Optimizing image quality and minimizing radiation dose in pediatric abdominal multiphase contrast-enhanced computed tomography: a study on CARE kV and CARE Dose 4D
Introduction
Computed tomography (CT) has become a widely used clinical examination method because of its fast-scanning speed and wide scanning field (1-3). However, in pediatric clinics, children’s sensitivity to ionizing radiation is significantly higher than that of adults (4), making it important to follow the principle of as low as reasonably achievable (ALARA) when performing CT examinations (5). Recently, the use of CARE Dose 4D, iterative reconstruction, and various postprocessing technologies (6-9) has helped reduce the radiation dose during CT, particularly in children. For example, the use of 70-kV voltage has reduced the radiation dose by approximately 50% in CT angiography (CTA) examinations for children (10). The variable scanning parameters used in different phases of contrast-enhanced CT (CECT) in children are chosen based on the different requirements for image quality and image noise tolerance in each scan phase (11-13). CTA focuses on observing the vascular structure with high contrast, which can withstand more image noise and allow for low voltage and low radiation dose for scanning. Meanwhile, the parenchymal phase requires a higher radiation dose to ensure good image quality, which could distinguish structures. Using the same scanning parameters for each phase of multiphase CECT would inevitably increase the unnecessary radiation dose. Therefore, finding a way to quickly set the appropriate scanning parameters for different phases of pediatric abdominal CECT scans in order to reduce the radiation dose while maintaining image quality is an urgent problem to be solved in children’s clinics.
CARE kV can select the optimal pre-examination voltage for patients based on their individual size, density, and clinical needs. When combined with CARE Dose 4D, it can adjust the current to reasonable levels based on the scout view, ultimately minimizing radiation exposure and improving scan technique while preserving diagnostic accuracy. This is critical in safeguarding the health of pediatric patients (14-17). However, the application of CARE kV in pediatric care has not been extensively researched (10,18). Given that abdominal CECT is an important tool for assessing tumors and other diseases, particularly in children (19), and that it is performed frequently, optimizing the application of CARE kV can increase its clinical utilization in pediatric multiphase abdominal CECT.
CARE kV and CARE Dose 4D can be used separately to adjust scanning voltage (14-18) and reduce radiation dose (6-10), which has been confirmed in multiple previous studies. However, there is an expectation whether the combined implementation of CARE kV and CARE Dose 4D may yield improved outcomes in clinical practice. Therefore, this study aimed to investigate the impact of the conjoined application of CARE kV and CARE Dose 4D on voltage selection, image quality, and radiation dose in pediatric patients who are subjected to abdominal CECT, incorporating the three stages of unenhanced CT, CTA, and parenchymal CT with different dose saving optimization index (DI) value. Furthermore, the objective is to assess the practicability of utilizing CARE kV in pediatric patients undergoing abdominal CECT and to optimize the selection of scanning parameters for the purpose of achieving an optimal balance between radiation dose and image quality. We present this article in accordance with the STROBE reporting checklist (available at https://qims.amegroups.com/article/view/10.21037/qims-23-1181/rc).
Methods
General information
This retrospective cross-sectional study was conducted in accordance with the Declaration of Helsinki (as revised in 2013). The study was approved by the Ethics Committee of Beijing Children’s Hospital (No. 2022-E-164-Y). The study protocols were performed following the approved guidelines and regulations of our hospital and informed consent forms were signed by the parents of pediatric patients. Fifty-seven children who underwent multiphase abdominal CECT were retrospectively and continuously enrolled from June 1, 2022 to August 3, 2022. The inclusion criteria were as follows: (I) patients underwent multiphase abdominal CECT from June 1, 2022 to August 3, 2022; (II) patients were under 18 years of age. The exclusion criteria were as follows: clinical documents or imaging data were not completed.
