Revolutionizing vascular imaging: trends and future directions of 4D flow MRI based on a 20-year bibliometric analysis
Introduction
Quantitative flow imaging is clinically crucial in certain pathological conditions such as aneurysms, valvular heart disease, and portal hypertension. It provides visualization of vascular anatomy and valuable hemodynamic information (1-5). More recently, 4-dimensional (4D) flow magnetic resonance imaging (MRI), alternatively known as 4D phase contrast (PC) or 3-dimensional (3D) time-resolved PC with 3-directional velocity encoding (VENC), has been increasingly performed in both cardiac and multiple vascular regions of the body (6-8).
PC MRI was first described in the 1980s and measures blood flow velocity using bipolar magnetic field gradients along a single direction (9-11); on this basis, 4D flow MRI acquires 3D data by phase-encoding selected blood flow velocities in any 3 dimensions in a single scan and combining this with the temporal resolution to provide a comprehensive hemodynamic assessment, with full volumetric coverage of the cardiovascular territory throughout the cardiac cycle (6,12,13). Studies have shown that 4D flow MRI offers a reliable in vivo hemodynamic assessment for measuring 3D velocity in various vascular regions, with excellent correlation to the standard technique (2D PC) (14-16). Moreover, the derived fluid mechanics parameters, such as wall shear forces, pulse wave velocity (PWV), pressure gradients, and other metrics, have been suggested to predict the development of various cardiovascular pathologies (17-20).
Currently, the applications of 4D flow MRI have been extended to detect hemodynamic changes in large cerebral arteries and veins, carotid arteries, aorta, abdominal (hepatic and renal arteries), and peripheral vessels (21-24). Many articles have focused on improving the technique of 4D flow MRI and its clinical application, but few studies have statistically analyzed these publications. Bibliometrics, a widely used approach to analyze large scientific data, uses qualitative and quantitative analysis to assess research hotspots and trends (25-27). The CiteSpace software developed by Chen et al. is extensively used in the medical field and provides a robust bibliometric and visual analysis to examine the dynamics of disciplinary development over time (28,29).
In this study, we used CiteSpace (version 6.1. R6; https://citespace.podia.com/) to visualize and analyze the relevant literature on 4D flow MRI published in the Web of Science Core Collection (WoSCC) database between 2003 and 2022. We aimed to build a knowledge structure of 4D flow MRI and explore research hotspots, frontier areas, and growing trends in the field over time.
Methods
Data sources
The WoSCC database was used for a literature search on 4D flow MRI. The search terms included the following: Topic = (“4D flow MRI” OR “four-dimensional flow MRI” OR “four-dimensional flow” OR “4D flow” OR “4D PC MRI” OR “four-dimensional phase contrast MRI” OR “four-dimensional phase contrast” OR “four-dimensional PC” OR “4D PC” OR “3D time-resolved PC with three-directional velocity encoding”) AND Language = English; literature type = article or review. All article searches and data extractions were performed on 20 January 2023 to avoid changes due to daily database updates.
Raw data were then acquired from WoSCC in plain-text format, including full records with references. All downloaded data were independently screened by reviewers (PS Ma and ZW Zhang) to exclude articles unrelated to 4D flow MRI. In case of disagreement between the 2 reviewers, a third independent reviewer (LS Zhu) was involved to assist in the decision-making process. Figure 1 shows a flowchart of this study. As all data were obtained directly from the database, no ethical declaration is provided in this article.
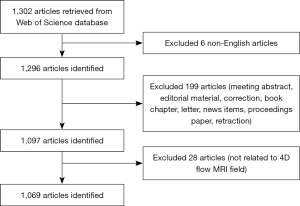
Statistical analysis
Microsoft Excel 2016 (https://www.microsoft.com/en-au/microsoft-365/excel) and CiteSpace (version 6.1.R6) were utilized to analyze the collected data. Excel 2016 showed the annual publication trend in terms of the number of articles, whereas CiteSpace performed co-occurrence keyword analysis to explore research hotspots and emerging trends, keyword burst analysis to identify frontier areas, and the timeline view and cluster analysis of co-cited references to reveal the current hotspots and underlying knowledge structure of research on 4D flow MRI. The time parameters of the software were set to cover the period from 2003 to 2022, with a time interval of 1 year. The appropriate thresholds were determined based on distinct nodes, and the map clipping approaches were configured to “Pathfinder”, “Pruning sliced networks”, and “Pruning the merged network”; the visualization options were “Cluster View Static” and “Show Merged Network”.
Results
Annual publications
A total of 1,069 papers met the search criteria and were selected for further analysis. Figure 2 shows the annual distribution of 4D flow MRI publications. The first period was between 2003 and 2009, when only a few 4D flow MRI studies were published each year. Since then, the number of publications has increased steadily and slowly from 2010 to 2014, remaining at 9–35 publications per year. Notably, there has been an increase in the number of published papers since 2015 (from 65 in 2015 to 180 in 2022), indicating that this field has consistently received growing attention from academics worldwide.
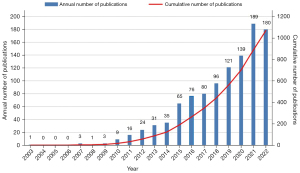
Analysis of journals
Articles on 4D flow MRI were published in 206 scholarly journals. The number of publications in the Journal of Magnetic Resonance Imaging was the highest [impact factor (IF) 2022 =4.4; 135 publications], followed by Magnetic Resonance in Medicine (IF2022 =3.3; 80 publications), Journal of Cardiovascular Magnetic Resonance (IF2022 =6.4; 62 publications), and European Radiology (IF2022 =5.9; 32 publications). Table 1 lists the top 10 journals with the most published 4D flow MRI studies, which may be a useful resource and inspiration for new investigators searching for further research.
