Application of conventional ultrasound coupled with shear wave elastography in the assessment of muscle strength in patients with type 2 diabetes
Introduction
Alterations in skeletal muscle in individuals with type 2 diabetes mellitus (T2DM), in addition to the traditional macro- and microvascular complications, have been the focus of research in recent years. Sarcopenia is an age-related progressive loss of skeletal muscle mass and strength (1). The presence of diabetes and its related complications can accelerate the development of sarcopenia (2). For example, decreased mitochondrial function induces changes in muscle fiber composition and the transition from an oxidative to glycolytic fiber type, which leads to sarcopenia (3). Another mechanism that contributes to sarcopenia in diabetes is diabetic peripheral neuropathy (DPN) and reduction in motor neurons (4), which can cause muscle weakness, atrophy, and adipose tissue infiltration. Sarcopenia is emerging as one of the crucial complications of diabetes. However, when patients with diabetes are considered as a whole, those patients with decreased muscle strength have greater leg muscle mass than do those without diabetes because of differences in body size (5). Therefore, decreased muscle strength may be more informative than loss of muscle mass in the prognosis of patients with diabetes combined with sarcopenia (6).
Patients with diabetes are more likely to experience decreased skeletal muscle strength over time than are age-matched controls, especially in the lower extremities (7,8). Maintaining or increasing muscle mass does not prevent a decrease in muscle strength (9). Moreover, physical disability is likely to occur as a consequence of falls in patients with diabetes, which is attributed to the increased risk of infection and slow healing (10,11). Resistance training exercises have been confirmed to be effective methods for improving muscle strength and lowering the risk of falls (12). Thus, the investigation of the muscle function in patients with diabetes may be critical for early intervention and for preventing injurious falls.
Leg muscle strength can be directly evaluated by measuring various muscle indicators, such as mass, size, stiffness, and contractile characteristics. Lower extremity muscle strength can be measured with isokinetic muscle testing, which has certain limitations, such as the requirement of special equipment and a patient with an amenable health condition. Grip strength correlates with leg strength, but it only indirectly reflects the overall condition of the leg muscle group. Lower extremity muscle strength can also be indirectly assessed using different functional tests (13,14) and function questionnaires (15). These methods can only reveal the comprehensive functional changes in older adult individuals and do not indicate the specific muscle function. Hence, by quantifying the elasticity of the musculoskeletal structures, ultrasound techniques have been developed to acquire not only morphological data but also biomechanical characteristics.
Muscle morphological parameters such as thickness, pennation angle, fiber length, and cross-sectional area (CSA) can be used to evaluate the structural and functional states of skeletal muscles (16). However, for individuals with diabetes, before any observable changes in muscle morphology can be measured, the imbalance of muscle protein metabolism, accumulation of glycation end products, and the alteration of muscle composition via intermuscular adipose tissue (IMAT) infiltration changing in the tissue’s mechanical properties can ultimately cause changes in muscle stiffness (4,17). Currently, determining the changes in skeletal muscle mainly depends on invasive biopsy. Thus, a simple, repeatable, and noninvasive imaging technique for muscle imaging is needed in clinic. Shear wave elastography (SWE) is a relatively new and widely used ultrasound-based technique (either assessed in kilopascal or meters per second) based on the principle of detecting changes in tissue stiffness caused by specific pathophysiological processes such as inflammation, aging, and malignant masses (18). As research into SWE for muscle biomechanical applications continues to progress, SWE is proving to be a potential viable approach for measuring muscle functional characteristics (19,20).
Muscle force is induced by changes in muscle elasticity. Therefore, muscle stiffness is an indicator of muscle biomechanics that can indirectly reflect the changes in muscle composition. Shear wave velocity (SWV) has been proven to correlated with muscle strength (21) and functional tests (22) in healthy populations, indicating the potential value of SWE in reflecting the structural and functional state of muscles. In disease states, reduced muscle stiffness detected using SWE is associated with muscle weakness (23,24). Variations in muscle stiffness may also reflect muscle edema and muscle atrophy. The relatively simple SWE measurement can be used to assess the status of skeletal muscles and monitor the changes in contractile properties over time as diseases progress and resolve. However, it remains unclear whether SWE, a convenient and quantitative method, can be used to detect changes in muscle stiffness before observable muscle morphology changes in patients with diabetes.
The SWE technique may provide a new perspective on the biomechanical status of diabetic muscles. Related studies (25,26) have chiefly focused on the ultrasonic features of skeletal muscle in those with T2DM. We thus aimed to further investigate the correlations between muscle function and the characteristics of muscle size and stiffness as measured via ultrasound to explore its clinical relevance. We hypothesized that (I) ultrasound can detect the altered muscle stiffness and size in patients with diabetes as compared to healthy controls and that (II) these findings are associated with isokinetic muscle strength and physical performance. We present this article in accordance with the STROBE reporting checklist (available at https://qims.amegroups.com/article/view/10.21037/qims-23-1152/rc).
Methods
Study design
Flowchart of participant selection is shown in Figure S1. In this cross-sectional study, 60 patients diagnosed with T2DM were recruited by endocrinologists from the Department of Endocrinology at The Third Affiliated Hospital of Southern Medical University between September 2021 and September 2022. Diabetes was defined as a fasting plasma glucose level of at least 126 mg/dL (≥6.1 mmol/L) as per the American Diabetes Association criteria (27). The exclusion criteria were a history of injury or operation of the lower limb, a history or clinical signs of neuromuscular disorders, any muscle-induced disease, and the presence of other types of diabetes mellitus. Additionally, 35 healthy sex-, age-, height-, and weight-matched normoglycemic controls were recruited so that the muscle characteristics of controls could be compared to those of patients with T2DM. This study was approved by the ethics committee of The Third Affiliated Hospital of Southern Medical University (approval No. 202224) and was conducted in accordance with the Declaration of Helsinki (as revised in 2013). Informed consent was obtained from all participants.
