Concurrent mapping of brain activation from multiple subjects during social interaction by hyperscanning: a mini-review
Introduction
Our communication or social interactions with one another is essential for us to acquire knowledge and to develop our own personalities. Although the forms of social interactions are very broad including imitations, exchanges, completions and cooperation, as well as making decisions (1), we basically exchange our thoughts and ideas in two different manners. The dominant manner is through our sophisticated languages, which is also a characteristic that distinguishing ourselves from other creatures (1,2). The other way is by using non-verbal signs, such as our gestures and facial expressions, which can provide us with additional auxiliary information for social interactions (1,2).
Interestingly enough, although our social nature has been shaped for hundreds and thousands of years, neuroscience studies only just began to shed light on social interaction in the recent years (1,3,4). More importantly, previous neuroimaging studies have exhibited two basic limitations in elucidating neural correlates of social interactions. The first restriction is from the low ecological validity, since most of the previous experiments were performed in an enclosed room, in which individuals were instructed by computer programs, to complete the test tasks (2). However, this is not the case for social interactions in real life, in which individuals need to talk and act with each other simultaneously in a more natural way. Therefore, further neuroscience or neuroimaging studies should be performed by using a more realistic experimental paradigm which can duplicate a real-life situation. The other limitation is that previous studies can only acquire brain data from a single participant each time (5). However, as two or more individuals are engaged in social interactions, it is essential to conduct a concurrent recording from multiple subjects with multiple setups rather than to perform it in isolation (1,2,5).
Recently, a new strategy that had combined two functional magnetic resonance imaging (fMRI) machines together for simultaneously measuring two participants’ brain activity was adopted, which was coined as “hyperscanning” method (6). Since then, extensive hyperscanning studies have been performed, which improves our understanding of brain-to-brain synchronization during a social interaction (7). To date, hyperscanning has enabled the inspection of social interaction by using various neuroimaging techniques such as electroencephalograph (EEG) (8-30), functional near inferred spectroscopy (fNIRS) (31-48) and fMRI (49-55). Meanwhile, the experimental paradigms involved in hyperscanning studies can be categorized into six types of tasks: (I) imitation tasks; (II) coordination/joint tasks; (III) eye contact/gaze tasks; (IV) economic games/exchanges; (V) cooperation and competition tasks; and (VI) interactions under natural scenario. In particular, it is noted that during the performance of all those tasks, two major neural systems are largely involved (1,2,5). One is the mirror neuron system (MNS), which plays an important role in tasks involving movements, such as imitation and coordination/joint tasks (56). The other is a mentalizing system (MS), which is engaged in tasks pertaining to the inferences of yourself or others’ intentions or thoughts (57), such as the economic game (58) and natural social interactions (33,36).
In this review, fMRI, EEG and fNIRS hyperscanning neuroimaging technologies which have engaged in social interaction are first introduced. Then, the representative experimental paradigms that were extensively adopted in hyperscanning, are also summarized in detail. Subsequently, two core neural systems involved in social interactions are carefully demonstrated. One is MNS, which consists of the primary motor, sensory cortex and parietal cortex, and is responsible for the imitation process; the second one is MS comprising the TPJ (temporal-parietal cortex) and PFC (prefrontal cortex), which is in charge of a more complex cognitive process. More importantly, the future of research perspectives and clinical implications of hyperscanning, are stated clearly in the final section.
Hyperscanning neuroimaging techniques
fMRI hyperscanning
As it is hard to place two or more participants into one fMRI tube, two or more fMRI machines should be utilized for an fMRI hyperscanning method to simultaneously record multiple participants’ brain signals. In that circumstance, two or more remote fMRI apparatus can be connected by an intranet, while the data sets are stored in a host client (Figure 1A) (6). To date, several fMRI hyperscanning studies (49-55) have been conducted to inspect the inter-brain synchrony (Table 1). For example, neural correlates of trust between two individuals, had been examined by fMRI hyperscanning. They had discovered that trust is an essential social process, involved in all human interaction (54). Inarguably, fMRI hyperscanning has exhibited its advantages in mapping the coherence of brain regions which were associated with social interaction with high structural accuracy and excellent imaging depth. However, it is not accessible and available for everyone, because of the high cost of multiple fMRI setups. More importantly, the ecological validity is also relatively low, since the lab was under the controlled circumstances for fMRI, and is significantly different from real life.
