNR measurements in the main pulmonary arteries. Measurements at each location were repeated three times with the mean values used to reduce intra-observer variability. Two observers performed the measurements separately with excellent correlation between them (R=0.918, P<0.001). Mean values of measurements from these two observers were used as the final results.
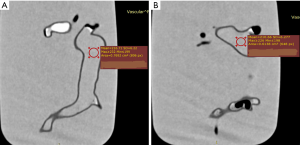
Radiation dose calculation
Volume CT dose index (CTDIvol) and dose length product (DLP) were available on CT console after scans. These values were used to calculate effective dose based on a tissue conversion coefficient of 0.014 mSv/mGy/cm for chest CT dose calculation (26).
Statistical analysis
Data were analysed using SPSS 24.0 (IBM Corporation, Armonk, NY, USA). Mean and standard deviation were used to represent continuous variables. A paired sample Student T test was used to determine any significant differences in SNR measurements among different CTPA protocols. Inter-observer agreement for image quality assessment was assessed by kappa statistics: poor: k<0.20; fair: k=0.21–0.40; moderate: k=0.41–0.60; good: k=0.61–0.80, and excellent agreement k=0.81–1.00. A statistically significant difference was reached at a P value of less than 0.05.
Results
Insertion of small blood clots into the side or peripheral pulmonary was found to be challenging due to softness of the blood clots which were easily broken into small pieces during the insertion procedure (Figure 1). We managed to insert two small blood clots in the pulmonary arteries with one in the left segmental pulmonary arterial branch and another one in the distal segment of right main pulmonary artery simulating thrombus. CTPA scans with different protocols were successfully performed on the 3D printed model with simulation of PE.
Table 1 shows SNR measurements at the main pulmonary arteries corresponding to different CTPA protocols. Although low kVp 70 and 80 protocols were associated with increased image noise when compared to 100 and 120 kVp protocols, a high CT attenuation was found in these low kVp protocols in comparison with the high kVp protocols (340–440 vs. 210–260 HU). Thus, SNR measured with the 70 kVp protocols was found to be even higher than that with 80 or 100 kVp protocols as shown in Table 1. There were no significant differences in SNR measurements across all CTPA protocols (P>0.05), regardless of the pitch or kVp values. SNR was slightly higher in the 120 kVp with pitch 0.9 and 2.2 protocols, however, this did not reach statistical significance compared to other protocols (P>0.05).
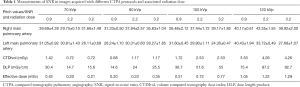
Full table
Figures 4-7 show coronal reformatted images acquired with different CTPA protocols demonstrating pulmonary emboli in the pulmonary artery branches. Despite low kVp or high pitch protocols, thrombi are still visible in all images, although 100 and 120 kVp protocols allowed for better visualization of the small thrombus, especially at the left side. Inter-observer agreement was fair (k=0.333, P=0.118). The protocol of 70 kVp and pitch 3.2 was scored 3, the lowest score by these two assessors, indicating that images are still acceptable with the low-dose protocol. All of the remaining images were scored 4 or 5 by the two assessors.
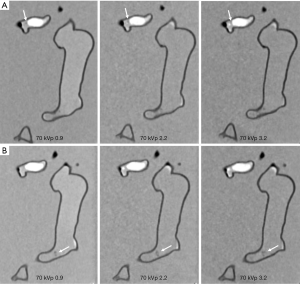
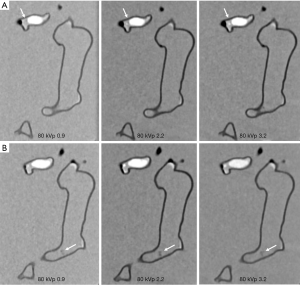
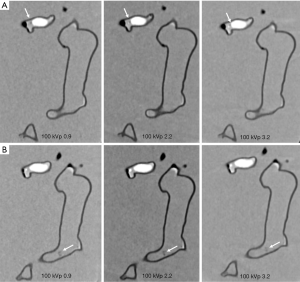
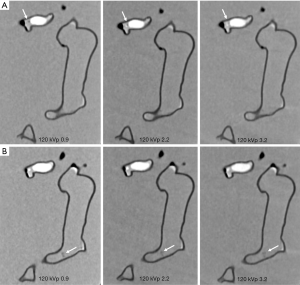
Table 1 shows radiation dose values associated with these CTPA protocols. When kVp was reduced from 120 to 70 and pitch was increased from 0.9 to 2.2 or 3.2, radiation dose was reduced by up to 84% without compromising diagnostic image quality as evaluated by qualitative and quantitative assessments. It should be noted that CTDIvol remains the same for pitch of 2.2 and 3.2 protocols and this is due to the use of same mAs of 80 for these high pitch protocols. Therefore, as shown in Table 1, the effective dose of these high pitch protocols remains unchanged or even slightly increased. Further, due to selection of different mAs corresponding to kVp values in the low pitch 0.9 protocols, the CTPA protocol of 80 kVp and 0.9 pitch resulted in the lowest dose compared to other protocols. This needs to be interpreted with caution.