Demographic and clinical information
Sociodemographic characteristics, including age, height, weight, and sex, were collected. Participants completed the Strength, Assistance with Walking, Rising from a Chair, Climbing Stairs, and Falls (SARC-F) questionnaire (15), which is an simple screen directly related to comprehensive muscle function (see details in Table S1) based on five domains. Thigh circumference was measured at the lower third of the distance between the anterior superior iliac spine and condylus medialis femoris. The calf circumference was measured in the thickest section.
DPN is a common complication of diabetes. To further examine the effect of DPN, all patients were examined for characteristic sign and symptoms by a trained neurologist with at least 10 years of experience in determining the presence of DPN. The diagnostic criteria for DPN were based on the 2017 diagnostic methods proposed by the American Diabetes Association. The detailed methods are described elsewhere (28).
Muscle strength assessments
Bilateral knee extension strength was quantitatively evaluated in all participants by an experienced physical specialist with at least 3 years of experience in motor function assessment. The instrument used was an IsoMed 2000 isokinetic dynamometer (D&R Ferstl GmbH, Hemau, Germany), which has high reliability for the knee test in both neuropathic and healthy individuals (29,30). The detailed measurement methods are based on a previous study (21). A mean of 10 repeated sets, the peak torque [PT; in Newton meters (Nm)], the PT to body weight ratio (PT/BW, in Nm/kg), and total work (TW; in J) were selected to represent muscle strength, relative muscle strength between different populations, and muscle function, respectively.
A Jamar Plus+ dynamometer (Patterson Medical, Warrenville, IL, USA), which is the most reliable and widely used grip-strength testing instrument (31), was used three times in each hand to acquire data on maximal grip strength.
Functional performance measures
All participants underwent four functional tests (13,14) performed by the same physical therapist to assess lower extremity physical performance: the short physical performance battery (SPPB), the timed up-and-go test (TUGT), the 30-s chair stand test (CST), and the 6-meter walk test (6MWT). For the SPPB, all participants were evaluated using the standing balance test, the 2.44-m walking test, and the five-time sit-to-stand test. For the TUGT, participants were instructed to stand up from a chair, walk 3 m, return to the chair, and sit down, and the time required was recorded using a stopwatch. For the CST, the maximum number of sit-to-stand movements without use of arms was measured within 30 s. For the 6MWT, participants were instructed to walk 6 m at their normal pace twice and the average speed was recorded.
Ultrasound procedures
All ultrasound images were acquired using a LOGIQ E10 ultrasound device (GE HealthCare, Chicago, IL, USA) equipped with a linear transducer (ML6-15). Via palpation, a line was drawn between the anterior superior iliac spine and the superior margin of the condylus medialis femoris with participant in the supine position (Figure 1A). The site was marked at the lower third of the line, and the probed was moved parallel along the red line perpendicular to the long axis of the limb to locate the quadriceps femoris during the subsequent measurements.
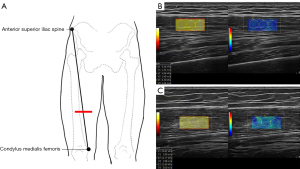
The thickness and CSA of the rectus femoris (RF) and the thickness of the anterior quadriceps (AQ) were measured in the transverse section using B-mode ultrasound at the thickest part. The probe was rotated and aligned in the direction of the muscle fascicles (32). Muscle stiffness was measured in the longitudinal direction using SWE mode for the knee extensors [RF, vastus intermedius (VI), vastus medialis (VM), and vastus lateralis (VL)]. A color-coded box superimposed on a gray-scale image was used to represent the region of interest (ROI), with focal penetration defects or obvious fibrous septa being avoided. To avoid artifacts in the circular ROIs, three 5-mm-diameter circles were placed in the ROI for quantitative analysis (Figure 1B,1C). The values were recorded as SWV in elasticity mode. These data acquisition methods have been previously validated (21,33,34). The same SWV measurement was applied to all the participants. The average SWV values for each muscle were calculated for further analysis.
Finally, for measurement of muscle stiffness of the biceps brachii (BB), the participants were asked to flex the elbow at 90° with their forearm resting on their body and their hand in supination while the probe was positioned at the middle portion of the muscle belly where the largest muscle bundle was located.
To demonstrate the reproducibility of conventional ultrasound and SWE, two practiced operators with at least 3 years of experience each in musculoskeletal ultrasound scanning adopted the same method to obtain ultrasonic images.
Statistical analysis
The baseline demographics and measured variables of the participants are expressed using descriptive statistics. Continuous variables are presented as the mean ± standard deviation. Interclass correlation coefficient (ICC) analysis was performed on the measurement data obtained by the two operators, with ICC >0.75 being considered to indicate high confidence (35). The independent samples t-test (Student t-test) was used to compare the demographics and measured variables between patients with diabetes and healthy controls. Comparisons of the demographics and measured variables among patients with or without DPN and healthy controls were performed using analysis of variance (ANOVA), with the Bonferroni method being used for multiple comparisons.
Additionally, Pearson’s correlation coefficients were used to determine the relationships between various variables. A portion of the data (1.4%) were missing due to reasons such as the poor compliance of patients. Further data processing for missing data was not performed. All tests were two-sided, and the analyses were carried out using the SPSS 25.0 (IBM Corp., Armonk, NY, USA) and R software version 4.1.1 (http://cran.r-project.org). A t value was considered significant at α=0.05.
Results
Demographic and clinical information
The baseline characteristics of the 60 patients with T2DM (32 male and 28 female) and 35 healthy controls (17 male and 18 female) are summarized in Table 1. Patients with T2DM and healthy controls were matched in terms of age (P=0.294), weight (P=0.574), height (P=0.617), and body mass index (BMI) (P=0.732) but not in terms of the thigh circumference (P=0.011) or calf circumference (P=0.031), which were smaller in patients with T2DM.