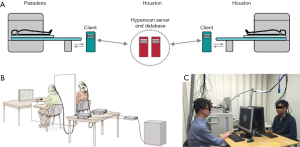
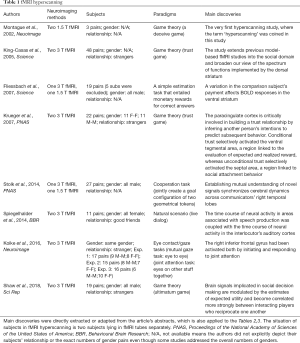
Full table
EEG hyperscanning
Since the electrical activity of human brain was firstly recorded by Hans Berger in 1924, EEG has become a core neuroimaging tool in the study of cognition and diseases (59,60). More importantly, EEG is also one of the most powerful techniques for noninvasively exploring neural oscillations (61), in which the EEG signals are originated from the synchronized synaptic activity in populations of cortical neurons (62). Although EEG has been extensively utilized for mapping single individual’s brain dynamics underlying specific cognitive tasks, the potential of EEG in exploring the inter-brain interactions or inter-brain connections has not been fully exploited.
Recently, a number of EEG hyperscanning studies (Table 2) were conducted (8-30), aiming to reveal the complex brain interactions between two or multiple participants, as illustrated in Figure 1B (26). These studies exhibited that EEG hyperscanning can map the moment-to-moment interactions between two or more individuals simultaneously, which can elucidate how co-variations of the tested individuals’ brain activations are correlated with their social interactions. However, despite EEG being suitable to inspect inter-brain synchronization due to its high time resolution, it is still very challenging for EEG to capture the neural activity from deep brain structures.
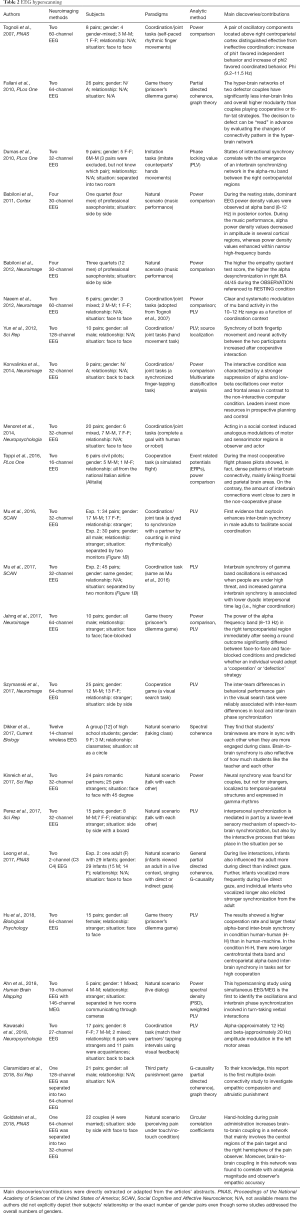
Full table
fNIRS hyperscanning
fNIRS is also a noninvasive and affordable neuroimaging technique, which utilizes the near-infrared light to image brain activation, by measuring the concentration changes of oxyhemoglobin (HbO) and deoxyhemoglobin (HbR) (63-65). In addition, fNIRS has exhibited its unbeatable advantages in inspecting infants or children’s brain activation (66) since it is relatively more tolerant with movement artifacts. More importantly, fNIRS hyperscanning (Table 3) can ideally be applied to a natural scenario (33,35,36,39), as illustrated in Figure 1C. Although fNIRS has a better temporal resolution when compared to fMRI, it has the low spatial resolution and limited capability to detect deep brain structures.
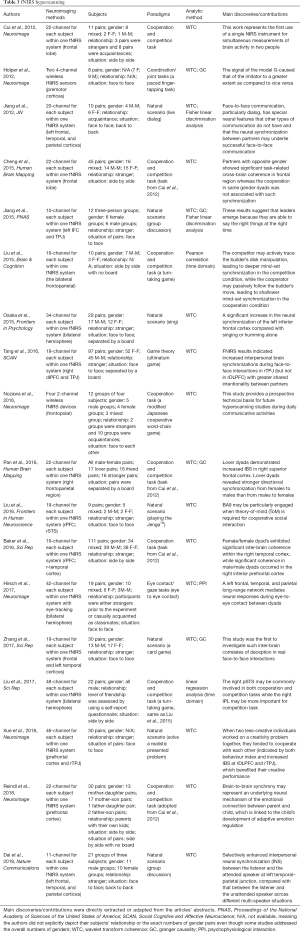
Full table
Hyperscanning paradigms adopted in social interaction
Altogether, there were about six categories of experimental paradigms that were routinely used by the hyperscanning method in the investigation of social interaction.
Imitation tasks
The first category is the imitation tasks, during which one participant imitates the others’ movements or behaviors. Although we cannot request one participant to teach the others how to perform specific tasks in the laboratory, we can still instruct one participant to simulate the other individual’s actions or behaviors (Figure 2A). For example, in one EEG hyperscanning study (17), the participant was instructed to imitate the counterpart’s meaningless hand movements. The results showed that inter-brain synchronization of right centroparietal regions at alpha-mu band was strongly correlated with the interactional synchrony (Figure 2B).