Discussion
In this phantom study, we further confirmed the feasibility of low-dose CTPA protocols with simulation of thrombus in peripheral pulmonary arteries. Quantitative assessment of image quality did not show any significant differences among these CTPA protocols with 70 kVp protocols even producing higher SNR than that of 80 or 100 kVp protocols. Qualitative analysis of image quality by experienced observers showed that all of the images are acceptable for detection of small thrombus, despite lowering kVp to 70 or increasing pitch to 3.2. Radiation dose reduction by more than 80% could be achieved with use of low-dose CTPA protocol while maintaining diagnostic image quality.
Low-dose CTPA has been reported in the literature with radiation dose down to 2 mSv or even less than 1.0 mSv (7-12). Lu et al. compared 80 kVp and 2.2 pitch protocol with 100 kVp and pitch 1.2 in 100 patients with suspected PE with 50 cases in each group (8). No significant difference was found in subjective scoring of image quality between the two groups, while quantitative measurements of image quality were significantly higher in the low-dose protocol than the standard group, with significant dose reduction achieved in the low kVp and high pitch protocol (0.9 vs. 1.7 mSv). Li and colleagues reported similar findings by using 70 kVp and high pitch 3.2 CTPA protocol in 80 patients with 40 in each group (9). Their results showed no significant difference in the subjective image quality but with significantly higher quantitative assessments in the low-dose group. Up to 80% dose reduction was noted in the 70 kVp and 3.2 protocol (0.4 vs. 2.0 mSv). Our results are consistent with these findings. Low-dose CTPA protocol comprising 70 kVp and 3.2 pitch was scored to be acceptable for detection of small thrombus in the pulmonary arteries, with more than 80% dose reduction. The effective dose of the low-dose protocol in our study is between 0.20 and 0.21 mSv, almost half of what has been reported by Li’s study. Thus, our study validates the feasibility of using lower kVp and high pitch CTPA protocol with further dose reduction.
Despite the great benefit of reducing radiation dose with a high-pitch CT protocol, the disadvantage of increasing pitch is associated with increased image noise which could affect diagnostic quality. This was noticed in our recent paper with increased image noise in the 70 kVp protocol with a pitch of 3.2 affecting visualization of thrombus and pulmonary arterial wall (25). With the latest CT scanners, advanced IR algorithms are developed to improve image quality by reducing image noise associated with the use of low-dose CT protocols (27,28). This is confirmed by findings in our study as the IR available with the Siemens Force scanner represents the latest algorithm used in image reconstruction for reducing image noise. A combination of low-dose protocol with advanced IR resulted in increased quantitative image quality, with low kVp 70 and high pitch 2.2 and 3.2 protocols still producing diagnostic images, but with much lower radiation dose.
3D printed models derived from patient’s imaging data are increasingly used in medical applications, with most of the studies focusing on the clinical value of patient-specific 3D printed models such as pre-surgical planning and simulation, medical education and patient-doctor communication (18-23,29,30). A new research direction of clinical application of 3D printed models is to develop optimal CT scanning protocols for radiation dose reduction. Abdullah et al. created a 3D printed cardiac phantom based on CT images of anthropomorphic chest phantom and inserted filling materials into the phantom to simulate different anatomical structures (31). CT scans of the 3D printed model showed that CT attenuations of these filling materials were similar to those from patient’s CT images (contrast medium, air, oil/fat and jelly/muscle). Despite the novel design of this cardiac insert phantom, testing different scanning protocols on the 3D printed model remains to be investigated.