Table 1
Characteristics | Patients with diabetes (n=60) | Healthy controls (n=35) | 95% CI of mean difference | t value | P value |
---|---|---|---|---|---|
Age (years) | 57.35±9.91 | 55.23±9.16 | 2.21 (−1.88, 6.12) | 1.056 | 0.294 |
Duration of diabetes (years) | 6.76±6.38 | – | – | – | – |
Female (%) | 46.67 | 51.43 | – | 0.201 | 0.654 |
Height (cm) | 161.71±7.77 | 162.51±7.38 | −0.80 (−3.99, 2.39) | −0.502 | 0.617 |
Weight (kg) | 63.2±13.35 | 64.65±11.34 | −1.45 (−6.58, 3.67) | −0.564 | 0.574 |
BMI (kg/m2) | 24.05±4.20 | 24.33±2.96 | −0.28 (−1.88, 1.33) | −0.343 | 0.732 |
Thigh circumference (cm) | 43.69±4.27 | 45.69±3.21 | −2.00 (−3.54, −0.46) | −2.589 | 0.011* |
Calf circumference (cm) | 34.28±3.26 | 35.71±2.91 | −1.42 (−2.71, −0.14) | −2.202 | 0.031* |
The results are expressed as the mean ± standard deviation, percentage, 95% CI of the mean difference, t value, and corresponding P value. *, P<0.05 indicates a significant difference. CI, confidence interval; BMI, body mass index.
According to the presence or absence of DPN, all patients with T2DM were divided into two groups: with DPN (n=26) and without DPN (n=34). There was no statistically significant difference in terms of age (P=0.063), weight (P=0.596), height (P=0.727), or BMI (P=0.326) between the three groups (see details in Table S2).
Clinical and muscle strength assessments
Table 2 shows the difference in physical performance and isokinetic muscle testing results between patients with diabetes and healthy controls. Patients with T2DM exhibited worse general physical performance than did their nondiabetic counterparts, as evidenced by significantly higher SARC-F scores (P=0.007) and lower SPPB scores (P<0.001). In addition, the results of the 6WMT, CST, and TUGT test indicated respectively slower walking speed (P=0.004), worse muscle endurance (P<0.001), and worse coordination and balance (P<0.001) in patients with T2DM. In line with the findings for physical performance findings, the PT (P=0.004), PT/BW (P<0.001), and TW (P=0.001) of the quadriceps femoris were greater in healthy controls than in patients with T2DM pointing to a decline in lower-limb muscle strength. In contrast, grip strength showed no significant difference between the two groups (P=0.102). In multiple comparisons, patients with DPN had weaker knee muscle strength (PT: P=0.041; PT/BW: P=0.036; TW: P=0.022) than did those without DPN. However, no statistically significant difference in leg muscle strength (PT: P=0.396; PT/BW: P=0.114; TW: P=0.272) was observed between patients without DPN and healthy controls (see details in Table S3).
Table 2
Characteristics | Patients with diabetes (n=60) | Healthy controls (n=35) | 95% CI of mean difference | t value | P value |
---|---|---|---|---|---|
Clinical and muscle assessments | |||||
CST | 34.38±10.26 | 46.37±11.77 | −11.99 (−16.75, −7.21) | −5.015 | <0.001* |
TUGT (s) | 10.03±2.11 | 8.67±1.39 | 1.36 (0.64, 2.07) | 3.766 | <0.001* |
6MWT (m/s) | 1.07±0.19 | 1.19±0.17 | −0.11 (−0.19, −0.04) | −2.949 | 0.004* |
SPPB | 11.12±1.19 | 11.89±0.47 | −0.77 (−1.11, −0.42) | −4.431 | <0.001* |
Handgrip strength (kg) | 30.66±9.90 | 34.05±9.45 | −3.38 (−7.46, 0.69) | −1.655 | 0.102 |
SARC-F | 0.28±0.56 | 0.06±0.24 | 0.23 (0.06, 0.39) | 2.759 | 0.007* |
Isokinetic muscle testing | |||||
PT | 77.68±31.03 | 97.14±30.44 | −19.46 (−32.46, −6.46) | −2.985 | 0.004* |
PT/BW | 1.22±0.36 | 1.49±0.33 | −0.27 (−0.42, −0.13) | −3.742 | <0.001* |
TW | 614.77±257.84 | 791.76±247.52 | −176.99 (−283.53, −70.45) | −3.310 | 0.001* |
The results are expressed as the mean ± standard deviation, 95% CI of the mean difference, t value, and the corresponding P value. *, P<0.05 indicates a significant difference. CI, confidence interval; CST, 30-s chair stand test; TUGT, timed up-and-go test; 6MWT, 6-meter walk test; SPPB, short physical performance battery; SARC-F, Strength, Assistance with Walking, Rising from a Chair, Climbing Stairs, and Falls; PT, peak torque; PT/BW, peak torque to body weight ratio; TW, total work.
Ultrasonic results
There were statistically significant differences between patients with T2DM and controls in RF thickness (10.69±3.21 vs. 13.09±2.41 mm, respectively; P<0.001), AQ thickness (23.45±7.11 vs. 27.25±5.25 mm, respectively; P=0.004), and RF-CSA (3.04±1.10 vs. 4.11±0.95 cm2, respectively; P<0.001).
SWE exhibited good test-retest reliability of repeated measurements, with ICCs between 0.827 and 0.976 (P<0.001) (see details in Table S4).