CT imaging methodology
All data were acquired using a 64-row CT detector (SOMATOM Definition Edge VA48A, Siemens Healthcare, Forchheim, Germany). CARE kV was used to automatically adjust the scanning voltage, with a basic reference tube voltage of 120 kV and an adjustment range of 70–120 kV. CARE Dose 4D was used to automatically adjust the scanning current, with a basic reference tube current of 110 mAs and an adjustment range of 28–270 mAs. All examinations were performed in three scanning phases: unenhanced CT, CTA, and parenchymal. Each phase used a different DI for CARE kV to adjust the scanning voltage (13,16). Based on previous studies (13,16), the DI value was 3, 12, and 7 for the unenhanced scan phase, CTA phase, and parenchymal phase, respectively. Regarding the image reconstruction, a 3-mm-thick iterative reconstruction (sinogram affirmed iterative reconstruction, level 3) image was used. After acquiring the unenhanced CT images, an iodinated contrast medium (320 mgI/mL iodixanol) was administered using a high-pressure injector (Accutron CT-D, Medtron, Germany). The contrast medium dosage was calculated based on the body weight of each child (400–600 mgI/kg). The flow rate was adjusted based on a fixed injection time of 18 s, and the contrast-enhanced scan started 20 s after the administration of contrast injection for the CTA and 60 s after the administration of contrast injection for the parenchymal phase, during the CECT examination, no adverse events occurred due to the injection of contrast medium. During the scan, the children were positioned supine with their arms raised above their heads and allowed to breathe freely without holding their breath. If the children could not cooperate, oral sedatives were administered to keep them still during the scan.
Qualitative image quality evaluation
All images were transferred to the imaging workstation (Multi-Modality Workplace; Siemens Healthcare) and anonymized to conceal all patient and scanning data. One radiologist with 12 years of experience (9 years of adult radiology experience and 3 years of pediatric radiology experience) and another with 16 years of pediatric radiology work experience independently conducted subjective scoring. The default reconstruction window width of all images was set to 400, with a window level of 40. However, the window width and level were adjusted according to the radiologists’ requirements. Furthermore, the multiplanar reconstruction tool provided by the workstation was used to examine the images.
In terms of qualitative image quality, according to similar study (13), we used a 5-point Likert scale for two evaluations: (I) overall image quality and (II) subjective image noise. The evaluation criteria for the overall image quality were as follows: very good (5 points), good but with slightly unclear tissue edges (4 points), sufficient for diagnosis despite slightly fuzzy tissue edges (3 points), unable to diagnose because of indistinct tissue edges (2 points), and tissue edges completely indistinguishable (1 point). The criteria for the image noise were as follows: rare noise in the image (5 points), low amount of noise in the image (4 points), moderate noise that does not hinder diagnosis (3 points), excessive noise that impedes diagnosis (2 points), and severe noise that prevents structure identification (1 point).
Quantitative image quality measurement
Following the qualitative image evaluation, the two radiologists quantitatively evaluated the image and measured the image noise. The layer of the liver hilum was selected for the measurement image, and a circular region of interest (ROI) was selected, which comprised approximately 1/4 of the area of the descending aorta at the same level. The three ROIs were placed in the center of the descending aorta, the uniform part of the right lobe of the liver, and the uniform part of the right vertical spine muscle individually; thereafter, the CT value and standard deviation were measured. The standard deviation was used to represent the objective noise of the tissue. Furthermore, we measured three ROIs on the adjacent upper and lower slices simultaneously, and the average value of the three slices was recorded.
Radiation dose
The volume CT dose index (CTDIvol) and the dose length product (DLP) were recorded for the three scan phases. The effective dose (ED) was calculated using the following formula: ED = k × DLP, where k is the conversion factor. According to the ICRP103 recommendation (20), the k range for children depends on the scanning voltage and their age and falls between 0.0246 and 0.0935.
Statistical analysis
The subjective score and objective noise measurement values were expressed as mean ± standard deviation. For normally distributed data, analysis of variance was used for comparison between groups, followed by the Bonferroni correction test to determine differences. The data that did not follow a normal distribution were compared using the Friedman test. The kappa test was used to determine the consistency of the subjective scores of the two radiologists. All statistical analyses were performed using SPSS (version 17.0; IBM Corp., Armonk, NY, USA), and differences with P<0.05 (two-sided) were considered statistically significant.
