Accurate puncture of targets in the lower lung lobes with respiratory motion using a teleoperated robotic system and low-dose computed tomography fluoroscopy: an experimental animal study
Introduction
Many navigation-assisted computed tomography (CT)-guided puncture systems have been developed in recent years, including robotic arm navigation, electromagnetic navigation, and optical navigation (1-3). However, the punctures performed by these systems are based on pre-scanned static images, not real-time images. Moreover, the target position changes with breathing movements and heartbeat. Freehand puncture also uses static images and relies on operator’s experience, reducing the success rate. CT fluoroscopy locates lesions accurately and in real time. However, fluoroscopy can expose physicians, radiologists, and patients to high radiation doses (RDs) (4,5). To overcome the problems related to “blind puncture” and high RDs, our team developed a teleoperated surgical robotic system that can accurately puncture lung targets during breathing in vivo under CT fluoroscopy with low RDs and minimal invasiveness. We present this article in accordance with the ARRIVE reporting checklist (available at https://qims.amegroups.com/article/view/10.21037/qims-24-843/rc).
Methods
Animal model
Ten Bama miniature pigs (Beijing Shi Chuang Century Miniature Pig Breeding Base) of both sexes (weight, 30–35 kg) were randomly and blindly assigned to one of two groups using a random number generator. Data collectors were aware of the group allocation at the different stages of the experiment. Group A received freehand punctures with conventional CT, and group B received robot-assisted punctures with real-time CT fluoroscopy. A protocol was prepared before the study without registration. The study was approved by the Animal Ethics Committee of the First Medical Center of the Chinese PLA General Hospital (approval No. 2020-X16-43), and all experiments were performed in accordance with institutional guidelines for the care and use of laboratory animals. After general anesthesia, a sterile stainless-steel 0.7×2-mm metal target was inserted in different regions of the lower lobes of the lungs of ten pigs under CT fluoroscopic guidance, totaling 20 targets. After target implantation, lung CT was performed to detect complications such as massive pneumothorax or bleeding.
Equipment
We used a Big Bore 16-slice CT scanner (Brilliance 16, Philips Medical Systems, Cleveland, OH, USA) with an 85-cm aperture. Fluoroscopy time was controlled by a radiologist using a foot pedal. RD (in mGy) was calculated using the dose length product. Punctures were performed using a 17-G 11.5-cm long co-axial introducer needle (TSK Co., Ltd., Tokyo, Japan).
The robotic system (Universal Robots Company, Odense, Denmark) had six degrees of freedom, and movement angle and speed were adjusted using a control panel (6). The teleoperated robotic system contained the following components: control host, remote control panel, operator control unit, mechanical arm, laser positioner, needle holder, monitoring system, and a foot pedal. The remote control panel gave operating instructions, including needle insertion, translation movements (horizontal and vertical planes), rotation (horizontal and vertical planes), and stop. The robotic arm was remotely controlled using a control interface. A 6-axis collaborative robotic arm (UR5e, Universal Robots) was mounted on a stable base. A needle holder was used to clamp and fix puncture needles and included a clamping arm made of nylon-6 (PA6) and a nylon fixation clamp made by 3D printing without X-ray artifacts. The robotic system had four cameras. Cameras were strategically placed in the four corners of the CT scanning bed, and images were displayed the puncture sites and the position of the animal on monitors in the CT control room (Figure 1).
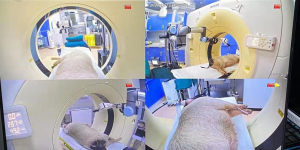
Operation method
After general anesthesia, each pig was placed on the CT table to plan the puncture path and record the position of the metal target to be punctured. A low RD (350-mm field of view, 120 KV, and 30 mA) was used in both groups based on previous animal studies. The flowchart of the procedures is shown in Figure 2. The conventional tube current was 100 mA.