As shown in Figure 2, the SWV was significantly lower in the case group (RF: 1.66±0.23 m/s; VL: 1.38±0.19 m/s; VM: 1.34±0.15 m/s) than in the control group (RF: 1.83±0.18 m/s; VL: 1.53±0.19 m/s; VM: 1.51±0.16 m/s) (all P values <0.001). This suggested that muscle stiffness at rest was reduced in patients with T2DM compared with healthy controls. In contrast, patients with T2DM and healthy controls were not significantly different in terms of VI (2.59±0.34 vs. 2.52±0.23 m/s, respectively; P=0.299) or BB (3.48±0.38 vs. 3.61±0.61 m/s, respectively; P=0.257). Because the quadriceps femoris serves as a muscle group with a consistent knee extension function, the average of the SWV of each muscle forming the quadriceps femoris (RF, VI, VM and VL) (AVER) was calculated for further analysis and was lower in case group than in the control group (1.73±0.17 vs. 1.84±0.12, respectively; P<0.001). Finally, no statistically significant differences were observed in muscle stiffness between patients with and without DPN (RF: P>0.99; VM: P=0.195; VL: P=0.061; AVER: P=0.749), although there were statistically significant differences between those without DPN and control participants (RF: P=0.008; VM: P=0.001; AVER: P=0.031) but not for VL (P=0.086).

Correlation of ultrasonic parameters with performance tests and muscle strength
Correlation analysis (Figure 3) indicated that a higher number of chair stands (RF thickness: r=0.58, P<0.001; AQ thickness: r=0.45, P<0.001; RF-CSA: r=0.48, P<0.001) and higher SPPB scores (RF thickness: r=0.47, P<0.001; AQ thickness: r=0.40, P<0.001; RF-CSA: r=0.43, P<0.001) were correlated with greater muscle size. In contrast, a higher SARC-F score (RF thickness: r=−0.39, P<0.001; AQ thickness: r=−0.39, P<0.001; RF-CSA: r=−0.35, P<0.001) was associated with smaller muscle size. In addition, number of chair stands and SPPB scores were positively correlated with the SWV values for the RF, VL, and VM, with correlation coefficients between 0.21 and 0.37 (all P values <0.05) indicating a poor correlation. The AQ thickness (r=0.13, P=0.196) and the SWV values of the RF (r=0.12, P=0.261) and VL (r=0.15, P=0.144) had no significant correlation with walking speed.
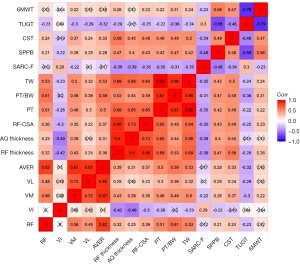
In addition, positive correlations were observed between all ultrasonic parameters except for VI and the isokinetic muscle testing (PT, TW, and PT/BW) (Figure 3). Specifically, muscle sizes showed positive correlations with leg extension strength, and the correlation coefficients were between 0.44 and 0.69 (both P values <0.001). The SWVs of the RF, VL, and VM, along with the AVER were significantly positively correlated with muscle strength, with the correlation coefficients of these associations being between 0.30 and 0.61 (P<0.05); however, this relationship was not observed for VI (PT: r=−0.26, P=0.012; PT/BW: r=−0.20, P=0.058; TW: r=−0.23, P=0.027). Finally, in the Pearson correlation coefficient analyses, all isokinetic testing results were correlated with the functional tests (P<0.05).
Discussion
This study demonstrated that SWE is a repeatable technique between examiners for the assessment of muscle stiffness. The results of this preliminary study indicated that patients had a lower muscle stiffness (i.e., lower SWV) than did healthy controls, which confirms our hypothesis of altered muscle stiffness being present in those with diabetes. Moreover, SWV values were correlated with muscle strength and functional performance.
Clinical and muscle strength assessments
Muscle strength assessment is critical for the management of patients with neuromuscular disorders because it reflects the impact of chronic conditions, disease progression, and physiological decline (10,36,37). In agreement with a previous study (7), our findings included decreased leg muscle strength in patients with T2DM compared with healthy controls. As a major antigravity muscle group, the knee extensors contribute significantly to body support and control during gait. Activities of daily living, such as walking, climbing stairs, and standing up from a chair, become difficult when the knee extension strength is below a certain level. Decreased muscle strength and functional mobility can result in physical impairment and poor quality of life in patients with diabetes (38). Our findings are consistent with those of previous studies (8,39) that reported a decline in leg muscle function might indeed be the result of mobility limitation in patients with T2DM.
Most studies on this subject have focused on assessing lower-extremity muscle strength. A case-control study indicated that grip strength was significantly lower in patients with diabetes than in normoglycemic controls (38), whereas other studies have shown that strength at the elbow and wrist is preserved (8). In our study, although patients exhibited decreased lower limb muscle strength, handgrip strength was comparable, suggesting that such antigravity muscles, such as the quadriceps femoris, are likely to be most affected by early alterations in muscular function as compared to the upper limb muscles. The impaired motor capacity of the lower extremities is attributable to the process of distal neuropathy, and this altered muscle function may be correlated with DPN; however, more studies are needed to confirm this relationship.
Morphological changes in skeletal muscle
Muscle strength is correlated with muscle volume, which is reflected by muscle CSA and thickness. Muscle thickness measured by ultrasonography is correlated with muscle mass evaluated by the gold standard, dual-energy X-ray absorptiometry (40). In this study, patients with diabetes exhibited smaller muscle thickness and CSA than did normal controls, indicating the deterioration of muscle mass. Loss of muscle mass could lead to metabolic disorder in those with T2DM because skeletal muscle figures prominently in regulating blood glucose (41). Ultrasound can be used to evaluate changes in muscle mass, and it is highly reproducible (r=0.96–0.99) (42,43). Wang et al. (44) reported that older adults with a gastrocnemius muscle thickness less than 1.5 cm can be considered to have low skeletal muscle mass. Future studies should be focused on the cutoff value of ultrasound-measured muscle thickness for low muscle mass in patients with diabetes.
Loss of muscle mass leads to a loss of muscle strength. Ata et al. (45) reported that RF muscle thickness can be used to predict gait speed or physical performance. Our study focused on both muscle strength and functional performance in patients with diabetes and showed that muscle thickness reflects not only physical mobility but also limb muscle strength. We found that smaller muscle size was moderately associated with weaker muscle strength and poorer physical performance, emphasizing that muscle atrophy may be related to muscle weakness, which is in line with other reports (46,47).