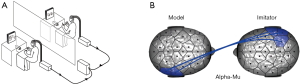
Coordination tasks
The second category is the coordination tasks, in which two or more participants need to try their best to act in a synchronized manner. Interestingly, behavioral synchronization in our daily life is one mechanism through which we coordinate our behaviors during social interaction. For example, when we are walking together, our footsteps might be unconsciously synchronized with one another even though our foot lengths and our intrinsic cycles are totally different (22). In addition, coordination/joint movements can also be synchronized, such as self-paced rhythmic finger movements (15,16). In particular, a number of EEG or fNIRS hyperscanning studies have been performed to examine the neural synchronizations in coordination/joint movements (9,15,16,20,22,24,29). One example was illustrated in the previous reports (20,24), in which dyads were instructed to synchronize with each other by counting in their mind rhythmically. This study also examined how the social context such as threats, or oxytocin, affected the coordinated movements (20,24), which showed that oxytocin can enhance inter-brain synchronization to facilitate social coordination (20).
Eye contact/gaze tasks
The third category was the eye contact or gaze tasks, in which dyads are instructed to look in each other’s eyes, or look towards the third object. Interestingly, the mutual gaze or eye-to-eye contact has the functions that offer pivotal social cues in social interaction and communication. In particular, a universally recognized social link or a pipeline can be established during a non-verbal communication through eye contact or a mutual gaze (35). Importantly, we can infer the others’ intentions as well using eye-to-eye contact (50). Further, eye-to-eye contact, through which reciprocal information between individuals are dynamically exchanged, provides a great opportunity to model the neural mechanisms of human interpersonal communication by hyperscanning neuroimaging techniques (35,50). For example, an interesting study was performed, in which the dyads were instructed to look at each other’s eyes or eyes in portraits (35). And they discovered that the inter-brain coherence of the left superior temporal, middle temporal and supramarginal gyri as well as the pre- and supplementary motor cortices were significantly increased in the eye-to-eye contact case when compared to the data from the eye-to-picture gaze case (35).
Economic games involving game theory/exchange tasks
The fourth category is playing economic games/exchange tasks, in which one participant provided an economic offer while the counterpart need to make a decision on whether they wanted to take it or not. Game theory can offer a rich collection of both behavioral tasks and well-specified models aiming to articulate social interactions where decision-makers have to interact with one another (58). By contrast, exchange is the most basic type of social interaction, which involves a social process whereby social behavior is exchanged for some type of reward for equal or greater value. One instantiation of game theory/exchange is the trust game, in which one participant need decide how much money should be returned to your opponent (52), as illustrated in Figure 3A. One hyperscanning study illustrated that the paracingulate cortex is critically involved in building a trustworthy relationship (54). In addition, the prisoner’s dilemma game was also utilized as a task for the design of hyperscanning. This required the two participants to make their own decisions simultaneously (Figure 3B). The prisoner’s dilemma game usually consists of three experimental conditions: win-win, lose-lose, and a tit for tat case (14,21,27). Interestingly, previous reports have demonstrated that the decision to defect can be decoded in advance by monitoring the changes of connectivity patterns, as shown in Figure 3C (21). Further, the ultimatum game is also applied to the paradigm design for hyperscanning (Figure 3D), in which one participant need decide to take your opponent’s offer or not (34,55).
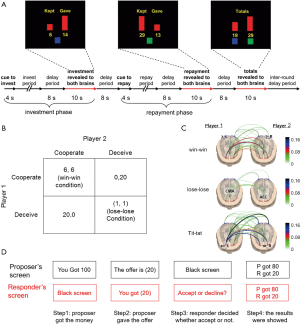
Cooperation and competition tasks
The fifth category is cooperation and competition tasks, in which participants need to achieve a goal cooperatively or competitively. Cooperation and competition tasks are ubiquitous, in which goals should be obtained efficiently. One representative paradigm used in the hyperscanning studies was to explore the brain synchronization’s underlying cooperation or competition, as plotted in Figure 4A, which consisted of three conditions: the cooperate, competitive, and control conditions (31). Interestingly, this paradigm was first initiated in 2012, and later was utilized to examine the brain coherence differences between groups of the same sex and of mixed sexes (Figure 4B) (40,44), groups with lovers and strangers (Figure 4C) (42), or groups with parents verse the child and the stranger verse the child (38). In addition, other paradigm designs were also formulated to inspect the inter-brain synchronization engaged in cooperation and competition (13,32,41,46).