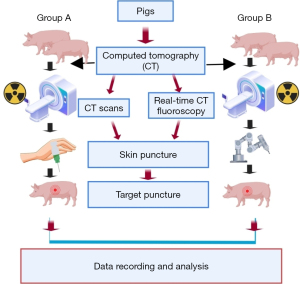
- Freehand puncture. Radiologists (attending physicians with more than 10 years of surgical experience) performed freehand punctures under conventional CT in group A (control) using a CT scanner with a slice thickness of 5 mm and a scan length of 4.0 cm in the Z axis. Ten targets were placed in the right lung, and the puncture path was determined. The puncture process is presented in Figure 3.
- Robot-assisted puncture under CT fluoroscopy. The robotic system and CT system are shown in Figure 4. The CT table was positioned at the target position. The collimator width was 6 mm. The effective RD was 66%. The needle holder was moved to the scanning plane, and the needle was fixed to the holder. The laser beam was turned on to confirm the needle position, and the position of the puncture site was estimated based on the puncture path. The needle was placed close to the puncture site using the robotic console. We used intermittent fluoroscopy, and fluoroscopy time was controlled by a radiologist by pressing the foot pedal. The alignment between the needle tip and the target was assessed under fluoroscopic guidance. The needle position and path were adjusted until the “aiming line” [a metal artifact formed by the needle during a CT fluoroscopy (6)] of the needle tip crossed the target, and the mechanical arm was lifted from the body surface in the opposite direction of the needle. The intersection of the two beams was considered the puncture site (Figure 5A).
First, we made a 2-mm incision on the skin with a scalpel, pressed the incision to prevent bleeding, and slowly moved the robotic arm to the incision. The alignment between the “aiming line” and the target was assessed in real time under fluoroscopy. Then, scans were performed continuously by pressing the foot pedal, and the spatial position of the needle tip and target was displayed in real time. We used a joystick to control the movements of the robotic arm, the needle holder and perform continuous punctures (Figure 5B). The position of the target and “aiming line” changed with respiratory motion (Figure 6A,6B). The needle path was adjusted using the joystick under fluoroscopy until the “aiming line” aligned with the target (Figure 6C). The target was reached after adjusting the needle depth (Figure 6D). - Ten punctures were performed in each puncture mode. Data on the number of needle adjustments (NA), puncture time (PT), operation time (OT), and RD were collected and analyzed. Postsurgical complications, including pneumothorax and bleeding, were evaluated. At study endpoints, all pigs were intravenous injection of 10% KCl, 15–20 mL under deep anesthesia.
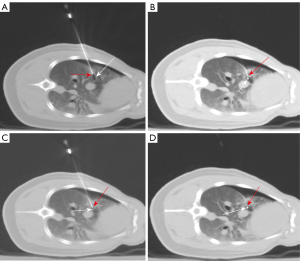
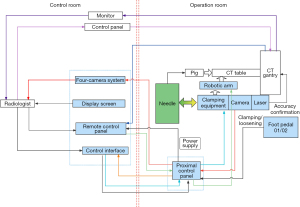
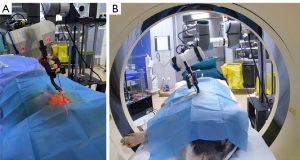
Statistical analysis
Statistical analysis was performed using SPSS version 26.0 (IBM Corp., Armonk, NY, USA). Surgical data were compared using a Mann-Whitney U test. A P value of less than 0.05 was considered statistically significant.
Results
Twenty punctures were performed and the accuracy of all punctures was 100%. There was mild bleeding in the thoracic cavity in one pig and mild pneumothorax after metal target placement in another animal. Nonetheless, these conditions did not affect the accuracy of freehand punctures (Figure 3) and robot-assisted punctures using low-dose CT (Figure 6). Surgical data are shown in Table 1.