Elastic changes in skeletal muscle
Given that early muscle microstructural changes related to atrophy may be accompanied by abnormal elastography findings, we investigated the potential application use of SWE in patients with diabetes. As expected and consistent with the literature (48), we found that SWE served as a repeatable technique for the assessment of muscle stiffness and may thus be the preferred method for clinical measurement. The results of another preliminary study showed that diabetes may cause reduced stiffness in the leg muscles, which could serve as a diagnostic indicator in clinical settings. Reduced muscle stiffness is partly attributable to an increase in IMAT in the skeletal muscle of patients with diabetes. In general, muscles are not infiltrated by adipose tissue, but IMAT levels are higher in patients with T2DM and metabolic syndrome than in those without these conditions, and this is associated with insulin resistance and decreased physical function with age (49).
Our study also demonstrated that the softer the muscle stiffness in a relaxed position is, the poorer the motor ability of the quadriceps femoris. The decrease in the SWV of the quadriceps femoris reflected impaired muscle mechanical characteristics; that is, the deterioration of the tissue’s elastic properties was associated with the functional performance of the patients. This is because the increased IMAT content in the skeletal muscle is inextricably linked to decreased muscle mass and strength in the T2DM population (50). Skeletal muscles are composed of contractile components (actin and myosin) and passive elastic components (connective tissue) (51). The amount of fat infiltrating the muscle may alter its components. Decreased muscle stiffness results in impaired dynamic joint stability (52) and decreased explosive strength (19), leading to a decline in physical function. In addition, the decrease in SWV may be the result of the metabolic impairment of skeletal muscle, as IMAT is associated with insulin resistance (53).
The use of gray-scale sonography in the period of muscle microstructural changes may not be able to detect subtle abnormalities in muscle components. Resting muscle elasticity in the form of SWV is closely associated with patients’ motor activities and could provide useful information regarding the early decline in muscular function. However, this study did not perform a muscle biopsy to assess the fat content of the muscle tissue, and future studies could further investigate the relationship between the elasticity values measured using SWE and the fat content of the muscle tissue.
Effect of DPN on skeletal muscle
To further investigate the effect of DPN, patients were divided into two groups based on the presence or absence of DPN. Ijzerman et al. (54) demonstrated the additive negative effect of DPN in aggravating the decline of muscular strength in patients with neuropathy, which is consistent with our findings.
Loss of knee extension strength has been thought to be related to muscular atrophy (55); however, no measurable muscle atrophy was observed between patients with and without DPN in our study. This finding may be in partly due to intramuscular fat accumulation in diabetic muscles, which may mask muscle atrophy. Patients with DPN had higher IMAT volume which has been shown to be associated with poor muscle strength and function (53). Moreover, the positive muscle weakening in patients with DPN coupled with a lack of abnormal SWV may be explained by an insufficient number of patients in the subgroup analysis. Further research should recruit more participants and conduct electrophysiological studies for the definitive diagnosis of diabetic neuropathy.
Applications of SWE in skeletal muscle
Compared to conventional muscle ultrasound, SWE has some limitations in applicability due methodological issues. In this study, we acquired ultrasound information in the supine position because SWV consistently demonstrates lower variability in relaxed positions than in stretched conditions (56). Moreover, the greater the measurement depth is, the higher the SWE measurement variability will be, and bone-proximity artifacts can also impact the measurements (57). This might explain why the SWV value of the VI was different from those of the other three muscles of the quadriceps femoris.
Therefore, the quadriceps femoris was chosen as a representative object for the study of muscle function, especially as it did not require special positioning. The quadriceps femoris served as a muscle group with consistent knee extension function, but the RF muscle displayed a significant SWV difference between the groups, which was related to a reduction in muscle strength. This was consistent with the findings obtained from the calculation of the SWV averages for the quadriceps. Therefore, we suggest that future SWE studies in patients with diabetes should adopt the RF as a representative muscle to minimize data acquisition time.
Limitations
This study has several limitations that should be mentioned. First, skeletal muscle plays an important role in glucose uptake, and metabolic disorders can be associated with muscle impairment in patients with diabetes (36); however, we did not examine the metabolic status in this study. Second, we did not investigate the effect of other complications such as peripheral arterial disease, which has been proven to be related to decreased muscular function in the lower extremities. Nonetheless, we found that the results obtained from conventional ultrasound and SWE were consistent. Therefore, future studies should recruit a sufficiently large sample of participants and observe disease duration to determine the potential value of SWE measurement conducted before changes in muscle morphology occur.
Conclusions
Our findings demonstrate that ultrasound can be used to evaluate muscle mass and strength in patients with diabetes. Decreased thigh muscle stiffness was correlated with diminished lower-extremity strength and functional impairment. However, our results did not support the negative effect of DPN on muscle stiffness. Quantitative SWE measurement can serve as convenient means for detecting biomechanical alterations in diseased muscles. Further studies in large populations with diabetes and long-term observations are warranted to confirm the application of SWE in this context.
Acknowledgments
Funding: This study was funded by
Footnote
Reporting Checklist: The authors have completed the STROBE reporting checklist. Available at https://qims.amegroups.com/article/view/10.21037/qims-23-1152/rc
Conflicts of Interest: All authors have completed the ICMJE uniform disclosure form (available at https://qims.amegroups.com/article/view/10.21037/qims-23-1152/coif). The authors have no conflicts of interest to declare.
Ethical Statement: The authors are accountable for all aspects of the work in ensuring that questions related to the accuracy or integrity of any part of the work are appropriately investigated and resolved. The study was approved by the Ethics Committee of The Third Affiliated Hospital of Southern Medical University (approval No. 202224) and was conducted in accordance with the Declaration of Helsinki (as revised in 2013). Informed consent was obtained from all participants.