Table 1
Ranking | Journals | IF | No. of published papers |
---|---|---|---|
1 | Journal of Magnetic Resonance Imaging | 4.4 | 135 |
2 | Magnetic Resonance in Medicine | 3.3 | 80 |
3 | Journal of Cardiovascular Magnetic Resonance | 6.4 | 62 |
4 | European Radiology | 5.9 | 32 |
5 | Magnetic Resonance Imaging | 2.5 | 31 |
6 | International Journal of Cardiovascular Imaging | 2.1 | 29 |
7 | Frontiers in Cardiovascular Medicine | 3.6 | 24 |
8 | Magnetic Resonance in Medical Sciences | 3.0 | 23 |
9 | Scientific Reports | 4.6 | 23 |
10 | Annals of Biomedical Engineering | 3.8 | 21 |
IF, impact factor calculated in 2022.
Analysis of countries and institutes
A total of 1,069 research articles on 4D flow MRI were published in 48 countries/regions. Figure 3A shows the cooperative network involving over 10 countries or regions. The top 10 countries/regions are listed in Table 2. Betweenness centrality (BC) is a crucial metric that highlights the significance of a node within a network; nodes with higher BC play a more pivotal and influential role in the realm of 4D flow MRI. The United States of America (USA) produced the most articles [498], accounting for 46.6% of the total, followed by Germany (221 articles), the Netherlands (119 articles), the United Kingdom (UK) (108 articles), and Sweden (105 articles). Although the USA contributed the most articles, as can be seen in the diagram, its BC value was zero, suggesting that the USA is a world leader in this field but has limited international collaboration.
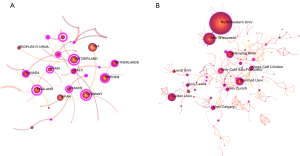
Table 2
Ranking | Country/region | Frequency | Centrality | Institution | Frequency | Centrality |
---|---|---|---|---|---|---|
1 | USA | 498 | 0 | Northwestern Univ | 171 | 0.17 |
2 | Germany | 221 | 0.9 | Univ Wisconsin | 92 | 0.17 |
3 | Netherlands | 119 | 0.31 | Stanford Univ | 64 | 0.38 |
4 | England | 108 | 1.01 | Leiden Univ | 60 | 0.11 |
5 | Sweden | 105 | 0.45 | Linkoping Univ | 53 | 0.34 |
6 | Japan | 89 | 0.08 | Univ Calif San Francisco | 40 | 0.44 |
7 | Switzerland | 80 | 0.94 | Kings Coll London | 36 | 0.11 |
8 | China | 60 | 0 | Univ Calgary | 32 | 0.15 |
9 | Canada | 52 | 0.24 | Lund Univ | 30 | 0.05 |
10 | France | 48 | 0.72 | Univ Zurich | 28 | 0.33 |
4D flow MRI, 4-dimensional flow magnetic resonance imaging.
Nearly 263 institutes participated in 4D flow MRI research (Figure 3B). Table 2 shows that Northwestern University published the highest number of papers [171], followed by Univ Wisconsin (92 articles), Stanford Univ (64 articles), Leiden Univ (60 articles), and Linkoping Univ (53 articles), each of which published 50 or more papers. In terms of BC, Univ Calif San Francisco (0.44), Stanford Univ (0.38), Linkoping Univ (0.34), Northwestern Univ (0.17), and Univ Wisconsin (0.17) collaborated closely with other institutions. In addition, 3 of the top 5 institutions with the most articles were from the USA, suggesting that Northwestern Univ, Univ Wisconsin, and Stanford Univ have conducted more in-depth research on 4D flow MRI.
Analysis of author co-authorship and co-citation
These 1,069 articles were written by 488 authors, with 255 co-authors. Table 3 lists the top 10 prolific and highly co-cited authors. Markl was the leading author in the 4D Flow MRI field with 152 published studies, followed by Barker (79 articles) and Wieben (59 articles), who all focused on the study of hemodynamic quantification and visualization of vascular structure using 4D flow MRI data. The co-authorship network is shown in Figure 4A, where Markl, Johnson, Carr, Wieben, and Westenberg played an important role in the collaboration of researchers because of their greater centrality. A map of the relationships between all cited authors is shown in Figure 4B. Markl (588 co-citations) ranked first among the top 10 co-cited authors, followed by Dyverfeldt (400 co-citations), Hope (218 co-citations), and Stalder (208 co-citations).
Table 3
Ranking | Author’s publication volume | Co-cited author | |||||
---|---|---|---|---|---|---|---|
Author | Frequency | Centrality | Author | Frequency | Centrality | ||
1 | Markl, Michael | 152 | 0.57 | Markl M | 588 | 0.01 | |
2 | Barker, Alex J | 79 | 0.08 | Dyverfeldt P | 400 | 0.03 | |
3 | Wieben, Oliver | 59 | 0.10 | Hope MD | 218 | 0.04 | |
4 | Ebbers, Tino | 47 | 0.05 | Stalder AF | 208 | 0.00 | |
5 | Westenberg, Jos J M | 41 | 0.10 | Frydrychowicz A | 203 | 0.00 | |
6 | Schnell, Susanne | 38 | 0.08 | Barker AJ | 202 | 0.16 | |
7 | Johnson, Kevin M | 31 | 0.40 | Van Ooij P | 199 | 0.05 | |
8 | Carr, James | 30 | 0.14 | Bock J | 169 | 0.05 | |
9 | Roldan-alzate, Alejandro | 27 | 0.02 | Stankovic Z | 161 | 0.00 | |
10 | Carlhall, Carl-Johan | 27 | 0.01 | Kilner PJ | 156 | 0.00 |
4D flow MRI, 4-dimensional flow magnetic resonance imaging.