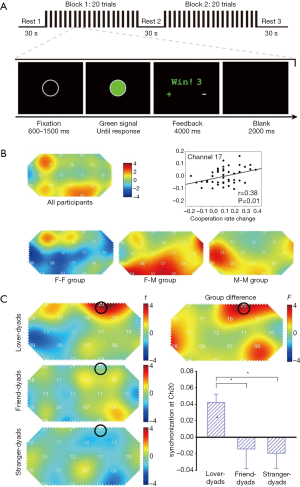
Natural scenario
The paradigms mentioned above do offer great opportunities in inspecting the inter-brain dynamics during social interaction. However, only social interaction through a natural scenario can reflect the real situations in our daily life, which is also the dominated way of communication and thought exchange. An interesting test has been performed, in which two participants were instructed to have conversations with each other while their neural data was concurrently recorded (25,26,28,33,36,39,51). Intriguingly, their findings showed that inter-brain synchronization was higher for a face-to-face talk case, as compared to that of the back-to-back talk case (39). In addition, neural synchronization under other circumstances was also explored, such as music playing (18,19), singing together (43), playing games (47,48) or taking a class (10). For example, one study showed that during class time, students’ brainwaves are more in sync with each other while they are highly engaged in the teaching (10).
Neural systems involved in hyperscanning during social interaction
Two main neural systems are involved in inter-brain connections (1,2,5). One is the MNS, which includes the primary motor cortex and posterior parietal cortex. The second one is the MS, which consists of the temporal-parietal junction (TPJ), precuneus and prefrontal cortex (PFC).
MNS
When we imitate or even just see the others’ actions or movements, neurons in the MNS are fired. This phenomenon was discovered in both monkey and human brains (56). In human brains, the MNS (Figure 5) consists of the inferior frontal gyrus (IFG) and inferior parietal lobule (IPL), which is related to language, motor and sensory detection. In addition, the superior temporal gyrus (STG) also plays an essential role in imitations, which can provide additional visual information inputs (56), in which the encoded information of imitated actions is first transformed into a more sophisticated visual representation through STG and then is delivered to the IPL. Once the IPL is activated, potential movements are able to be executed. In addition, the IFG is also activated to manipulate the potential action, which can provide additional supplemental information, such as the goal of the action.
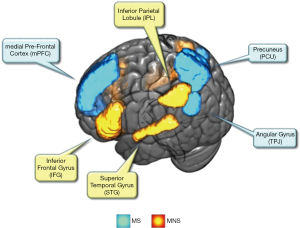
The present hyperscanning studies associated with imitation show empirical evidence that MNS is involved in dual participant imitation (8,17). For example, one study demonstrated that when two participants were synchronized in behaviors, their brains were also tuned to the same frequency. Consequently, an inter-brain sychornizing network in the alpha-mu band between the right centroparietal regions was produced (Figure 2B).
MS
Besides imitating others’ actions, we might as well try to understand others’ intentions or emotions by their gestures, behaviors and facial expressions, which is termed as mentalizing (57,68). The TPJ and PFC particularly, and the dorsomedial PFC (DMPFC) are the two main brain regions associated with the mentalizing process (68).
The TPJ is the boundary brain region between the temporal and parietal cortex, which is labelled in a red circled area in Figure 5 (67). As depicted in a previous study (69), the mentalizing process contains two steps. In the first step, the static social images are coded as a neural representation from the extrastriate body area. For step two, the encoded representations are constructed to generate moving social entities, and are then incorporated into a context for interpreting the intention. Interestingly, several fNIRS hyperscanning studies have highlighted the TPJ as their region of interest (33,34,39). For example, in an adapted version of the ultimatum game, the interpersonal brain coherence for the right TPJ was higher for underlying the face-to-face condition, than that of the face blocked condition. This indicated the functions of right TPJ, is collaborative in social interactions (34).
The PFC, likes a commander, is also involved in the mentalizing processes. It is responsible for the planning, regulation, integrating of information, and other high cognitive functions. Accumulated neuroimaging evidences have shown that the PFC was related to interpersonal brain synchronization (31-34,39,41). For example, the inter-brain coherence in left inferior frontal cortex was significantly higher in face-to-face dialogues, than those from back-to-back dialogues, face-to-face monologue, or back-to-back monologues (39).
In summary, both MNS and MS play vital roles in social interactions, although the relationship between them is still unclear. A few studies demonstrated that they are collaborated (70), whereas additional reports also stated that MNS is inferior to MS (57).
Future perspectives and clinical implications of hyperscanning
Multimodality hyperscanning
Further investigation should be performed by using EEG-fNIRS, fNIRS-fMRI or EEG-fMRI hyperscanning techniques, since the multimodality neuroimaging methods can take advantage of the high temporal resolution of the EEG/fNIRS and the high spatial resolutions of an fMRI. To date, hyperscanning studies that utilize two or three neuroimaging modalities (e.g., EEG & fNIRS fusion) have not been extensively examined. Interestingly, multimodality can provide us new perspectives that a single modality cannot offer, because each neuroimaging method possesses its own advantages. For example, our group recently discovered that a combed EEG and fNIRS can enhance the sensitivity of lie detections (71). Although this is not a hyperscanning study, it enlightens us to more intriguing results or findings about the inter-brain dynamics which can be identified by applying a multimodality hyperscanning method for testing social interaction. In particular, more linked neural information can be revealed, based on the fused measures from neurovascular and neuroelectrical signals, which enable us to gain a more full understanding of the inter-brain effects during social interactions in our daily life.