Open Access Statement: This is an Open Access article distributed in accordance with the Creative Commons Attribution-NonCommercial-NoDerivs 4.0 International License (CC BY-NC-ND 4.0), which permits the non-commercial replication and distribution of the article with the strict proviso that no changes or edits are made and the original work is properly cited (including links to both the formal publication through the relevant DOI and the license). See: https://creativecommons.org/licenses/by-nc-nd/4.0/.
References
- Chen LK, Woo J, Assantachai P, Auyeung TW, Chou MY, Iijima K, et al. Asian Working Group for Sarcopenia: 2019 Consensus Update on Sarcopenia Diagnosis and Treatment. J Am Med Dir Assoc 2020;21:300-307.e2. [Crossref] [PubMed]
- Wang T, Feng X, Zhou J, Gong H, Xia S, Wei Q, Hu X, Tao R, Li L, Qian F, Yu L. Type 2 diabetes mellitus is associated with increased risks of sarcopenia and pre-sarcopenia in Chinese elderly. Sci Rep 2016;6:38937. [Crossref] [PubMed]
- Bassi-Dibai D, Santos-de-Araújo AD, Dibai-Filho AV, de Azevedo LFS, Goulart CDL, Luz GCP, Burke PR, Garcia-Araújo AS, Borghi-Silva A. Rehabilitation of Individuals With Diabetes Mellitus: Focus on Diabetic Myopathy. Front Endocrinol (Lausanne) 2022;13:869921. [Crossref] [PubMed]
- Sinclair AJ, Abdelhafiz AH, Rodríguez-Mañas L. Frailty and sarcopenia - newly emerging and high impact complications of diabetes. J Diabetes Complications 2017;31:1465-73. [Crossref] [PubMed]
- Park SW, Goodpaster BH, Strotmeyer ES, de Rekeneire N, Harris TB, Schwartz AV, Tylavsky FA, Newman AB. Decreased muscle strength and quality in older adults with type 2 diabetes: the health, aging, and body composition study. Diabetes 2006;55:1813-8. [Crossref] [PubMed]
- Newman AB, Kupelian V, Visser M, Simonsick EM, Goodpaster BH, Kritchevsky SB, Tylavsky FA, Rubin SM, Harris TB. Strength, but not muscle mass, is associated with mortality in the health, aging and body composition study cohort. J Gerontol A Biol Sci Med Sci 2006;61:72-7. [Crossref] [PubMed]
- Park SW, Goodpaster BH, Strotmeyer ES, Kuller LH, Broudeau R, Kammerer C, de Rekeneire N, Harris TB, Schwartz AV, Tylavsky FA, Cho YW, Newman AB. Health, Aging, and Body Composition Study. Accelerated loss of skeletal muscle strength in older adults with type 2 diabetes: the health, aging, and body composition study. Diabetes Care 2007;30:1507-12. [Crossref] [PubMed]
- Andersen H, Nielsen S, Mogensen CE, Jakobsen J. Muscle strength in type 2 diabetes. Diabetes 2004;53:1543-8. [Crossref] [PubMed]
- Goodpaster BH, Park SW, Harris TB, Kritchevsky SB, Nevitt M, Schwartz AV, Simonsick EM, Tylavsky FA, Visser M, Newman AB. The loss of skeletal muscle strength, mass, and quality in older adults: the health, aging and body composition study. J Gerontol A Biol Sci Med Sci 2006;61:1059-64. [Crossref] [PubMed]
- Macgilchrist C, Paul L, Ellis BM, Howe TE, Kennon B, Godwin J. Lower-limb risk factors for falls in people with diabetes mellitus. Diabet Med 2010;27:162-8. [Crossref] [PubMed]
- Schwartz AV, Hillier TA, Sellmeyer DE, Resnick HE, Gregg E, Ensrud KE, Schreiner PJ, Margolis KL, Cauley JA, Nevitt MC, Black DM, Cummings SR. Older women with diabetes have a higher risk of falls: a prospective study. Diabetes Care 2002;25:1749-54. [Crossref] [PubMed]
- Egger A, Niederseer D, Diem G, Finkenzeller T, Ledl-Kurkowski E, Forstner R, Pirich C, Patsch W, Weitgasser R, Niebauer J. Different types of resistance training in type 2 diabetes mellitus: effects on glycaemic control, muscle mass and strength. Eur J Prev Cardiol 2013;20:1051-60. [Crossref] [PubMed]
- Cruz-Jentoft AJ, Baeyens JP, Bauer JM, Boirie Y, Cederholm T, Landi F, Martin FC, Michel JP, Rolland Y, Schneider SM, Topinková E, Vandewoude M, Zamboni MEuropean Working Group on Sarcopenia in Older People. Sarcopenia: European consensus on definition and diagnosis: Report of the European Working Group on Sarcopenia in Older People. Age Ageing 2010;39:412-23. [Crossref] [PubMed]
- Sawada S, Ozaki H, Natsume T, Deng P, Yoshihara T, Nakagata T, Osawa T, Ishihara Y, Kitada T, Kimura K, Sato N, Machida S, Naito H. The 30-s chair stand test can be a useful tool for screening sarcopenia in elderly Japanese participants. BMC Musculoskelet Disord 2021;22:639. [Crossref] [PubMed]
- Malmstrom TK, Morley JE. SARC-F: a simple questionnaire to rapidly diagnose sarcopenia. J Am Med Dir Assoc 2013;14:531-2. [Crossref] [PubMed]
- El-Ansary D, Marshall CJ, Farragher J, Annoni R, Schwank A, McFarlane J, Bryant A, Han J, Webster M, Zito G, Parry S, Pranata A. Architectural anatomy of the quadriceps and the relationship with muscle strength: An observational study utilising real-time ultrasound in healthy adults. J Anat 2021;239:847-55. [Crossref] [PubMed]