Our recent papers have addressed this limitation by developing a realistic pulmonary artery model with different CTPA protocols tested on the model with simulation of PE (24,25). With model’s accuracy validated in the first paper, we scanned the model with a combination of different kVp and pitch values on a 128-slice dual-source CT scanner by inserting thrombus in the main pulmonary arteries. A dose reduction of 80% was achieved with use of low kVp 80 and high pitch 3.2 protocol when compared to the standard 100 or 120 kVp protocols, while low-dose 70 kVp and high pitch 3.2 was not recommended due to high image noise (25). In the current study, we simulated small thrombus in peripheral pulmonary arteries and scanned the 3D printed model using the same CTPA protocols as in our previous paper, but on a latest CT scanner with use of advanced IR algorithm for image reconstruction. No significant differences were found in SNR measurements among all the protocols, with all images scored as diagnostic by two observers. Therefore, low-dose CTPA with 70 kVp and high pitch 2.2 or 3.2 is acceptable for detection of small PE with dose reduction up to 80%. Findings of this study further advanced our previous research and others, thus contributing to the current literature by recommending low-dose CTPA protocols with significant dose reduction.
Some limitations in this study should be acknowledged. Limitations that have been addressed in our previous papers still apply to the current study, such as the necessity of simulating a realistic anatomical environment with lungs, ribs, heart and other thoracic structures. Although small thrombus was inserted into in the left peripheral pulmonary artery branch, we failed to insert the thrombus in other small branches of the right pulmonary artery due to difficulty with handling the soft blood clots during the procedure. This could be addressed in future studies by simulating peripheral PE which is made of materials with similar attenuation to that of blood but with solid properties which can be easily deployed in the 3D printed models. Finally, air bubbles which are present in the pulmonary artery branches could affect the visualization and assessment of image quality to some extent (Figure 8). This needs to be considered in further experiments with approaches undertaken to reduce the negative impact of air bubbles.
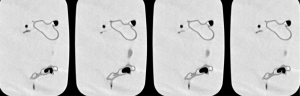
In conclusion, we have simulated the small PE in the 3D printed pulmonary model and scanned the model with different CT pulmonary angiography protocols using the latest 3rd generation dual-source CT scanner with images reconstructed using the advanced IR algorithm. Low-dose CTPA protocols comprising low kVp 70 and high pitch of 2.2 or 3.2 result in more than 80% dose reduction, but still producing acceptable image quality as determined by quantitative and qualitative assessments. The small PE can still be detected on this low-dose CTPA protocol, further validating the feasibility of lowering kVp to 70 and increasing pitch up to 3.2 for diagnosis of patients with suspected PE. Findings of this study could stimulate further similar research to develop optimal CT scanning protocols in other areas based on realistic 3D printed models.
Acknowledgements
Authors would like to thank Mr Tom Tiang from Perth Children’s Hospital for his assistance in CT scanning of the pulmonary artery model. We think Mr Gil Stevenson for his assistance in the statistical analysis.
Footnote
Conflicts of Interest: The authors have no conflicts of interest to declare.
Ethical Statement: Ethical approval was waived in this study as it is based on a phantom experiment.