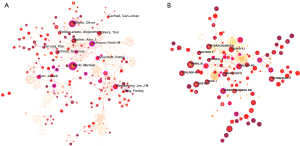
Analysis of reference co-citation
A total of 19,431 references were obtained, with the top 15 co-cited references with their co-citation counts listed in Table 4. The paper by Dyverfeldt et al. titled “4D flow cardiovascular magnetic resonance consensus statement”, published in the Journal of Cardiovascular Magnetic Resonance in 2015, is a key reference in the field of 4D flow MRI with a remarkable 187 co-citations. This paper is important because it provides a thorough analysis of 4D flow MRI in the context of cardiovascular applications, particularly in relation to the heart and large blood vessels such as the aorta and pulmonary arteries (30). The second most co-cited reference, “4D Flow MRI” by Markl et al. with 74 co-citations, plays a crucial role in summarizing acquisition strategies, including Cartesian and radial data acquisition, and analysis methods for 4D flow MRI, which has greatly shaped the research and clinical utilization of this imaging technique (13). Both papers serve as foundational resources that continue to guide research and innovation in 4D flow MRI, influencing its growth and adoption.
Table 4
References | Frequency | Centrality | Year |
---|---|---|---|
Dyverfeldt P, 2015 (30) | 187 | 0 | 2015 |
Markl M, 2012 (13) | 74 | 0 | 2012 |
Guzzardi DG, 2015 (31) | 66 | 0.07 | 2015 |
Markl M, 2011 (32) | 61 | 0 | 2011 |
Rodriguez-Palomares JF, 2018 (33) | 57 | 0.12 | 2018 |
Potters WV, 2015 (34) | 51 | 0.07 | 2015 |
Barker AJ, 2012 (35) | 49 | 0.04 | 2012 |
Garcia J, 2019 (36) | 45 | 0 | 2019 |
Mahadevia R, 2014 (37) | 43 | 0 | 2014 |
Schnell S, 2017 (38) | 42 | 0 | 2017 |
Bissell MM, 2013 (39) | 42 | 0.1 | 2013 |
Ma LE, 2019 (40) | 41 | 0 | 2019 |
Stankovic Z, 2014 (41) | 41 | 0.01 | 2014 |
van Ooij P, 2016 (42) | 37 | 0 | 2016 |
Hope MD, 2010 (21) | 36 | 0.02 | 2010 |
4D flow MRI, 4-dimensional flow magnetic resonance imaging.
The top 10 clusters, representing the main research hotspots, were generalized and ordered from the largest to smallest number of co-cited references (Figure 5). The modularity Q was 0.889 and the weighted average silhouette was 0.951, indicating a rational classification and uniformly distributed clusters internally. The first cluster was ‘‘#0 Fontan patient’’, followed by ‘‘#1 Valve-related hemodynamics’’, and “#2 Unruptured cerebral aneurysm”. This suggests that hemodynamic analysis is currently focused on the heart and intracranial arteries, with less attention paid to abdominal vessels such as the portal vein. In addition, themes such as “Fontan patient”, “aortic dilation”, and “neurovascular application” have become important research hotspots, according to the timeline view (Figure 6).
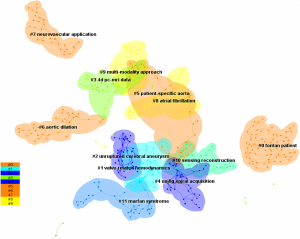
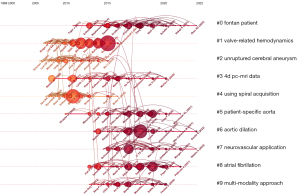
Analysis of keywords and burst keywords
The keyword co-occurrence map is shown in Figure 7A. The term “Blood flow” was used the most (n=373 times), followed by “4D flow MRI” (n=315), “Quantification” (n=277), “Wall shear stress” (n=258), and “Phase contrast MRI” (n=185; Table 5). Among the top 10 most-cited keywords, the nodes representing “Wall shear stress” and “Pattern” are marked with purple circles and have a good BC of 0.13, indicating that these nodes may reflect developing trends in the field of 4D flow MRI.
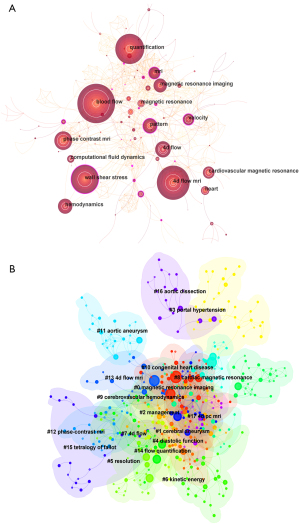
Table 5
Ranking | Count | Centrality | Keywords | Ranking | Count | Centrality | Keywords |
---|---|---|---|---|---|---|---|
1 | 373 | 0.04 | Blood flow | 11 | 126 | 0.09 | MRI |
2 | 315 | 0.01 | 4D flow MRI | 12 | 122 | 0.01 | Velocity |
3 | 277 | 0.01 | Quantification | 13 | 90 | 0.00 | Heart |
4 | 258 | 0.13 | Wall shear stress | 14 | 87 | 0.05 | Magnetic resonance |
5 | 185 | 0.06 | Phase contrast MRI | 15 | 84 | 0.06 | Computational fluid dynamics |
6 | 175 | 0.00 | 4D flow | 16 | 83 | 0.06 | Visualization |
7 | 161 | 0.01 | Hemodynamics | 17 | 75 | 0.02 | Bicuspid aortic value |
8 | 156 | 0.13 | Pattern | 18 | 65 | 0.11 | Disease |
9 | 137 | 0.02 | Magnetic resonance imaging | 19 | 60 | 0.00 | Valve |
10 | 133 | 0.05 | Cardiovascular magnetic resonance | 20 | 57 | 0.13 | Congenital heart disease |
4D flow MRI, 4-dimensional flow magnetic resonance imaging.