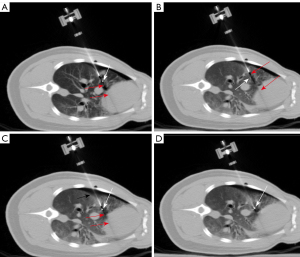
Table 1
Indicator | Group | Median (Q1–Q3) | Z | P |
---|---|---|---|---|
OT (s) | A | 655.000 (592.500–811.250) | −3.780 | 0.00016 |
B | 148.500 (131.750–157.750) | |||
NA | A | 5.000 (4.750–6.000) | −4.063 | 0.00005 |
B | 1.000 | |||
PT (s) | A | 467.500 (420.000–576.250) | −3.788 | 0.00015 |
B | 22.500 (21.500–24.500) | |||
RD (mGy) | A | 80.000 (75.500–94.400) | −1.140 | 0.25414 |
B | 90.200 (84.825–98.900) |
Group A: received freehand punctures with conventional CT; Group B: received robot-assisted punctures with real-time CT fluoroscopy. OT, operation time; NA, number of needle adjustments; PT, puncture time; RD, radiation dose; CT, computed tomography.
The results of the comparative analysis using the Mann-Whitney U test revealed significant between-group differences in OT, NA, and PT (P<0.05). However, the difference in RD was not statistically significant (P>0.05). The four surgical indicators were compared using box plots (Figure 7A-7D). The median OT, NA, and PT values were significantly higher in group A (P<0.05) (Figure 7A-7C). The median DR was similar between the groups (P>0.05) (Figure 7D). The smaller deviations of OT, NA, and PT in group B indicate that robot-assisted punctures offer greater stability than freehand punctures.
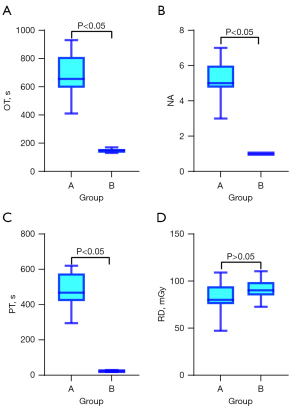
In summary, the robotic system has better OT, NA and PT than freehand puncture without increasing radiation dosage.
Discussion
Although most lung nodules are small (a few millimeters) and can be treated surgically, some patients are not eligible for surgery because of the presence of multiple nodules or poor lung function or being unwilling to undergo surgery. Therefore, minimally invasive ablations of lung nodules under CT fluoroscopy are becoming more common (7-10). However, respiratory movements limit the efficacy of the treatment of small nodules (11-14).
Freehand techniques are associated with a high number of punctures, and puncture accuracy is lower in lung regions with higher tidal volumes, such as the lower lobe, increasing the risk of tissue damage, prolonging OT, and increasing RD (15-17). Furthermore, conventional CT-guided puncture is a step-by-step process where needle position and trajectory are evaluated at several time points and adjusted accordingly if necessary.
Navigation systems can puncture targets rapidly and accurately. Nonetheless, these systems use pre-scanned images, and punctures are affected by breathing motion (14,18-21). We have developed a robotic system that uses real-time CT fluoroscopy and demonstrated the safety and high accuracy of this system. The teleoperated robot achieved the accurate puncture of targets in the lower lung lobes through target localization, real-time monitoring of respiratory motion, precise control of the robotic arm, and low-dose CT fluoroscopy.
CT is more commonly used than digital subtraction angiography (DSA) because the former has a higher resolution and accurately recognizes anatomical structures (22-25). Therefore, new strategies to reduce RD during puncture under CT guidance are warranted.
Our robotic system can perform percutaneous punctures using low-dose CT under fluoroscopic guidance while obtaining CT images in real time. The results showed that RD was similar between the two puncture modes; nonetheless, the robotic system was associated with a lower NA and shorter PT and OT.
Human phantom studies showed that a tube current of 30 mA was associated with a significantly lower RD than a current of 50 mA without decreasing the signal-to-noise ratio (6). Therefore, we used a current of 30 mA in both animal groups. Freehand punctures are performed using conventional CT; scans are short and intermittent, and the scanning range is large. During real-time CT fluoroscopy, a single-layer scan using a 6-mm collimator reduced the RD only by 34%. These results indicated that RD was similar between the two methods.