References
- Albrecht MH, Bickford MW, Nance JW Jr, Zhang L, De Cecco CN, Wichmann JL, Vogl TJ, Schoepf UJ. State-of-the-Art pulmonary CT angiography for acute pulmonary embolism. AJR Am J Roentgenol 2017;208:495-504. [Crossref] [PubMed]
- Sun Z, Lei J. Diagnostic yield of CT pulmonary angiography in the diagnosis of pulmonary embolism: a single center experience. Interv Cardiol 2017;9:191-8. [Crossref]
- Chen EL, Ross JA, Grant C, Wilbur A, Mehta N, Hart E, Mar WA. Improved image quality of low-dose CT pulmonary angiograms. J Am Coll Radiol 2017;14:648-53. [Crossref] [PubMed]
- Devaraj A, Sayer C, Sheard S, Grubnic S, Nair A, Vlahos I. Diagnosing acute pulmonary embolism with computed tomography: imaging update. J Thorac Imaging 2015;30:176-92. [Crossref] [PubMed]
- Henzler T, Barraza JM, Nance JW Jr, Costello P, Krissak R, Fink C, Schoepf UJ. CT imaging of acute pulmonary embolism. J Cardiovasc Comput Tomogr 2011;5:3-11. [Crossref] [PubMed]
- Wichmann JL, Hu X, Kerl JM, Schulz B, Frellesen C, Bodelle B, Kaup M, Scholtz JE, Lehnert T, Vogl TJ, Bauer RW. 70 kVp computed tomography pulmonary angiography: potential for reduction of iodine load and radiation dose. J Thorac Imaging 2015;30:69-76. [Crossref] [PubMed]
- Martini K, Meier A, Higashhigaito K, Saltybaeva N, Alkadhi H, Frauenfelder T. Prospective randomized comparison of high-pitch CT at 80 kVp under free breathing with standard-pitch CT at 100 kVp under breath-hold for detection of pulmonary embolism. Acad Radiol 2016;23:1335-41. [Crossref] [PubMed]
- Lu GM, Luo S, Meinel FG, McQuiston AD, Zhou CS, Kong X, Zhao YE, Zheng L, Schoepf UJ, Zhang LJ. High-pitch computed tomography pulmonary angiography with iterative reconstruction at 80 kVp and 20 mL contrast agent volume. Eur Radiol 2014;24:3260-8. [Crossref] [PubMed]
- Li X, Ni QQ, Schoepf UJ, Wichmann JL, Felmly LM, Qi L, Kong X, Zhou CS, Luo S, Zhang LJ, Lu GM. 70-kVp high-pitch computed tomography pulmonary angiography with 40 mL contrast agent: initial experience. Acad Radiol 2015;22:1562-70. [Crossref] [PubMed]
- Bolen MA, Renapurkar RD, Popovic ZB, Popovic ZB, Heresi GA, Flamm SD, Lau CT, Lau CT, Halliburton SS. High-pitch ECG synchronized pulmonary CT angiography versus standard CT pulmonary angiography: a prospective randomized study. AJR Am J Roentgenol 2013;201:971-6. [Crossref] [PubMed]
- Sabel BO, Buric K, Karara N, Thierfelder KM, Dinkel J, Sommer WH, Meinel FG. High-pitch CT pulmonary angiography in third generation dual-source CT: image quality in an unselected patient population. PLoS ONE 2016;11:e0146949. [Crossref] [PubMed]
- Boos J, Kropil P, Lanzman RS, Aissa J, Schleich C, Heusch P, Sawichi LM, Antoch G, Thomas C. CT pulmonary angiography: simultaneous low-pitch dual-source acquisition mode with 70 kVp and 40 ml of contrast medium and comparison with high-pitch spiral dual-source acquisition with automated tube potential selection. Br J Radiol 2016;89:20151059. [Crossref] [PubMed]
- Bucher AM, Kerl MJ, Albrecht MH, Beeres M, Ackermann H, Wichmann JL, Vogl TJ, Bauer RW, Lehnert T. Systematic comparison of reduced tube current protocols for high-pitch and standard-pitch pulmonary CT angiography in a large single-center population. Acad Radiol 2016;23:619-27. [Crossref] [PubMed]
- Kligerman S, Lahiji K, Weihe E, Lin CT, Terpenning S, Jeudy J, Frazier A, Pugatch R, Galvin JR, Mittal D, Kothari K, White CS. Detection of pulmonary embolism on computed tomography: improvement using a model-based iterative reconstruction algorithm compared with filtered back projection and iterative reconstruction algorithms. J Thorac Imaging 2015;30:60-8. [Crossref] [PubMed]