In the keyword clustering map (Figure 7B), 18 clusters were identified. The values of modularity Q and silhouette S were 0.7276 and 0.8742, respectively. The clusters were classified into 2 categories: (I) 4D flow MRI technique (“computational fluid dynamics”, “phase contrast”, “wall shear stress”, “resolution”, “geometry”, and “kinetic energy”); (II) Clinical applications related to 4D flow MRI, mainly involving quantification of hemodynamics in different parts of the body [“bicuspid aortic valve”, “cerebrovascular hemodynamics”, “human left ventricle”, “thoracic aorta”, “portal hypertension”, “heart failure” and “abdominal aortic aneurysm” (Table 6)].
Table 6
Cluster ID | Size | Silhouette value | Mean year | Cluster label | Label (LLR) |
---|---|---|---|---|---|
0 | 38 | 0.845 | 2012 | Magnetic resonance imaging | Magnetic resonance imaging; wall shear stress; phase contrast; bicuspid aortic valve; geometry |
1 | 38 | 0.901 | 2014 | Cerebral aneurysm | Cerebral aneurysm; computational fluid dynamics; intracranial aneurysm; intracranial aneurysms; relative residence time |
2 | 34 | 0.682 | 2014 | Management | Management; thoracic aorta; fetal; patient-specific heat map; automatic labeling |
3 | 31 | 0.858 | 2018 | Portal hypertension | Portal hypertension; cirrhosis; aortic disease; relative pressure; portal vein |
4 | 30 | 0.802 | 2017 | Diastolic function | Diastolic function; heart failure; flow components; 3d cine; human left ventricle |
5 | 29 | 0.926 | 2014 | Resolution | Resolution; aneurysm; abdominal aortic aneurysm; volunteer; reconstruction |
6 | 27 | 0.866 | 2017 | Kinetic energy | Kinetic energy; atrial fibrillation; cardiac MRI; stasis; left ventricle |
7 | 27 | 0.941 | 2014 | 4D flow | 4D flow; Alzheimer’s disease; middle cerebral artery; pulsatility index; 4D flow CMR |
8 | 26 | 0.913 | 2012 | Cardiac magnetic resonance | Cardiac magnetic resonance; cerebrospinal fluid; syringomyelia; Chiari malformation; Marfan syndrome |
9 | 22 | 0.873 | 2017 | Cerebrovascular hemodynamics | Cerebrovascular hemodynamics; aortic hemodynamics; patient-specific simulation; computational fluid dynamics; image-based modeling |
4D flow MRI, 4-dimensional flow magnetic resonance imaging; LLR, log-likelihood ratio; CMR, cardiovascular magnetic resonance.
The burst keyword mapping (Figure 8) shows the top 25 keywords with the highest burst strength. The first keyword burst, “visualization”, had the highest burst strength (14.79) and lasted from 2003 to 2016. The second keyword burst, “velocity mapping”, began in 2003 and lasted until 2013. The above data show that researchers have been focusing on visualizing vasculature and quantifying hemodynamics from 4D flow MRI for many years. The keywords “stiffness” and “tetralogy of Fallot” have attracted considerable attention in the past 4 years.
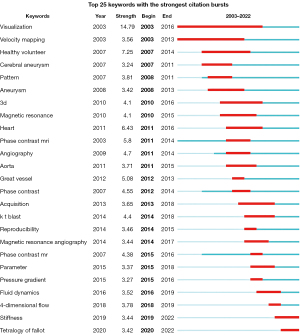
Discussion
To our knowledge, this is the first bibliometric analysis to be carried out for 4D flow MRI. Since its introduction, the technique has grown in popularity among researchers because it offers comprehensive volumetric assessment of blood flow and allows retrospective analysis at any angle (43,44), features that are not possible with conventional 2D PC-MRI. The number of articles published on 4D flow MRI has witnessed a remarkable surge over the last 2 decades, from one in 2003 to 180 in 2022.
General information
Among many countries, the USA has made the largest contribution. Three of the top 5 most productive institutions (Northwestern Univ, Univ Wisconsin, and Stanford Univ) and 7 of the top 10 prolific authors are from the USA. However, the low BC value suggests that increased collaboration and scholarly communication with other countries or institutions is needed to facilitate growth and advancement in this field. The remaining 4 of the top 5 active countries (Germany, Netherlands, England, and Sweden) are key collaborators with other countries in terms of their high BC values, despite publishing fewer articles than the USA.
The most influential author was found to be Markl from the USA, who ranked first in both cited papers [588] and published papers [152]. Most of Markl’s articles were published in top radiology or cardiovascular journals, such as the Journal of Cardiovascular Magnetic Resonance, Journal of Magnetic Resonance Imaging, Circulation: Cardiovascular Imaging and Radiology. Among Markl’s publications, the article “4D flow cardiovascular magnetic resonance consensus statement”, issued in the Journal of Cardiovascular Magnetic Resonance in 2015, was the most representative. The article is well known for its contribution to the development of consensus recommendations for the entire process of clinical implementation of 4D flow MRI, including patient preparation, data acquisition, handling, and analysis, as well as for providing an overview of potential clinical applications in various vascular regions, which is critical in guiding the direction of 4D flow MRI research. Dyverfeldt (30) from Sweden and Hope (45) from the USA have also made substantial contributions to the field, all focused on investigating the value of 4D flow MRI in visualizing and quantifying blood flow characteristics related to the cardiovascular system (33,35,46).