Fluoroscopy time should be minimized to reduce RD. Therefore, needle position and path should be estimated before starting fluoroscopy. We assessed the alignment between the needle tip and the target, and the needle position and trajectory were adjusted to reduce fluoroscopy time and RD. The puncture was initiated when the “aiming line” crossed the target.
Given that the target position changes during breathing and heartbeat, freehand punctures are more challenging and NA is higher in the lower lobes, which have higher tidal volumes than upper lobes, and in lung regions closer to the heart. The target position can be adjusted in real time, even in patients with pneumothorax or other complications (Figure 6A-6D), increasing puncture accuracy and efficiency.
We observed that the depth of needle insertion increased with tissue thickness. The cases in which the needle tip does not quickly and completely penetrate the pleura can lead to tissue deformation (i.e., the needle pushes against the pleura and lung tissue, Figure 6A) and deviations from the target. This effect is common in lung tissues because of their low density and the presence of air and is even more common in cases of pneumothorax. In these cases, it is necessary to plan the puncture path, manually adjust the needle holder, and increase the puncture speed when the needle is close to the parietal pleura to increase the impact force and inertia, resembling freehand puncture. In targets located in the lower lobe of the right lung with pneumothorax, after penetrating the pleura, the needle path needs to be adjusted until the “aiming line” crosses the target. The puncture angle should be almost perpendicular to the pleural surface to minimize tissue deformation. When tissue deformation is large, the needle or target may deviate from the planned trajectory, and the needle or target cannot be visualized. When the needle deviates from the trajectory, we use the joystick to adjust the direction of the needle tip to the anterior or posterior side. Needles adjusted to the planned trajectory can continue guiding punctures. The needles that cannot be visualized after repeated adjustments should be withdrawn until the tip is visible. In cases of target deviation, the target should be lifted until the tip is visible. If the target is not visible after this attempt, scans are repeated to locate the target, and puncture is resumed.
Laser locators are used in pig models to increase puncture accuracy because needles do not penetrate thick skin easily. Therefore, a small incision is made in the skin preoperatively while the needle is kept at 10 cm from the skin in the opposite direction of the puncture trajectory. The intersection of the two crossing laser beams on the skin is considered the puncture site. Compared with positioning methods based on surface markers, incisions made at this intersection increase puncture accuracy.
The robotic system is mounted on the floor beside the CT table, and this assembly is stationary (except for the robotic arm) to avoid puncturing non-target tissues.
Conclusions
In conclusion, robot-assisted punctures did not cause severe complications, indicating safety and feasibility, and were associated with decreased NA and shorter PT and OT, increasing the accuracy and efficiency of percutaneous punctures, especially in the lower lobes of the lungs with respiratory motion. These features may benefit future clinical research and applications.
Acknowledgments
Funding: This work was supported by grants from
Footnote
Reporting Checklist: The authors have completed the ARRIVE reporting checklist. Available at https://qims.amegroups.com/article/view/10.21037/qims-24-843/rc
Conflicts of Interest: All authors have completed the ICMJE uniform disclosure form (available at https://qims.amegroups.com/article/view/10.21037/qims-24-843/coif). All authors report that this work has received grant support from the National Natural Science Foundation of China (grant No. 81771941) and the Special Scientific Research Projects on Military Health Care (grant No. 22BJZ18). The authors have no other conflicts of interest to declare.
Ethical Statement: The authors are accountable for all aspects of the work in ensuring that questions related to the accuracy or integrity of any part of the work are appropriately investigated and resolved. The study was approved the Animal Ethics Committee of the First Medical Center of the Chinese PLA General Hospital (approval No. 2020-X16-43), and all experiments were performed in accordance with institutional guidelines for the care and use of laboratory animals.
Open Access Statement: This is an Open Access article distributed in accordance with the Creative Commons Attribution-NonCommercial-NoDerivs 4.0 International License (CC BY-NC-ND 4.0), which permits the non-commercial replication and distribution of the article with the strict proviso that no changes or edits are made and the original work is properly cited (including links to both the formal publication through the relevant DOI and the license). See: https://creativecommons.org/licenses/by-nc-nd/4.0/.