- Laqmani A, Regier M, Veldhoen S, Backhaus A, Wassenberg F, Sehner S, Groth M, Nagel HD, Adam G, Henes FO. Improved image quality and low radiation dose with hybrid iterative reconstruction with 80 kV CT pulmonary angiography. Eur J Radiol 2014;83:1962-9. [Crossref] [PubMed]
- McLaughlin PD, Liang T, Homiedan M, Louis LJ, O'Connell TW, Krzymyk K, Nicolaou S, Mayo JR. High pitch, low voltage dual source CT pulmonary angiography: assessment of image quality and diagnostic acceptability with hybrid iterative reconstruction. Emerg Radiol 2015;22:117-23. [Crossref] [PubMed]
- Wortman JR, Adduci AJ, Sodickson AD. Synergistic radiation dose reduction by combining automatic tube voltage selection and iterative reconstruction. J Thorac Imaging 2016;31:111-8. [Crossref] [PubMed]
- Olivieri LJ, Krieger A, Loke YH, Nath DS, Kim PC, Sable CA. Three-dimensional printing of intracardiac defects from three-dimensional echocardiographic images: feasibility and relative accuracy. J Am Soc Echocardiogr 2015;28:392-7. [Crossref] [PubMed]
- Cantinotti M, Valverde I, Kutty S. Three-dimensional printed models in congenital heart disease. Int J Cardiovasc Imaging 2017;33:137-44. [Crossref] [PubMed]
- Lau IW, Liu D, Xu L, Fan Z, Sun Z. Clinical value of patient-specific three-dimensional printing of congenital heart disease: Quantitative and qualitative Assessments. PLoS One 2018;13:e0194333. [Crossref] [PubMed]
- Liu D, Sun Z, Chaichana T, Ducke W, Fan Z. Patient-specific 3D printed models of renal tumours using home-made 3D printer in comparison with commercial 3D printer. J Med Imaging Health Inf 2018;8:303-8. [Crossref]
- Sun Z, Liu D. A systematic review of clinical value of three-dimensional printing in renal disease. Quant Imaging Med Surg 2018;8:311-25. [Crossref] [PubMed]
- Lau I, Sun Z. Three-dimensional printing in congenital heart disease: A systematic review. J Med Radiat Sci 2018;65:226-36. [Crossref] [PubMed]
- Aldosari S, Squelch A, Sun Z. Patient-specific 3D printed pulmonary artery model: A preliminary study. Digit Med 2017;3:170-7. [Crossref]
- Aldosari S, Jansen S, Sun Z. Optimization of computed tomography pulmonary angiography using 3D printed model with simulation of pulmonary embolism. Quant Imaging Med Surg 2019;9:53-62.
- McCollough CH, Primak AN, Braun N, Kofler J, Yu L, Christner J. Strategies for reducing radiation dose in CT. Radiol Clin North Am 2009;47:27-40. [Crossref] [PubMed]
- Leithner D, Gruber-Rouh T, Beeres M, Wichmann JL, Mahmoudi S, Martin SS, Lenga L, Albrecht MH, Booz C, Vogl TJ, Scholtz JE. 90-kVp low-tube-voltage CT pulmonary angiography in combination with advanced modeled iterative reconstruction algorithm: effects on radiation dose, image quality and diagnostic accuracy for the detection of pulmonary embolism. Br J Radiol 2018;91:20180269. [Crossref] [PubMed]
- Leithner D, Wichmann JL, Mahmoudi S, Martin SS, Albrecht MH, Vogl TJ, Scholtz JE. Diagnostic yield of 90-kVp low-tube-voltage carotid and intracerebral CT-angiography: effects on radiation dose, image quality and diagnostic performance for the detection of carotid stenosis. Br J Radiol 2018;91:20170927. [Crossref] [PubMed]
- White SC, Sedler J, Jones TW, Seckeler M. Utility of three-dimensional models in resident education on simple and complex intracardiac congenital heart defects. Congenit Heart Dis 2018;13:1045-9. [Crossref] [PubMed]
- Loke YH, Harahsheh AS, Krieger A, Olivieri LJ. Usage of 3D models of tetralogy of Fallot for medical education: impact on learning congenital heart disease. BMC Med Educ 2017;17:54. [Crossref] [PubMed]
- Abdullah KA, McEntee MF, Reed W, Kench PL. Development of an organ-specific insert phantom generated using a 3D printer for investigations of cardiac computed tomography protocols. J Med Radiat Sci 2018;65:175-83. [Crossref] [PubMed]