Research hotspots
Based on the above analysis, the hotspots and frontiers were separated into 2 major categories: technologies and clinical applications. The former contributes to the advancement of medical imaging and the quantification of complex hemodynamic parameters, whereas the latter is essential for diagnosis, ongoing monitoring, and treatment strategy optimization across a range of disease states.
4D flow technique
Early research on 4D flow MRI focused on advancing acquisition techniques and data analysis strategies, with keywords mainly including “wall shear stress”, “phase-contrast MRI”, “4D flow”, “hemodynamics”, “pattern”, “velocity mapping”, “visualization”, “resolution”, “reconstruction”, and “kinetic energy”. Non-Cartesian sampling, such as radial samplings [vastly undersampled isotropic projection reconstruction (PC-VIPR)] and spiral sampling, is a promising approach for accelerated PC-MRI acquisition compared to Cartesian sampling (47-49). In addition, approaches such as parallel imaging and compressed sensing are often used in tandem to further reduce acquisition time (50-52). On account of the complexity of 4D flow MRI data (3 spatial dimensions, 3 velocity directions, and time within cardiac cycles), researchers are actively seeking ways to optimize sequences to achieve higher image quality while scanning in a reasonable amount of time. Currently, the main tools used to process 4D flow MRI data include the commercial software cvi42 (https://www.circlecvi.com/), known for its user-friendly interface and automated quantification of blood flow parameters, and the in-house tool (e.g., Python, MATLAB), which allows customization through scripting. Notably, there is still significant variability in indicators obtained from 4D flow MRI across different post-processing software packages (53). Future work should focus on developing more intelligent automated algorithms, improving the quality of open-source tools, and further evaluating the consistency of various software to accelerate the use of post-processing tools in clinical settings.
VENC, crucial for estimating blood flow, emerged as an early research hotspot that persisted for almost a decade, as shown by keyword burst analysis. When it comes to 4D flow MRI, VENC is an essential component that makes it possible to precisely quantify blood flow. Researchers have investigated the appropriate range of VENC in detail in latest years, namely, 30–60 cm/s for the normal portal system and 80–120 cm/s for cerebrovascular applications (6,8). However, due to the disease’s complexity and diversity, there is no uniform standard for VENC, and it should be determined based on the clinical setting.
Furthermore, the emergence of advanced hemodynamic indices such as kinetic energy (KE) and wall shear stress (WSS) has intrigued the public interest (46,54). The KE of intracardiac blood flow reflects the myocardial work expended in accelerating the blood, and its value lies in its ability to provide detailed insights into the dynamic motion and energetic properties of fluids, particularly within the cardiovascular system studied by 4D flow MRI (55,56). WSS is a critical parameter in the study of fluid dynamics within blood vessels, representing the frictional force exerted tangentially by blood flow on the arterial endothelial layer. It directly influences the onset and progression of atherosclerosis, regulates endothelial function, and provides crucial information for risk assessment and treatment strategies (37,39,57,58). However, accurate determination of WSS faces numerous challenges, such as complex data processing, resolution and image quality concerns, motion artefacts, signal-to-noise ratio (SNR) issues, limitations in hemodynamics modelling, and translation of findings into clinical practice (59-61). Further advances are needed in data processing techniques, motion correction algorithms, mathematical modelling, improvement of SNR, and interdisciplinary collaboration between researchers and clinicians.
Surprisingly, artificial intelligence (AI) has not yet been identified in our hotspot analysis, despite reports of its use in the reconstruction of 4D flow MRI data (62,63) and the vessel segmentation of morphological images (64). This may be due to the fact that AI research in this area is still in its infancy, with few papers published. It is reasonable to expect that the progression of AI will play a pivotal role in expediting the clinical adoption and advancement of 4D flow MRI.
Clinical application
Clinical research on 4D flow MRI is mainly focused on the quantitative analysis of blood flow in the neurovascular, portal, and cardiovascular systems. 4D flow MRI research hotspots in the brain are “cerebral aneurysm”, “intracranial aneurysm”, and “middle cerebral artery”, categorized as Cluster 1 and 9. When used in the intracranial setting, 4D flow MRI is a useful tool for evaluating aneurysm wall instability and describing blood flow patterns in patients with intracranial aneurysms and determining how they correlate with aneurysm growth and risk of rupture (65-67). Zhang et al. found that WSS and low mean vorticity were significantly associated with the aneurysmal wall enhancement (AWE) pattern (an indicator of the inflammatory process) in a study of 48 patients with 49 unruptured intracranial aneurysms, highlighting the potential of flow dynamics to predict aneurysm formation and rupture (68). Another survey by Futami et al. showed that the minimum WSS points were associated with wall instability in unruptured cerebral aneurysms (69). However, a study combining computational fluid dynamics (CFD) and in vitro particle velocimetry found that minor variations in the flow field and spatial resolution between modalities strongly influenced normalized WSS distributions (70), suggesting that further multimodality analysis to validate the clinical feasibility of hemodynamic parameters may be a future research trend.