Applications of hyperscanning in education and interrelationships
For most of the hyperscanning studies, neural data were recorded with two participants simultaneously, although several studies were also conducted by acquiring the brain signals from three or multiple participants (10,33,36). However, inspecting multiple individuals’ brain dynamics is crucial in some circumstances such as for the teaching and education settings. For example, one study demonstrated that students’ brain-to-brain group synchrony can track not only classroom engagement but also classroom social dynamics (10). But they did not explore the neural dynamics between teachers and students. In addition, the teaching style that can stimulate students’ inter-brain synchronization by inspecting the neural dynamics between teachers and students should be further investigated.
Interestingly, hyperscanning can also be applied to examining the interactions between an adult and a child (11,38) and interpersonal relationships, such as lovers (12,25,42). For example, lovers who held their hands together exhibited their capability in alleviating their pain perception (12).
Clinical implications
The hyperscanning method has exhibited a potential for the study of inter-brain synchronization of normal individuals during social interaction. In contrast, hyperscanning of abnormal individuals might manifest an aberrant, or null interpersonal dynamics for disorder detection, particularly those in social deficiencies such as autism and schizophrenia. For example, a previous hyperscanning study showed that autism patients have the ability in recognizing their counterparty’s intentions, but they cannot convey this information (72). To date, inspecting the interpersonal neural synchronizations among aberrant populations is still lacking. As a result, it is urgent for us to elucidate the neural mechanisms underlying those social deficits disorders by hyperscanning (1), which can pave a new avenue for improving the detection and treatment of neurological or psychiatric disorders.
Acknowledgements
Funding: This work was supported by the University of Macau (MYRG2016-00110-FHS and MYRG2018-00081-FHS), and the Macao Science and Technology Development Fund (FDCT 025/2015/A1 and FDCT 0011/2018/A1).
Footnote
Conflicts of Interest: The authors have no conflicts of interest to declare.
References
- Hari R, Kujala MV. Brain basis of human social interaction: from concepts to brain imaging. Physiol Rev 2009;89:453-79. [Crossref] [PubMed]
- Hasson U, Ghazanfar AA, Galantucci B, Garrod S, Keysers C. Brain-to-brain coupling: a mechanism for creating and sharing a social world. Trends Cogn Sci 2012;16:114-21. [Crossref] [PubMed]
- Van Overwalle F. A dissociation between social mentalizing and general reasoning. Neuroimage 2011;54:1589-99. [Crossref] [PubMed]
- Van Overwalle F. Social cognition and the brain: a meta-analysis. Hum Brain Mapp 2009;30:829-58. [Crossref] [PubMed]
- Schilbach L, Timmermans B, Reddy V, Costall A, Bente G, Schlicht T, Vogeley K. Toward a second-person neuroscience. Behav Brain Sci 2013;36:393-414. [Crossref] [PubMed]
- Montague PR, Berns GS, Cohen JD, McClure SM, Pagnoni G, Dhamala M, Wiest MC, Karpov I, King RD, Apple N. Hyperscanning: simultaneous fMRI during linked social interactions. Neuroimage 2002;16:1159-64. [Crossref] [PubMed]
- Babiloni F, Astolfi L. Social neuroscience and hyperscanning techniques: past, present and future. Neurosci Biobehav Rev 2014;44:76-93. [Crossref] [PubMed]
- Ménoret M, Varnet L, Fargier R, Cheylus A, Curie A, Des Portes V, Nazir TA, Paulignan Y. Neural correlates of non-verbal social interactions: a dual-EEG study. Neuropsychologia 2014;55:85-97. [Crossref] [PubMed]
- Konvalinka I, Bauer M, Stahlhut C, Hansen LK, Roepstorff A, Frith CD. Frontal alpha oscillations distinguish leaders from followers: multivariate decoding of mutually interacting brains. Neuroimage 2014;94:79-88. [Crossref] [PubMed]