Results
Patient characteristics
A total of 57 children (33 males and 24 females) with an average age of 6.52±4.30 years (age range, 0.1–15 years), and body mass index of 16.10±2.50 kg/m2 were selected retrospectively and continuously from June 1, 2022 to August 3, 2022. Among them, 17 cases were suspected of tumor lesions, 1 case of lymphangioma, 24 cases were suspected of urinary system abnormalities, and 15 cases of abdominal pain (Table 1).
Table 1
Characteristics | Values |
---|---|
Male:female, n | 33:24 |
Ages (years), mean ± SD (range) | 6.52±4.30 (0.1–15.0) |
Body weight (kg), mean ± SD (range) | 23.13±11.81 (4.0–59.0) |
Body height (cm), mean ± SD (range) | 115.6±30.04 (53.0–162.0) |
Body mass index (kg/m2), mean ± SD (range) | 16.10±2.50 (9.7–26.2) |
Neuroblastoma | 5 |
Wilm’s tumour | 4 |
Hepatoblastoma | 4 |
Lymphoma | 4 |
Angio lymphoma | 1 |
Malformation of urinary | 24 |
Abdominal pain | 15 |
SD, standard deviation.
Voltage distribution results and radiation dose
Voltage distribution results showed that the most selected voltage mode was 100, 70, and 80 kV in unenhanced CT, CTA, and the parenchymal phase, respectively (Table 2). There were significant statistical variances observed in the voltage selection across different phases (Friedman =85.31; P<0.001). Statistics showed that CTDIvol decreased by 49.37% and 20.30%; DLP decreased by 56.21% and 22.70%; and ED decreased by 65.80% and 37.66% when DI =12 and DI =7, respectively, compared with those when DI =3. The decrease in CTDIvol, DLP, and ED with the increase in the DI index was statistically significant (Table 3, Figure 1).
Table 2
Tube voltage (kV) | Unenhanced CT (DI =3) | CTA (DI =12) | Parenchymal phase (DI =7) | F value | P value |
---|---|---|---|---|---|
70 | 0 (0.00) | 36 (63.16) | 2 (3.51) | 85.31 | <0.001 |
80 | 4 (7.02) | 14 (24.56) | 32 (56.14) | ||
100 | 49 (85.96) | 5 (8.77) | 19 (33.33) | ||
120 | 4 (7.02) | 2 (3.51) | 4 (7.02) |
Data are presented as n (%). CT, computer tomography; DI, dose saving optimization index; CTA, CT angiography.
Table 3
Radiation dose | Unenhanced CT (DI =3) | CTA (DI =12) | Parenchymal phase (DI =7) | F value | P value |
---|---|---|---|---|---|
CTDIvol (mGy) | 3.99±1.99† | 2.02±1.71†§ | 3.18±2.10§ | 14.93 | <0.001 |
DLP (mGy∙cm) | 164.14±90.05†‡ | 65.31±56.87†§ | 115.29±92.25‡§ | 19.34 | <0.001 |
ED (mSv) | 4.62±3.31 | 1.58±1.08 | 2.88±1.56 | 12.35 | <0.001 |
Data are presented as mean ± SD. †, there was a statistical difference between unenhanced CT and CTA; ‡, there was a statistical difference between unenhanced CT and parenchymal phase; §, there was a statistical difference between CTA and parenchymal phase. CT, computer tomography; DI, dose saving optimization index; CTA, CT angiography; CTDIvol, volume CT dose index; DLP, the dose length product; ED, effective dose; SD, standard deviation.
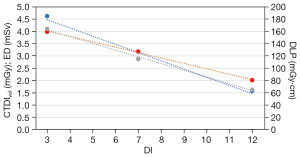
Image quality evaluation
Table 4 shows the qualitative and quantitative results of image quality. The results indicated that all images met the diagnostic requirements through qualitative evaluation. The overall quality score for CTA (DI =12) was the highest and that for unenhanced CT (DI =3) was the lowest. No significant correlation was observed between the quality score and DI value (Figure 2). The image noise score for CTA was the lowest (3.35±0.49) and that for unenhanced CT was the highest (3.93±0.39). The image noise score decreased with an increase in the DI value; the kappa value of the two observers was 0.83 (P<0.001). The overall qualitative image score in CTA was the highest at 4.50±0.45, indicating that arteries, especially small arterial blood vessels, can be displayed better, a good image quality was guaranteed while reducing the radiation dose (Figure 3).