The quantification of hemodynamics and the analysis of flow components in multiple pathophysiological states are the main research hotspots in the cardiac field. Representative keywords are “bicuspid aortic valve”, “diastolic function”, “heart failure”, and “atrial fibrillation”. Previous studies have shown that bicuspid aortic valve (BAV) is associated with complex, disturbed blood flow dynamics compared to participants with normal tricuspid aortic valves, even in the absence of aortic valve disease or aortic dilatation (21). Notably, the concept of “patient-specific heat maps” has been extensively applied to the aorta and is gradually extending to BAV patients (33,71), for whom WSS heat maps are generated, showing areas of increased or decreased WSS according to keyword clustering analysis (Table 6). A prospective study involving 20 BAV patients showed the consistency between a heat map increase in WSS (>95% of normal values) and histological wall damage (greater medial elastin degradation) (31). The creation of a WSS map is essential because it allows for the identification of abnormalities linked to different pathological conditions by comparing an individual’s WSS distribution with a healthy baseline. Furthermore, this approach contributes to the advancement of clinical research by establishing benchmarks for the study of blood flow dynamics, ultimately improving our understanding of the clinical significance of WSS in relation to various illnesses. However, 4D flow MRI-derived flow parameters are still in an exploratory stage, and there is no agreement on their normal values. This is an essential topic that will be addressed in future research.
The research hotspots for the portal system are categorized as Cluster 3, with representative keywords such as “portal hypertension”, “cirrhosis”, and “portal vein”. Over the past decade, 4D flow MRI techniques have shown great promise in visualizing vascular morphology and defining hemodynamic features in the cirrhotic population. A growing body of evidence supports the feasibility and robust internal consistency of 4D flow MRI in measuring hemodynamics in patients with cirrhosis (22,72,73). In addition, the flow parameters determined by 4D flow have been reported to be predictive of the prevalence and severity of liver cirrhosis and portal hypertension (50). In a study recruiting participants who were scheduled for both 4D flow MRI and gastroesophageal endoscopy, Motosugi et al. proposed the fractional flow change (FFC) index to quantify shunting from the portal vein and splenic vein to the varices, and a value of less than 0 is associated with endoscopic evaluation of high-risk esophageal varices (74). These findings suggest that parameters-derived 4D flow MRI could be useful in assessing the likelihood of ruptured esophageal varices and risk stratification. More basic research is needed to establish the specific mechanism of altered hemodynamic parameters associated with cirrhosis from a pathophysiological standpoint.
Emerging trends and frontiers
The burst analysis of keywords reveals the emerging trends related to 4D flow MRI. As illustrated in Figure 8, the research focus of 4D flow MRI has advanced from flow visualization (“visualization”, “velocity mapping”, “healthy volunteer”, “reproducibility”, and “angiography”) to preliminary clinical application in various vascular regions of the body (“cerebral aneurysm”, “heart”, “aorta”, “great vessel” and “tetralogy of Fallot”). However, many aspects of 4D flow MRI have yet to be explored.
Firstly, there is still much room in speeding up data acquisition of 4D flow MRI. Although several accelerated imaging approaches, such as non-Cartesian sampling, have been adopted for 4D flow MRI, imaging is still relatively time-consuming, and additional acceleration is expected as sequences evolve. Second, as indicated by the keywords “pattern”, “parameter”, and “fluid dynamics”, the analysis of blood flow patterns and components in diverse clinical contexts remains a popular research area. The concept of creating a WSS heat mapping across individuals and patient cohorts has recently been validated and is mostly limited to the aorta (71). There is still a lack of uniform standards for baseline values in different organs, and multicenter studies across countries are required to establish confidence intervals and the robustness of relevant parameters. Thirdly, “stiffness” has been a focus of attention over the past 4 years. Aortic stiffness assessment utilizing PWV derived from 4D flow MRI can reflect the severity of arterial dysfunction, and its feasibility has been demonstrated through in vivo models (75-79). More basic studies are required to understand the underlying mechanisms by which these imaging indicators reflect microscopic vascular alterations. Furthermore, multimodal imaging is predicted to emerge as a new trend in the field’s development (80,81). For instance, integrating 4D flow MRI and the liver stiffness assessed by magnetic resonance elastography to elucidate the inherent link between the hemodynamic properties of the portal vein and the liver stiffness in patients with chronic liver disease.
Limitations
There were several limitations to this study. Firstly, due to database variation in the CiteSpace software’s bibliometric analysis, only the WoSCC database was examined, while other important databases from PubMed or Google were ignored. As a result, the articles found may not be completely representative of all 4D flow MRI research. Second, CiteSpace was used to perform a general assessment of the present state of 4D flow imaging. Since full texts were not reviewed, some details might have been missed. Third, the retrieved articles were exclusively published in English, resulting in some linguistic bias.
Conclusions
We identified the overall scientific output of 4D flow MRI research from 2003 to 2022. The main directions for 4D flow MRI research are as follows: (I) the research hotspots remain in optimizing the 4D data acquisition scheme and exploring its applicability in different clinical settings, with keywords primarily including “visualization”, “resolution”, “brain”, “tetralogy of Fallot”, and “portal hypertension”; (II) multicenter collaborations are required to establish consensus or guidelines for determining the normal range of parameters derived from 4D flow MRI and to verify their robustness; (III) it was also noted that the research focus is gradually shifting from flow quantification to the analysis of microscopic vascular changes, such as “WSS” and “PWV”; (IV) the use of multimodality imaging will be crucial in the clinical evaluation and risk stratification in some certain diseases.
Acknowledgments
Funding: None.
Footnote
Conflicts of Interest: All authors have completed the ICMJE uniform disclosure form (available at https://qims.amegroups.com/article/view/10.21037/qims-23-1227/coif). The authors have no conflicts of interest to declare.
Ethical Statement: The authors are accountable for all aspects of the work in ensuring that questions related to the accuracy or integrity of any part of the work are appropriately investigated and resolved. Institutional Review Board approval was not required because our study was a bibliometric analysis that used only publicly available data.