References
- Cleary K, Watson V, Lindisch D, Taylor RH, Fichtinger G, Xu S, White CS, Donlon J, Taylor M, Patriciu A, Mazilu D, Stoianovici D. Precision placement of instruments for minimally invasive procedures using a "needle driver" robot. Int J Med Robot 2005;1:40-7. [Crossref] [PubMed]
- He XF, Xiao YY, Zhang X, Zhang XB, Zhang X, Wei YT, Zhang ZL, Wiggermann P. Preliminary clinical application of the robot-assisted CT-guided irreversible electroporation ablation for the treatment of pancreatic head carcinoma. Int J Med Robot 2020;16:e2099. [Crossref] [PubMed]
- Koethe Y, Xu S, Velusamy G, Wood BJ, Venkatesan AM. Accuracy and efficacy of percutaneous biopsy and ablation using robotic assistance under computed tomography guidance: a phantom study. Eur Radiol 2014;24:723-30. [Crossref] [PubMed]
- Burgard CA, Dinkel J, Strobl F, Paprottka PM, Schramm N, Reiser M, Trumm CG. CT fluoroscopy-guided percutaneous osteoplasty with or without radiofrequency ablation in the treatment of painful extraspinal and spinal bone metastases: technical outcome and complications in 29 patients. Diagn Interv Radiol 2018;24:158-65. [Crossref] [PubMed]
- Yamao Y, Yamakado K, Takaki H, Yamada T, Kodama H, Nagasawa N, Nakatsuka A, Uraki J, Takeda K. CT-fluoroscopy in chest interventional radiology: sliding scale of imaging parameters based on radiation exposure dose and factors increasing radiation exposure dose. Clin Radiol 2013;68:162-6. [Crossref] [PubMed]
- He X, Xiao Y, Zhang X, Zhang X, Zhang X, Wei Y, Zhang Z, Xue X, Zhang S. Low-dose CT fluoroscopy-guided interventional minimally invasive robot. Heliyon 2024;10:e28914. [Crossref] [PubMed]
- Gits HC, Khosravi Flanigan MA, Kapplinger JD, Reisenauer JS, Eiken PW, Breen WG, et al. Sublobar Resection, Stereotactic Body Radiation Therapy, and Percutaneous Ablation Provide Comparable Outcomes for Lung Metastasis-Directed Therapy. Chest 2024;165:1247-59. [Crossref] [PubMed]
- Mankidy BJ, Mohammad G, Trinh K, Ayyappan AP, Huang Q, Bujarski S, Jafferji MS, Ghanta R, Hanania AN, Lazarus DR. High risk lung nodule: A multidisciplinary approach to diagnosis and management. Respir Med 2023;214:107277. [Crossref] [PubMed]
- Tanner NT, Porter A, Gould MK, Li XJ, Vachani A, Silvestri GA. Physician Assessment of Pretest Probability of Malignancy and Adherence With Guidelines for Pulmonary Nodule Evaluation. Chest 2017;152:263-70. [Crossref] [PubMed]
- Xu Z, Wang X, Ke H, Lyu G. Cryoablation is superior to radiofrequency ablation for the treatment of non-small cell lung cancer: A meta-analysis. Cryobiology 2023;112:104560. [Crossref] [PubMed]
- Appelbaum L, Sosna J, Nissenbaum Y, Benshtein A, Goldberg SN. Electromagnetic navigation system for CT-guided biopsy of small lesions. AJR Am J Roentgenol 2011;196:1194-200. [Crossref] [PubMed]
- Liu J, Jiang Y, He R, Cui F, Lin Y, Xu K, Cai W, Hao Z, Zeng Y, Chen X, Zhang H, Shi J, Cheong H, Dong M, U K, Huang X, Jiang S, Huang J, Wang W, Liang H, Duan X, He J. Robotic-assisted navigation system for preoperative lung nodule localization: a pilot study. Transl Lung Cancer Res 2023;12:2283-93. [Crossref] [PubMed]