Table 4
Image quality | Unenhanced CT (DI =3) | CTA (DI =12) | Parenchymal phase (DI =7) | F value | P value |
---|---|---|---|---|---|
Overall image quality | 4.24±0.42† | 4.50±0.45† | 4.41±0.49 | 27.78 | 0.010 |
Subjective image noise | 3.93±0.39† | 3.35±0.49†§ | 3.81±0.42§ | 5.09 | <0.001 |
SD of aorta | 5.75±1.53†‡ | 9.99±7.69†§ | 6.98±1.99‡§ | 27.98 | <0.001 |
SD of liver | 5.18±1.38†‡ | 8.17±2.21†§ | 6.13±1.68‡§ | 41.65 | <0.001 |
SD of muscle | 4.94±1.07†‡ | 7.93±1.72†§ | 5.66±1.71‡§ | 59.09 | <0.001 |
Data are presented as mean ± SD. †, there was a statistical difference between unenhanced CT and CTA; ‡, there was a statistical difference between unenhanced CT and parenchymal phase; §, there was a statistical difference between CTA and parenchymal phase. CT, computer tomography; DI, dose saving optimization index; CTA, CT angiography; SD, standard deviation.
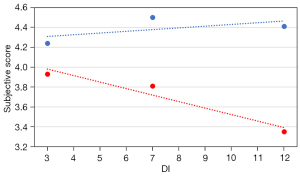
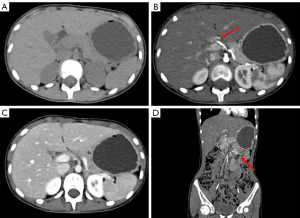
The objective noise for unenhanced CT (DI =3) was the lowest and that for CTA (DI =12) was the highest, with a statistical difference (P=0.010). The image noise for CTA (DI =12) was 57.72–73.73% higher than that for unenhanced CT (DI =3) and that for the parenchymal phase (DI =7) was 14.57–21.39% higher than that for unenhanced CT. The noise value increased with an increase in the DI value of all tissues (Figure 4).
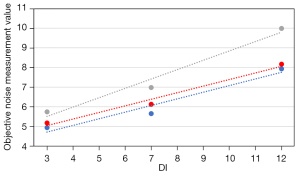
Discussion
Current research has emphasized a critical problem: how to reduce the radiation dose in children during CT. Reducing unnecessary scans or scanning procedures is the most effective measure (14). During the necessary scan procedure, the radiation dose could be reduced through automatic tube current modulation and iterative reconstruction technologies (21). Automatic tube current modulation technologies, such as CARE Dose 4D, can dynamically adjust the current intensity on the z-axis according to the size of the child to reduce the radiation dose compared with that during fixed-current CT. Combined with the iterative reconstruction algorithm, it can reduce the radiation dose, ensure or improve the image quality, and meet the diagnostic requirements (14,21,22).
Children’s bodies are small and suitable for low-voltage scanning. A previous phantom experiment (22) highlighted that a small body size can avoid the hardening artifacts produced by low-voltage scanning and can benefit from the effects of low voltage to enhance the CT value of the contrast medium in order to increase the contrast-noise ratio of enhanced CT images and improve image quality (23,24). To observe the density and enhancement degree of different tissues, CECT examination generally requires the unenhanced CT, CTA, and parenchymal phases. Unenhanced CT is used to observe high-density areas, such as those with bleeding, calcification or urinary stone (25), and to determine the scanning range for the subsequent phases. However, the density difference of each tissue in unenhanced CT is small, requiring a higher radiation dose to reduce image noise and maintain the contrast-noise ratio that meets the diagnostic requirements. CTA is mainly used to observe arteries and is suitable for using low-voltage scanning protocols to improve the CT value of the iodine contrast medium and increase the image contrast. In cases of high contrast, it can tolerate higher image noise to ensure the contrast-noise ratio of arteries. In the parenchymal phase, the contrast medium can also be used to increase the tissue contrast to determine the region of lesions. Because the contrast degree is lower than that in CTA, the radiation dose should be higher than that in CTA; however, the required radiation dose is lower than that in unenhanced CT. Therefore, the voltage and radiation dose during contrast-enhanced scanning in children are different. To reduce the radiation dose more accurately, the scanning parameters should be set for each scanning phase.