- Jang HC. Sarcopenia, Frailty, and Diabetes in Older Adults. Diabetes Metab J 2016;40:182-9. [Crossref] [PubMed]
- Ophir J, Céspedes I, Ponnekanti H, Yazdi Y, Li X. Elastography: a quantitative method for imaging the elasticity of biological tissues. Ultrason Imaging 1991;13:111-34. [Crossref] [PubMed]
- Ando R, Suzuki Y. Positive relationship between passive muscle stiffness and rapid force production. Hum Mov Sci 2019;66:285-91. [Crossref] [PubMed]
- Soldos P, Besenyi Z, Hideghéty K, Pávics L, Hegedűs Á, Rácz L, Kopper B. Comparison of Shear Wave Elastography and Dynamometer Test in Muscle Tissue Characterization for Potential Medical and Sport Application. Pathol Oncol Res 2021;27:1609798. [Crossref] [PubMed]
- Tang X, Wang L, Guo R, Huang S, Tang Y, Qiu L. Application of ultrasound elastography in the evaluation of muscle strength in a healthy population. Quant Imaging Med Surg 2020;10:1961-72. [Crossref] [PubMed]
- Pang J, Wu M, Liu X, Gao K, Liu Y, Zhang Y, Zhang E, Zhang T. Age-Related Changes in Shear Wave Elastography Parameters of the Gastrocnemius Muscle in Association with Physical Performance in Healthy Adults. Gerontology 2021;67:306-13. [Crossref] [PubMed]
- Maslarska M, Weis C, Bode C, Hehrlein C. Shear Wave Elastography of Peripheral Muscle Weakness in Patients with Chronic Congestive Heart Failure. Ultrasound Med Biol 2018;44:2531-9. [Crossref] [PubMed]
- Bachasson D, Dubois GJR, Allenbach Y, Benveniste O, Hogrel JY. Muscle Shear Wave Elastography in Inclusion Body Myositis: Feasibility, Reliability and Relationships with Muscle Impairments. Ultrasound Med Biol 2018;44:1423-32. [Crossref] [PubMed]
- Chen ZT, Jin FS, Guo LH, Li XL, Wang Q, Zhao H, Sun LP, Xu HX. Value of conventional ultrasound and shear wave elastography in the assessment of muscle mass and function in elderly people with type 2 diabetes. Eur Radiol 2023;33:4007-15. [Crossref] [PubMed]
- Nishihara K, Kawai H, Kera T, Hirano H, Fujiwara Y, Ihara K, Kim H, Obuchi S. Comparisons of muscle thicknesses, echo intensities, and motor functions between community-dwelling older Japanese adults with and without diabetes. Arch Gerontol Geriatr 2021;97:104516. [Crossref] [PubMed]
- American Diabetes Association. 2. Classification and Diagnosis of Diabetes: Standards of Medical Care in Diabetes-2019. Diabetes Care 2019;42:S13-28. [Crossref] [PubMed]
- Pan Q, Li Q, Deng W, Zhao D, Qi L, Huang W, Ma L, Li H, Li Y, Lyu X, Wang A, Yao H, Xing X, Guo L. Prevalence of and Risk Factors for Peripheral Neuropathy in Chinese Patients With Diabetes: A Multicenter Cross-Sectional Study. Front Endocrinol (Lausanne) 2018;9:617. [Crossref] [PubMed]
- Flansbjer UB, Lexell J, Brogårdh C. Long-term benefits of progressive resistance training in chronic stroke: a 4-year follow-up. J Rehabil Med 2012;44:218-21. [Crossref] [PubMed]
- Parraca JA, Adsuar JC, Domínguez-Muñoz FJ, Barrios-Fernandez S, Tomas-Carus P. Test-Retest Reliability of Isokinetic Strength Measurements in Lower Limbs in Elderly. Biology (Basel) 2022;11:802. [Crossref] [PubMed]
- Roberts HC, Denison HJ, Martin HJ, Patel HP, Syddall H, Cooper C, Sayer AA. A review of the measurement of grip strength in clinical and epidemiological studies: towards a standardised approach. Age Ageing 2011;40:423-9. [Crossref] [PubMed]
- Bavu E, Gennisson JL, Couade M, Bercoff J, Mallet V, Fink M, Badel A, Vallet-Pichard A, Nalpas B, Tanter M, Pol S. Noninvasive in vivo liver fibrosis evaluation using supersonic shear imaging: a clinical study on 113 hepatitis C virus patients. Ultrasound Med Biol 2011;37:1361-73. [Crossref] [PubMed]
- Du LJ, He W, Cheng LG, Li S, Pan YS, Gao J. Ultrasound shear wave elastography in assessment of muscle stiffness in patients with Parkinson's disease: a primary observation. Clin Imaging 2016;40:1075-80. [Crossref] [PubMed]
- Alfuraih AM, O'Connor P, Hensor E, Tan AL, Emery P, Wakefield RJ. The effect of unit, depth, and probe load on the reliability of muscle shear wave elastography: Variables affecting reliability of SWE. J Clin Ultrasound 2018;46:108-15. [Crossref] [PubMed]
- Shrout PE, Fleiss JL. Intraclass correlations: uses in assessing rater reliability. Psychol Bull 1979;86:420-8. [Crossref] [PubMed]
- Kalyani RR, Metter EJ, Egan J, Golden SH, Ferrucci L. Hyperglycemia predicts persistently lower muscle strength with aging. Diabetes Care 2015;38:82-90. [Crossref] [PubMed]
- Andersen H, Poulsen PL, Mogensen CE, Jakobsen J. Isokinetic muscle strength in long-term IDDM patients in relation to diabetic complications. Diabetes 1996;45:440-5. [Crossref] [PubMed]
- Leenders M, Verdijk LB, van der Hoeven L, Adam JJ, van Kranenburg J, Nilwik R, van Loon LJ. Patients with type 2 diabetes show a greater decline in muscle mass, muscle strength, and functional capacity with aging. J Am Med Dir Assoc 2013;14:585-92. [Crossref] [PubMed]