Open Access Statement: This is an Open Access article distributed in accordance with the Creative Commons Attribution-NonCommercial-NoDerivs 4.0 International License (CC BY-NC-ND 4.0), which permits the non-commercial replication and distribution of the article with the strict proviso that no changes or edits are made and the original work is properly cited (including links to both the formal publication through the relevant DOI and the license). See: https://creativecommons.org/licenses/by-nc-nd/4.0/.
References
- Rivera-Rivera LA, Turski P, Johnson KM, Hoffman C, Berman SE, Kilgas P, Rowley HA, Carlsson CM, Johnson SC, Wieben O. 4D flow MRI for intracranial hemodynamics assessment in Alzheimer's disease. J Cereb Blood Flow Metab 2016;36:1718-30. [Crossref] [PubMed]
- Bae YJ, Jung C, Kim JH, Choi BS, Kim E. Quantitative Magnetic Resonance Angiography in Internal Carotid Artery Occlusion with Primary Collateral Pathway. J Stroke 2015;17:320-6. [Crossref] [PubMed]
- Spain MG, Smith MD, Grayburn PA, Harlamert EA, DeMaria AN. Quantitative assessment of mitral regurgitation by Doppler color flow imaging: angiographic and hemodynamic correlations. J Am Coll Cardiol 1989;13:585-90. [Crossref] [PubMed]
- Heydari B, Kwong RY, Jerosch-Herold M. Technical advances and clinical applications of quantitative myocardial blood flow imaging with cardiac MRI. Prog Cardiovasc Dis 2015;57:615-22. [Crossref] [PubMed]
- Palaniyappan N, Cox E, Bradley C, Scott R, Austin A, O'Neill R, Ramjas G, Travis S, White H, Singh R, Thurley P, Guha IN, Francis S, Aithal GP. Non-invasive assessment of portal hypertension using quantitative magnetic resonance imaging. J Hepatol 2016;65:1131-9. [Crossref] [PubMed]
- Soulat G, McCarthy P, Markl M. 4D Flow with MRI. Annu Rev Biomed Eng 2020;22:103-26. [Crossref] [PubMed]
- Rizk J. 4D flow MRI applications in congenital heart disease. Eur Radiol 2021;31:1160-74. [Crossref] [PubMed]
- Hyodo R, Takehara Y, Naganawa S. 4D Flow MRI in the portal venous system: imaging and analysis methods, and clinical applications. Radiol Med 2022;127:1181-98. [Crossref] [PubMed]
- Moran PR. A flow velocity zeugmatographic interlace for NMR imaging in humans. Magn Reson Imaging 1982;1:197-203. [Crossref] [PubMed]
- Firmin DN, Nayler GL, Klipstein RH, Underwood SR, Rees RS, Longmore DB. In vivo validation of MR velocity imaging. J Comput Assist Tomogr 1987;11:751-6. [Crossref] [PubMed]
- Pradella M, Scott MB, Omer M, Hill SK, Lockhart L, Yi X, Amir-Khalili A, Sojoudi A, Allen BD, Avery R, Markl M. Fully-automated deep learning-based flow quantification of 2D CINE phase contrast MRI. Eur Radiol 2023;33:1707-18. [Crossref] [PubMed]
- Bissell MM, Raimondi F, Ait Ali L, Allen BD, Barker AJ, Bolger A, et al. 4D Flow cardiovascular magnetic resonance consensus statement: 2023 update. J Cardiovasc Magn Reson 2023;25:40. [Crossref] [PubMed]
- Markl M, Frydrychowicz A, Kozerke S, Hope M, Wieben O. 4D flow MRI. J Magn Reson Imaging 2012;36:1015-36. [Crossref] [PubMed]
- Brix L, Ringgaard S, Rasmusson A, Sørensen TS, Kim WY. Three dimensional three component whole heart cardiovascular magnetic resonance velocity mapping: comparison of flow measurements from 3D and 2D acquisitions. J Cardiovasc Magn Reson 2009;11:3. [Crossref] [PubMed]
- Frydrychowicz A, Wieben O, Niespodzany E, Reeder SB, Johnson KM, François CJ. Quantification of thoracic blood flow using volumetric magnetic resonance imaging with radial velocity encoding: in vivo validation. Invest Radiol 2013;48:819-25. [Crossref] [PubMed]
- Bollache E, van Ooij P, Powell A, Carr J, Markl M, Barker AJ. Comparison of 4D flow and 2D velocity-encoded phase contrast MRI sequences for the evaluation of aortic hemodynamics. Int J Cardiovasc Imaging 2016;32:1529-41. [Crossref] [PubMed]
- Oyre S, Paaske WP, Ringgaard S, Kozerke S, Erlandsen M, Boesiger P, Pedersen EM. Automatic accurate non-invasive quantitation of blood flow, cross-sectional vessel area, and wall shear stress by modelling of magnetic resonance velocity data. Eur J Vasc Endovasc Surg 1998;16:517-24. [Crossref] [PubMed]
- Markl M, Wallis W, Brendecke S, Simon J, Frydrychowicz A, Harloff A. Estimation of global aortic pulse wave velocity by flow-sensitive 4D MRI. Magn Reson Med 2010;63:1575-82. [Crossref] [PubMed]
- Tyszka JM, Laidlaw DH, Asa JW, Silverman JM. Three-dimensional, time-resolved (4D) relative pressure mapping using magnetic resonance imaging. J Magn Reson Imaging 2000;12:321-9. [Crossref] [PubMed]