In this study, each child was scanned in three phases and different DI values were used. Because the same scout view was used in all three phases, the changes in the voltage and radiation dose in the three phases were mainly affected by DI. The results showed that the DI value of unenhanced CT was 3, which was the lowest value in this study, furthermore, 100 kV was selected as the tube voltage in 85.96% of the cases (49/57), and the radiation dose was the highest in this phase. The CTDIvol reached 3.99±1.99 mGy, and the obtained image had the lowest noise (5.75±1.53). The DI value of CTA was 12, which was the highest value among the three phases. Moreover, 70 kV was selected as the tube voltage in 63.16% of the cases (36/57), whereas 70 or 80 kV was selected as the tube voltage in 87.72% of the cases (50/57). The lower voltage ensures the contrast degree of the arteries. Although the radiation dose was significantly reduced (to only 50.63% of unenhanced CT) and the image noise increased to the highest value (9.99±7.69), the lower scan voltage improves the contrast of the arteries and ensures good image quality. However, if the DI value is set to 12 in other scanning phases, it will inevitably superimpose a high level of image noise on the image, which reduces the contrast, and the subjective score may be less than 3 points, making the image difficult to diagnose. Therefore, in the phase beyond CTA, excessive DI values should be employed with caution. In the parenchymal phase, DI was 7, and the radiation dose was 20.30% lower than that in unenhanced CT. Although the subjective and objective noise values were slightly higher than those in the unenhanced CT phase, in 89.47% of the cases (51/57), 80 or 100 kV was chosen, which was lower than that in the unenhanced CT phase. Thus, the contrast of the enhanced image was also improved, making the boundaries of the parenchymal organs clearer, and the subjective score remained higher than that in the unenhanced CT phase.
This study has some limitations. First, the sample size was small, the effect of diseases on the image quality was not considered in this experiment, such as sever artery stenosis, which may cause difficulties in diagnosis under low dose scan, on the other hand, the patients’ age was not covering the newborn and not stratified, which will be included in a later experimental design. Second, using different scan tube voltage values will change the CT value of tissues. Because of the variation in scanning voltage between CECT and unenhanced CT, the enhancement degree will be determined differently. However, given that the difference is mainly caused by the iodine ions in CECT, the effect is minor. Third, because the number of studies on children’s CARE kV that can be used as references is limited (14,26), the DI setting, reference tube voltage, and reference tube current in this study may be unsuitable. In future research, a more detailed DI research protocol will be set up to determine the DI values, reference tube voltage, and reference tube current suitable for different scanning phases. Finally, we did not evaluate the potential impact of iterative reconstruction on image quality. In subsequent studies, we should select sinogram affirmed iterative reconstruction with different intensities (grade 1–5) and observe the effect difference.
Conclusions
The combination of CARE kV and CARE Dose 4D can reduce the radiation dose and scan voltage by properly adjusting the DI values of multiphase CECT while meeting the diagnostic requirements.
Acknowledgments
The authors would like to thank Dr. Xiaocun Li and Dr. Jiaxing Wu for their help in understanding the CARE kV and CARE Dose 4D techniques.
Funding: This study was sponsored by
Footnote
Reporting Checklist: The authors have completed the STROBE reporting checklist. Available at https://qims.amegroups.com/article/view/10.21037/qims-23-1181/rc
Conflicts of Interest: All authors have completed the ICMJE uniform disclosure form (available at https://qims.amegroups.com/article/view/10.21037/qims-23-1181/coif). The authors have no conflicts of interest to declare.
Ethical Statement: The authors are accountable for all aspects of the work in ensuring that questions related to the accuracy or integrity of any part of the work are appropriately investigated and resolved. The study was conducted in accordance with the Declaration of Helsinki (as revised in 2013). The study was approved by the Ethics Committee of Beijing Children’s Hospital (No. 2022-E-164-Y). Informed consent forms were signed by the parents of pediatric patients.