- Wang J, Zhang T, Xu Y, Yang M, Huang Z, Lin J, Xie S, Sun H. Comparison between percutaneous transthoracic co-axial needle CT-guided biopsy and transbronchial lung biopsy for the diagnosis of persistent pulmonary consolidation. Insights Imaging 2023;14:80. [Crossref] [PubMed]
- Wei L, Jiang S, Yang Z, Zhang G, Ma L. A CT-guided robotic needle puncture method for lung tumours with respiratory motion. Phys Med 2020;73:48-56. [Crossref] [PubMed]
- Adiga S, Athreya S. Safety, efficacy, and feasibility of an ultra-low dose radiation protocol for CT-guided percutaneous needle biopsy of pulmonary lesions: initial experience. Clin Radiol 2014;69:709-14. [Crossref] [PubMed]
- Prosch H, Stadler A, Schilling M, Bürklin S, Eisenhuber E, Schober E, Mostbeck G. CT fluoroscopy-guided vs. multislice CT biopsy mode-guided lung biopsies: accuracy, complications and radiation dose. Eur J Radiol 2012;81:1029-33. [Crossref] [PubMed]
- Tsalafoutas IA, Tsapaki V, Triantopoulou C, Gorantonaki A, Papailiou J. CT-guided interventional procedures without CT fluoroscopy assistance: patient effective dose and absorbed dose considerations. AJR Am J Roentgenol 2007;188:1479-84. [Crossref] [PubMed]
- Atashzar SF, Khalaji I, Shahbazi M, Talasaz A, Patel RV, Naish MD. Robot-assisted lung motion compensation during needle insertion. IEEE ICRA 2013.
- Pour Arab D, Voros S, Essert C. Dynamic path planning for percutaneous procedures in the abdomen during free breathing. Int J Comput Assist Radiol Surg 2020;15:1195-203. [Crossref] [PubMed]
- Duan X, He R, Jiang Y, Cui F, Wen H, Chen X, Hao Z, Zeng Y, Liu H, Shi J, Cheong H, Dong M, U K, Jiang S, Wang W, Liang H, Liu J, He J. Robot-assisted navigation for percutaneous localization of peripheral pulmonary nodule: an in vivo swine study. Quant Imaging Med Surg 2023;13:8020-30. [Crossref] [PubMed]
- Li J, Su L, Liu J, Peng Q, Xu R, Cui W, Deng Y, Xie W, Huang B, Chen J. Optical navigation robot-assisted puncture system for accurate lung nodule biopsy: an animal study. Quant Imaging Med Surg 2023;13:7789-801. [Crossref] [PubMed]
- Boekestijn I, Azargoshasb S, van Oosterom MN, Slof LJ, Dibbets-Schneider P, Dankelman J, van Erkel AR, Rietbergen DDD, van Leeuwen FWB. Value-assessment of computer-assisted navigation strategies during percutaneous needle placement. Int J Comput Assist Radiol Surg 2022;17:1775-85. [Crossref] [PubMed]
- Fu YF, Li GC, Xu QS, Shi YB, Wang C, Wang T. Computed tomography-guided lung biopsy: a randomized controlled trial of low-dose versus standard-dose protocol. Eur Radiol 2020;30:1584-92. [Crossref] [PubMed]
- Grasso RF, Cazzato RL, Luppi G, D'Agostino F, Schena E, Del Vescovo R, Giurazza F, Faiella E, Beomonte Zobel B. Percutaneous lung biopsies: performance of an optical CT-based navigation system with a low-dose protocol. Eur Radiol 2013;23:3071-6. [Crossref] [PubMed]
- Zhang HM, Huo XB, Wang HL, Zhang X, Fu YF. Computed tomography-guided cutting needle biopsy for lung nodules: A comparative study between low-dose and standard dose protocols. Medicine (Baltimore) 2021;100:e24001. [Crossref] [PubMed]