- Dikker S, Wan L, Davidesco I, Kaggen L, Oostrik M, McClintock J, Rowland J, Michalareas G, Van Bavel JJ, Ding M. Brain-to-brain synchrony tracks real-world dynamic group interactions in the classroom. Curr Biol 2017;27:1375-80. [Crossref] [PubMed]
- Leong V, Byrne E, Clackson K, Georgieva S, Lam S, Wass S. Speaker gaze increases information coupling between infant and adult brains. Proc Natl Acad Sci U S A 2017;114:13290-5. [Crossref] [PubMed]
- Goldstein P, Weissman-Fogel I, Dumas G, Shamay-Tsoory SG. Brain-to-brain coupling during handholding is associated with pain reduction. Proc Natl Acad Sci U S A 2018;115:E2528-37. [Crossref] [PubMed]
- Szymanski C, Pesquita A, Brennan AA, Perdikis D, Enns JT, Brick TR, Müller V, Lindenberger U. Teams on the same wavelength perform better: Inter-brain phase synchronization constitutes a neural substrate for social facilitation. Neuroimage 2017;152:425-36. [Crossref] [PubMed]
- Jahng J, Kralik JD, Hwang DU, Jeong J. Neural dynamics of two players when using nonverbal cues to gauge intentions to cooperate during the Prisoner's Dilemma Game. Neuroimage 2017;157:263-74. [Crossref] [PubMed]
- Naeem M, Prasad G, Watson DR, Kelso JS. Electrophysiological signatures of intentional social coordination in the 10–12Hz range. Neuroimage 2012;59:1795-803. [Crossref] [PubMed]
- Tognoli E, Lagarde J, DeGuzman GC, Kelso JS. The phi complex as a neuromarker of human social coordination. Proc Natl Acad Sci U S A 2007;104:8190-5. [Crossref] [PubMed]
- Dumas G, Nadel J, Soussignan R, Martinerie J, Garnero L. Inter-brain synchronization during social interaction. PLoS One 2010;5. [Crossref] [PubMed]
- Babiloni C, Buffo P, Vecchio F, Marzano N, Del Percio C, Spada D, Rossi S, Bruni I, Rossini PM, Perani D. Brains “in concert”: frontal oscillatory alpha rhythms and empathy in professional musicians. Neuroimage 2012;60:105-16. [Crossref] [PubMed]
- Babiloni C, Vecchio F, Infarinato F, Buffo P, Marzano N, Spada D, Rossi S, Bruni I, Rossini PM, Perani D. Simultaneous recording of electroencephalographic data in musicians playing in ensemble. Cortex 2011;47:1082-90. [Crossref] [PubMed]
- Mu Y, Guo C, Han S. Oxytocin enhances inter-brain synchrony during social coordination in male adults. Soc Cogn Affect Neurosci 2016;11:1882-93. [PubMed]
- De Vico Fallani F, Nicosia V, Sinatra R, Astolfi L, Cincotti F, Mattia D, Wilke C, Doud A, Latora V, He B. Defecting or not defecting: how to “read” human behavior during cooperative games by EEG measurements. PLoS One 2010;5. [Crossref] [PubMed]
- Yun K, Watanabe K, Shimojo S. Interpersonal body and neural synchronization as a marker of implicit social interaction. Sci Rep 2012;2:959. [Crossref] [PubMed]
- Toppi J, Borghini G, Petti M, He EJ, De Giusti V, He B, Astolfi L, Babiloni F. Investigating cooperative behavior in ecological settings: an EEG hyperscanning study. PLoS One 2016;11. [Crossref] [PubMed]
- Mu Y, Han S, Gelfand MJ. The role of gamma interbrain synchrony in social coordination when humans face territorial threats. Soc Cogn Affect Neurosci 2017;12:1614-23. [Crossref] [PubMed]
- Kinreich S, Djalovski A, Kraus L, Louzoun Y, Feldman R. Brain-to-brain synchrony during naturalistic social interactions. Sci Rep 2017;7:17060. [Crossref] [PubMed]
- Pérez A, Carreiras M, Duñabeitia JA. Brain-to-brain entrainment: EEG interbrain synchronization while speaking and listening. Sci Rep 2017;7:4190. [Crossref] [PubMed]
- Hu Y, Pan Y, Shi X, Cai Q, Li X, Cheng X. Inter-brain synchrony and cooperation context in interactive decision making. Biol Psychol 2018;133:54-62. [Crossref] [PubMed]
- Ahn S, Cho H, Kwon M, Kim K, Kwon H, Kim BS, Chang WS, Chang JW, Jun SC. Interbrain phase synchronization during turn-taking verbal interaction—a hyperscanning study using simultaneous EEG/MEG. Hum Brain Mapp 2018;39:171-88. [Crossref] [PubMed]
- Kawasaki M, Kitajo K, Yamaguchi Y. Sensory-motor synchronization in the brain corresponds to behavioral synchronization between individuals. Neuropsychologia 2018;119:59-67. [Crossref] [PubMed]
- Ciaramidaro A, Toppi J, Casper C, Freitag C, Siniatchkin M, Astolfi L. Multiple-Brain Connectivity During Third Party Punishment: an EEG Hyperscanning Study. Sci Rep 2018;8:6822. [Crossref] [PubMed]