Open Access Statement: This is an Open Access article distributed in accordance with the Creative Commons Attribution-NonCommercial-NoDerivs 4.0 International License (CC BY-NC-ND 4.0), which permits the non-commercial replication and distribution of the article with the strict proviso that no changes or edits are made and the original work is properly cited (including links to both the formal publication through the relevant DOI and the license). See: https://creativecommons.org/licenses/by-nc-nd/4.0/.
References
- Hoffman RM, Atallah RP, Struble RD, Badgett RG. Lung Cancer Screening with Low-Dose CT: a Meta-Analysis. J Gen Intern Med 2020;35:3015-25. [Crossref] [PubMed]
- Sun J, Chen C, Peng Y, Zhang Y, Tian H, Yu J, Cao J, Zeng Q. Comparison of magnetic resonance imaging and computed tomography to measure preoperative parameters of children with pectus excavatum. Pediatr Investig 2019;3:102-9. [Crossref] [PubMed]
- He M, Wang C, Xu L, Zhang H, Liu Y, Zhao Y, He S, Zhang Y, Yang H, Liu Y, Miao M, Chen Z, Pang L. Epidemiological and clinical characteristics of 35 children with COVID-19 in Beijing, China. Pediatr Investig 2020;4:230-5. [Crossref] [PubMed]
- Martin CJ. Effective dose: practice, purpose and pitfalls for nuclear medicine. J Radiol Prot 2011;31:205-19. [Crossref] [PubMed]
- Sun J, Li H, Li J, Yu T, Li M, Zhou Z, Peng Y. Improving the image quality of pediatric chest CT angiography with low radiation dose and contrast volume using deep learning image reconstruction. Quant Imaging Med Surg 2021;11:3051-8. [Crossref] [PubMed]
- Markart S, Fischer TS, Wildermuth S, Dietrich TJ, Alkadhi H, Leschka S, Guesewell S, Ditchfield MR, Oezden I, Lutters G, Waelti SL. Organ-based tube current modulation and bismuth eye shielding in pediatric head computed tomography. Pediatr Radiol 2022;52:2584-94. [Crossref] [PubMed]
- Greffier J, Dabli D, Hamard A, Belaouni A, Akessoul P, Frandon J, Beregi JP. Effect of a new deep learning image reconstruction algorithm for abdominal computed tomography imaging on image quality and dose reduction compared with two iterative reconstruction algorithms: a phantom study. Quant Imaging Med Surg 2022;12:229-43. [Crossref] [PubMed]
- Sun J, Li H, Li H, Li M, Gao Y, Zhou Z, Peng Y. Application of deep learning image reconstruction algorithm to improve image quality in CT angiography of children with Takayasu arteritis. J Xray Sci Technol 2022;30:177-84. [Crossref] [PubMed]
- McCollough CH, Guimarães L, Fletcher JG. In defense of body CT. AJR Am J Roentgenol 2009;193:28-39. [Crossref] [PubMed]
- Marukawa Y, Sato S, Tanaka T, Tada A, Kanie Y, Kanazawa S. Evaluating Low-kV Dual-source CT Angiography by High-pitch Spiral Acquisition and Iterative Reconstruction in Pediatric Congenital Heart Disease Patients. Acta Med Okayama 2017;71:407-12. [Crossref] [PubMed]
- Sun J, Yang L, Zhou Z, Zhang D, Han W, Zhang Q, Peng Y. Performance evaluation of two iterative reconstruction algorithms, MBIR and ASIR, in low radiation dose and low contrast dose abdominal CT in children. Radiol Med 2020;125:918-25. [Crossref] [PubMed]
- Khong PL, Ringertz H, Donoghue V, Frush D, Rehani M, Appelgate K, Sanchez R. ICRP publication 121: radiological protection in paediatric diagnostic and interventional radiology. Ann ICRP 2013;42:1-63. [Crossref] [PubMed]
- Sun J, Zhang Q, Zhou Z, Jia C, Yang W, Li H, Peng Y. Optimal tube voltage for abdominal enhanced CT in children: a self-controlled study. Acta Radiol 2020;61:101-9. [Crossref] [PubMed]