- Volpato S, Bianchi L, Lauretani F, Lauretani F, Bandinelli S, Guralnik JM, Zuliani G, Ferrucci L. Role of muscle mass and muscle quality in the association between diabetes and gait speed. Diabetes Care 2012;35:1672-9. [Crossref] [PubMed]
- Zhu S, Lin W, Chen S, Qi H, Wang S, Zhang A, Cai J, Lai B, Sheng Y, Ding G. The correlation of muscle thickness and pennation angle assessed by ultrasound with sarcopenia in elderly Chinese community dwellers. Clin Interv Aging 2019;14:987-96. [Crossref] [PubMed]
- Hulett NA, Scalzo RL, Reusch JEB. Glucose Uptake by Skeletal Muscle within the Contexts of Type 2 Diabetes and Exercise: An Integrated Approach. Nutrients 2022;14:647. [Crossref] [PubMed]
- Nijholt W, Scafoglieri A, Jager-Wittenaar H, Hobbelen JSM, van der Schans CP. The reliability and validity of ultrasound to quantify muscles in older adults: a systematic review. J Cachexia Sarcopenia Muscle 2017;8:702-12. [Crossref] [PubMed]
- Abe T, Kondo M, Kawakami Y, Fukunaga T. Prediction equations for body composition of Japanese adults by B-mode ultrasound. Am J Hum Biol 1994;6:161-70. [Crossref] [PubMed]
- Wang J, Hu Y, Tian G. Ultrasound measurements of gastrocnemius muscle thickness in older people with sarcopenia. Clin Interv Aging 2018;13:2193-9. [Crossref] [PubMed]
- Ata AM, Kara M, Kaymak B, Gürçay E, Çakır B, Ünlü H, Akıncı A, Özçakar L. Regional and total muscle mass, muscle strength and physical performance: The potential use of ultrasound imaging for sarcopenia. Arch Gerontol Geriatr 2019;83:55-60. [Crossref] [PubMed]
- Ikezoe T, Nakamura M, Shima H, Asakawa Y, Ichihashi N. Association between walking ability and trunk and lower-limb muscle atrophy in institutionalized elderly women: a longitudinal pilot study. J Physiol Anthropol 2015;34:31. [Crossref] [PubMed]
- Ando R, Saito A, Umemura Y, Akima H. Local architecture of the vastus intermedius is a better predictor of knee extension force than that of the other quadriceps femoris muscle heads. Clin Physiol Funct Imaging 2015;35:376-82. [Crossref] [PubMed]
- Taş S, Onur MR, Yılmaz S, Soylu AR, Korkusuz F. Shear Wave Elastography Is a Reliable and Repeatable Method for Measuring the Elastic Modulus of the Rectus Femoris Muscle and Patellar Tendon. J Ultrasound Med 2017;36:565-70. [Crossref] [PubMed]
- Yu F, Fan Y, Sun H, Li T, Dong Y, Pan S. Intermuscular adipose tissue in Type 2 diabetes mellitus: Non-invasive quantitative imaging and clinical implications. Diabetes Res Clin Pract 2022;187:109881. [Crossref] [PubMed]
- Hairi NN, Cumming RG, Naganathan V, Handelsman DJ, Le Couteur DG, Creasey H, Waite LM, Seibel MJ, Sambrook PN. Loss of muscle strength, mass (sarcopenia), and quality (specific force) and its relationship with functional limitation and physical disability: the Concord Health and Ageing in Men Project. J Am Geriatr Soc 2010;58:2055-62. [Crossref] [PubMed]
- He X, Huang WY, Leong HT, Qiu JH, Ma CC, Fu SC, Ong MT, Yung PS. Decreased passive muscle stiffness of vastus medialis is associated with poorer quadriceps strength and knee function after anterior cruciate ligament reconstruction. Clin Biomech (Bristol, Avon) 2021;82:105289. [Crossref] [PubMed]
- Riemann BL, Lephart SM. The Sensorimotor System, Part II: The Role of Proprioception in Motor Control and Functional Joint Stability. J Athl Train 2002;37:80-4.
- Tuttle LJ, Sinacore DR, Cade WT, Mueller MJ. Lower physical activity is associated with higher intermuscular adipose tissue in people with type 2 diabetes and peripheral neuropathy. Phys Ther 2011;91:923-30. [Crossref] [PubMed]
- IJzerman TH, Schaper NC, Melai T, Meijer K, Willems PJ, Savelberg HH. Lower extremity muscle strength is reduced in people with type 2 diabetes, with and without polyneuropathy, and is associated with impaired mobility and reduced quality of life. Diabetes Res Clin Pract 2012;95:345-51. [Crossref] [PubMed]
- Almurdhi MM, Reeves ND, Bowling FL, Boulton AJ, Jeziorska M, Malik RA. Reduced Lower-Limb Muscle Strength and Volume in Patients With Type 2 Diabetes in Relation to Neuropathy, Intramuscular Fat, and Vitamin D Levels. Diabetes Care 2016;39:441-7. [Crossref] [PubMed]
- Romano A, Staber D, Grimm A, Kronlage C, Marquetand J. Limitations of Muscle Ultrasound Shear Wave Elastography for Clinical Routine-Positioning and Muscle Selection. Sensors (Basel) 2021;21:8490. [Crossref] [PubMed]
- Bortolotto C, Turpini E, Felisaz P, Fresilli D, Fiorina I, Raciti MV, Belloni E, Bottinelli O, Cantisani V, Calliada F. Median nerve evaluation by shear wave elastosonography: impact of "bone-proximity" hardening artifacts and inter-observer agreement. J Ultrasound 2017;20:293-9. [Crossref] [PubMed]