- Cui X, Bryant DM, Reiss AL. NIRS-based hyperscanning reveals increased interpersonal coherence in superior frontal cortex during cooperation. Neuroimage 2012;59:2430-7. [Crossref] [PubMed]
- Nozawa T, Sasaki Y, Sakaki K, Yokoyama R, Kawashima R. Interpersonal frontopolar neural synchronization in group communication: An exploration toward fNIRS hyperscanning of natural interactions. Neuroimage 2016;133:484-97. [Crossref] [PubMed]
- Jiang J, Chen C, Dai B, Shi G, Ding G, Liu L, Lu C. Leader emergence through interpersonal neural synchronization. Proc Natl Acad Sci U S A 2015;112:4274-9. [Crossref] [PubMed]
- Tang H, Mai X, Wang S, Zhu C, Krueger F, Liu C. Interpersonal brain synchronization in the right temporo-parietal junction during face-to-face economic exchange. Soc Cogn Affect Neurosci 2016;11:23-32. [Crossref] [PubMed]
- Hirsch J, Zhang X, Noah JA, Ono Y. Frontal temporal and parietal systems synchronize within and across brains during live eye-to-eye contact. Neuroimage 2017;157:314-30. [Crossref] [PubMed]
- Dai B, Chen C, Long Y, Zheng L, Zhao H, Bai X, Liu W, Zhang Y, Liu L, Guo T. Neural mechanisms for selectively tuning in to the target speaker in a naturalistic noisy situation. Nat Commun 2018;9:2405. [Crossref] [PubMed]
- Holper L, Scholkmann F, Wolf M. Between-brain connectivity during imitation measured by fNIRS. Neuroimage 2012;63:212-22. [Crossref] [PubMed]
- Reindl V, Gerloff C, Scharke W, Konrad K. Brain-to-brain synchrony in parent-child dyads and the relationship with emotion regulation revealed by fNIRS-based hyperscanning. Neuroimage 2018;178:493-502. [Crossref] [PubMed]
- Jiang J, Dai B, Peng D, Zhu C, Liu L, Lu C. Neural synchronization during face-to-face communication. J Neurosci 2012;32:16064-9. [Crossref] [PubMed]
- Cheng X, Li X, Hu Y. Synchronous brain activity during cooperative exchange depends on gender of partner: A fNIRS-based hyperscanning study. Hum Brain Mapp 2015;36:2039-48. [Crossref] [PubMed]
- Liu T, Saito H, Oi M. Role of the right inferior frontal gyrus in turn-based cooperation and competition: a near-infrared spectroscopy study. Brain Cogn 2015;99:17-23. [Crossref] [PubMed]
- Pan Y, Cheng X, Zhang Z, Li X, Hu Y. Cooperation in lovers: An f NIRS-based hyperscanning study. Hum Brain Mapp 2017;38:831-41. [Crossref] [PubMed]
- Osaka N, Minamoto T, Yaoi K, Azuma M, Shimada YM, Osaka M. How two brains make one synchronized mind in the inferior frontal cortex: fNIRS-based hyperscanning during cooperative singing. Front Psychol 2015;6:1811. [Crossref] [PubMed]
- Baker JM, Liu N, Cui X, Vrticka P, Saggar M, Hosseini SH, Reiss AL. Sex differences in neural and behavioral signatures of cooperation revealed by fNIRS hyperscanning. Sci Rep 2016;6:26492. [Crossref] [PubMed]
- Xue H, Lu K, Hao N. Cooperation makes two less-creative individuals turn into a highly-creative pair. Neuroimage 2018;172:527-37. [Crossref] [PubMed]
- Liu T, Saito G, Lin C, Saito H. Inter-brain network underlying turn-based cooperation and competition: A hyperscanning study using near-infrared spectroscopy. Sci Rep 2017;7:8684. [Crossref] [PubMed]
- Zhang M, Liu T, Pelowski M, Yu D. Gender difference in spontaneous deception: A hyperscanning study using functional near-infrared spectroscopy. Sci Rep 2017;7:7508. [Crossref] [PubMed]
- Liu N, Mok C, Witt EE, Pradhan AH, Chen JE, Reiss AL. NIRS-based hyperscanning reveals inter-brain neural synchronization during cooperative Jenga game with face-to-face communication. Front Hum Neurosci 2016;10:82. [Crossref] [PubMed]
- Stolk A, Noordzij ML, Verhagen L, Volman I, Schoffelen J-M, Oostenveld R, Hagoort P, Toni I. Cerebral coherence between communicators marks the emergence of meaning. Proc Natl Acad Sci U S A 2014;111:18183-8. [Crossref] [PubMed]
- Koike T, Tanabe HC, Okazaki S, Nakagawa E, Sasaki AT, Shimada K, Sugawara SK, Takahashi HK, Yoshihara K, Bosch-Bayard J. Neural substrates of shared attention as social memory: A hyperscanning functional magnetic resonance imaging study. Neuroimage 2016;125:401-12. [Crossref] [PubMed]