- Shin HJ, Chung YE, Lee YH, Choi JY, Park MS, Kim MJ, Kim KW. Radiation dose reduction via sinogram affirmed iterative reconstruction and automatic tube voltage modulation (CARE kV) in abdominal CT. Korean J Radiol 2013;14:886-93. [Crossref] [PubMed]
- Yang B, Li ZL, Gao Y, Yang YY, Zhao W. Image quality evaluation for CARE kV technique combined with iterative reconstruction for chest computed tomography scanning. Medicine (Baltimore) 2017;96:e6175. [Crossref] [PubMed]
- Vingiani V, Abadia AF, Schoepf UJ, Fischer AM, Varga-Szemes A, Sahbaee P, Allmendinger T, Giovagnoli DA, Hudson HT, Marano R, Tinnefeld FC, Martin SS. Individualized coronary calcium scoring at any tube voltage using a kV-independent reconstruction algorithm. Eur Radiol 2020;30:5834-40. [Crossref] [PubMed]
- MacDougall RD, Kleinman PL, Callahan MJ. Size-based protocol optimization using automatic tube current modulation and automatic kV selection in computed tomography. J Appl Clin Med Phys 2016;17:328-41. [Crossref] [PubMed]
- Hojreh A, Homolka P, Gamper J, Unterhumer S, Kienzl-Palma D, Balassy C, Wrba T, Prosch H. Automated tube voltage selection in pediatric non-contrast chest CT. PLoS One 2018;13:e0204794. [Crossref] [PubMed]
- Brisse HJ, McCarville MB, Granata C, Krug KB, Wootton-Gorges SL, Kanegawa K, Giammarile F, Schmidt M, Shulkin BL, Matthay KK, Lewington VJ, Sarnacki S, Hero B, Kaneko M, London WB, Pearson AD, Cohn SL, Monclair T. Guidelines for imaging and staging of neuroblastic tumors: consensus report from the International Neuroblastoma Risk Group Project. Radiology 2011;261:243-57. [Crossref] [PubMed]
- Deak PD, Smal Y, Kalender WA. Multisection CT protocols: sex- and age-specific conversion factors used to determine effective dose from dose-length product. Radiology 2010;257:158-66. [Crossref] [PubMed]
- Wang L, Gong S, Yang J, Zhou J, Xiao J, Gu JH, Yang H, Zhu J, He B. CARE Dose 4D combined with sinogram-affirmed iterative reconstruction improved the image quality and reduced the radiation dose in low dose CT of the small intestine. J Appl Clin Med Phys 2019;20:293-307. [Crossref] [PubMed]
- Tang YC, Liu YC, Hsu MY, Tsai HY, Chen CM. Adaptive Iterative Dose Reduction 3D Integrated with Automatic Tube Current Modulation for CT Coronary Artery Calcium Quantification: Comparison to Traditional Filtered Back Projection in an Anthropomorphic Phantom and Patients. Acad Radiol 2018;25:1010-7. [Crossref] [PubMed]
- Zhang J, Kang S, Han D, Xie X, Deng Y. Application of intelligent optimal kV scanning technology (CARE kV) in dual-source computed tomography (DSCT) coronary angiography. Int J Clin Exp Med 2015;8:17644-53.
- Gottumukkala RV, Kalra MK, Tabari A, Otrakji A, Gee MS, Advanced CT. Techniques for Decreasing Radiation Dose, Reducing Sedation Requirements, and Optimizing Image Quality in Children. Radiographics 2019;39:709-26. [Crossref] [PubMed]
- Xiao JM, Hippe DS, Zecevic M, Zamora DA, Cai LM, Toia GV, Chandler AG, Dighe MK, O'Malley RB, Shuman WP, Wang CL, Mileto A. Virtual Unenhanced Dual-Energy CT Images Obtained with a Multimaterial Decomposition Algorithm: Diagnostic Value for Renal Mass and Urinary Stone Evaluation. Radiology 2021;298:611-9. [Crossref] [PubMed]
- Yu L, Li H, Fletcher JG, McCollough CH. Automatic selection of tube potential for radiation dose reduction in CT: a general strategy. Med Phys 2010;37:234-43. [Crossref] [PubMed]