- Spiegelhalder K, Ohlendorf S, Regen W, Feige B, van Elst LT, Weiller C, Hennig J, Berger M, Tüscher O. Interindividual synchronization of brain activity during live verbal communication. Behav Brain Res 2014;258:75-9. [Crossref] [PubMed]
- King-Casas B, Tomlin D, Anen C, Camerer CF, Quartz SR, Montague PR. Getting to know you: reputation and trust in a two-person economic exchange. Science 2005;308:78-83. [Crossref] [PubMed]
- Fliessbach K, Weber B, Trautner P, Dohmen T, Sunde U, Elger CE, Falk A. Social comparison affects reward-related brain activity in the human ventral striatum. Science 2007;318:1305-8. [Crossref] [PubMed]
- Krueger F, McCabe K, Moll J, Kriegeskorte N, Zahn R, Strenziok M, Heinecke A, Grafman J. Neural correlates of trust. Proc Natl Acad Sci U S A 2007;104:20084-9. [Crossref] [PubMed]
- Shaw DJ, Czekóová K, Staněk R, Mareček R, Urbánek T, Špalek J, Kopečková L, Řezáč J, Brázdil M. A dual-fMRI investigation of the iterated Ultimatum Game reveals that reciprocal behaviour is associated with neural alignment. Sci Rep 2018;8:10896. [Crossref] [PubMed]
- Iacoboni M, Dapretto M. The mirror neuron system and the consequences of its dysfunction. Nat Rev Neurosci 2006;7:942-51. [Crossref] [PubMed]
- Frith CD, Frith U. The neural basis of mentalizing. Neuron 2006;50:531-4. [Crossref] [PubMed]
- Sanfey AG. Social decision-making: insights from game theory and neuroscience. Science 2007;318:598-602. [Crossref] [PubMed]
- Rösler F. From single-channel recordings to brain-mapping devices: the impact of electroencephalography on experimental psychology. Hist Psychol 2005;8:95. [Crossref] [PubMed]
- Zhang ZM, Wang MY, Guo X, Miao X, Zhang T, Gao D, Yuan Z. Attentional avoidance of threats in obsessive compulsive disorder: An event related potential study. Behav Res Ther 2017;97:96-104. [Crossref] [PubMed]
- Cohen MX. Where does EEG come from and what does it mean? Trends Neurosci 2017;40:208-18. [Crossref] [PubMed]
- Jackson AF, Bolger DJ. The neurophysiological bases of EEG and EEG measurement: A review for the rest of us. Psychophysiology 2014;51:1061-71. [Crossref] [PubMed]
- Wang M-Y, Lu F-M, Hu Z, Zhang J, Yuan Z. Optical mapping of prefrontal brain connectivity and activation during emotion anticipation. Behav Brain Res 2018;350:122-8. [Crossref] [PubMed]
- He Y, Wang MY, Li D, Yuan Z. Optical mapping of brain activation during the English to Chinese and Chinese to English sight translation. Biomed Opt Express 2017;8:5399-411. [Crossref] [PubMed]
- Wang MY, Zhang J, Lu FM, Xiang YT, Yuan Z. Neuroticism and conscientiousness respectively positively and negatively correlated with the network characteristic path length in dorsal lateral prefrontal cortex: A resting-state fNIRS study. Brain Behav 2018. [Crossref] [PubMed]
- Lloyd-Fox S, Blasi A, Elwell C. Illuminating the developing brain: the past, present and future of functional near infrared spectroscopy. Neurosci Biobehav Rev 2010;34:269-84. [Crossref] [PubMed]
- Begliomini C, Cavallo A, Manera V, Becchio C, Stramare R, Miotto D, Castiello U. Potential for social involvement modulates activity within the mirror and the mentalizing systems. Sci Rep 2017;7:14967. [Crossref] [PubMed]
- Saxe R. Uniquely human social cognition. Curr Opin Neurobiol 2006;16:235-9. [Crossref] [PubMed]
- Carter RM, Huettel SA. A nexus model of the temporal–parietal junction. Trends Cogn Sci 2013;17:328-36. [Crossref] [PubMed]
- Van Overwalle F, Baetens K. Understanding others' actions and goals by mirror and mentalizing systems: a meta-analysis. Neuroimage 2009;48:564-84. [Crossref] [PubMed]
- Lin X, Sai L, Yuan Z. Detecting Concealed Information with Fused Electroencephalography and Functional Near-infrared Spectroscopy. Neuroscience 2018;386:284-94. [Crossref] [PubMed]
- Chiu PH, Kayali MA, Kishida KT, Tomlin D, Klinger LG, Klinger MR, Montague PR. Self responses along cingulate cortex reveal quantitative neural phenotype for high-functioning autism. Neuron 2008;57:463-73. [Crossref] [PubMed]