- Dyverfeldt P, Kvitting JP, Sigfridsson A, Engvall J, Bolger AF, Ebbers T. Assessment of fluctuating velocities in disturbed cardiovascular blood flow: in vivo feasibility of generalized phase-contrast MRI. J Magn Reson Imaging 2008;28:655-63. [Crossref] [PubMed]
- Hope MD, Hope TA, Meadows AK, Ordovas KG, Urbania TH, Alley MT, Higgins CB. Bicuspid aortic valve: four-dimensional MR evaluation of ascending aortic systolic flow patterns. Radiology 2010;255:53-61. [Crossref] [PubMed]
- Roldán-Alzate A, Frydrychowicz A, Niespodzany E, Landgraf BR, Johnson KM, Wieben O, Reeder SB. In vivo validation of 4D flow MRI for assessing the hemodynamics of portal hypertension. J Magn Reson Imaging 2013;37:1100-8. [Crossref] [PubMed]
- Galizia MS, Barker A, Liao Y, Collins J, Carr J, McDermott MM, Markl M. Wall morphology, blood flow and wall shear stress: MR findings in patients with peripheral artery disease. Eur Radiol 2014;24:850-6. [Crossref] [PubMed]
- Kvitting JP, Ebbers T, Wigström L, Engvall J, Olin CL, Bolger AF. Flow patterns in the aortic root and the aorta studied with time-resolved, 3-dimensional, phase-contrast magnetic resonance imaging: implications for aortic valve-sparing surgery. J Thorac Cardiovasc Surg 2004;127:1602-7. [Crossref] [PubMed]
- Guler AT, Waaijer CJ, Palmblad M. Scientific workflows for bibliometrics. Scientometrics 2016;107:385-98. [Crossref] [PubMed]
- Li Y, Gao Q, Chen N, Zhang Y, Wang J, Li C, He X, Jiao Y, Zhang Z. Clinical studies of magnetic resonance elastography from 1995 to 2021: Scientometric and visualization analysis based on CiteSpace. Quant Imaging Med Surg 2022;12:5080-100. [Crossref] [PubMed]
- Wen R, Zhang M, Xu R, Gao Y, Liu L, Chen H, Wang X, Zhu W, Lin H, Liu C, Zeng X. COVID-19 imaging, where do we go from here? Bibliometric analysis of medical imaging in COVID-19. Eur Radiol 2023;33:3133-43. [Crossref] [PubMed]
- Chen C, Hu Z, Liu S, Tseng H. Emerging trends in regenerative medicine: a scientometric analysis in CiteSpace. Expert Opin Biol Ther 2012;12:593-608. [Crossref] [PubMed]
- Synnestvedt MB, Chen C, Holmes JH. CiteSpace II: visualization and knowledge discovery in bibliographic databases. AMIA Annu Symp Proc 2005;2005:724-8.
- Dyverfeldt P, Bissell M, Barker AJ, Bolger AF, Carlhäll CJ, Ebbers T, Francios CJ, Frydrychowicz A, Geiger J, Giese D, Hope MD, Kilner PJ, Kozerke S, Myerson S, Neubauer S, Wieben O, Markl M. 4D flow cardiovascular magnetic resonance consensus statement. J Cardiovasc Magn Reson 2015;17:72. [Crossref] [PubMed]
- Guzzardi DG, Barker AJ, van Ooij P, Malaisrie SC, Puthumana JJ, Belke DD, Mewhort HE, Svystonyuk DA, Kang S, Verma S, Collins J, Carr J, Bonow RO, Markl M, Thomas JD, McCarthy PM, Fedak PW. Valve-Related Hemodynamics Mediate Human Bicuspid Aortopathy: Insights From Wall Shear Stress Mapping. J Am Coll Cardiol 2015;66:892-900. [Crossref] [PubMed]
- Markl M, Kilner PJ, Ebbers T. Comprehensive 4D velocity mapping of the heart and great vessels by cardiovascular magnetic resonance. J Cardiovasc Magn Reson 2011;13:7. [Crossref] [PubMed]
- Rodríguez-Palomares JF, Dux-Santoy L, Guala A, Kale R, Maldonado G, Teixidó-Turà G, Galian L, Huguet M, Valente F, Gutiérrez L, González-Alujas T, Johnson KM, Wieben O, García-Dorado D, Evangelista A. Aortic flow patterns and wall shear stress maps by 4D-flow cardiovascular magnetic resonance in the assessment of aortic dilatation in bicuspid aortic valve disease. J Cardiovasc Magn Reson 2018;20:28. [Crossref] [PubMed]
- Potters WV, van Ooij P, Marquering H, vanBavel E, Nederveen AJ. Volumetric arterial wall shear stress calculation based on cine phase contrast MRI. J Magn Reson Imaging 2015;41:505-16. [Crossref] [PubMed]
- Barker AJ, Markl M, Bürk J, Lorenz R, Bock J, Bauer S, Schulz-Menger J, von Knobelsdorff-Brenkenhoff F. Bicuspid aortic valve is associated with altered wall shear stress in the ascending aorta. Circ Cardiovasc Imaging 2012;5:457-66. [Crossref] [PubMed]
- Garcia J, Barker AJ, Markl M. The Role of Imaging of Flow Patterns by 4D Flow MRI in Aortic Stenosis. JACC Cardiovasc Imaging 2019;12:252-66. [Crossref] [PubMed]
- Mahadevia R, Barker AJ, Schnell S, Entezari P, Kansal P, Fedak PW, Malaisrie SC, McCarthy P, Collins J, Carr J, Markl M. Bicuspid aortic cusp fusion morphology alters aortic three-dimensional outflow patterns, wall shear stress, and expression of aortopathy. Circulation 2014;129:673-82. [Crossref] [PubMed]
- Schnell S, Ansari SA, Wu C, Garcia J, Murphy IG, Rahman OA, Rahsepar AA, Aristova M, Collins JD, Carr JC, Markl M. Accelerated dual-venc 4D flow MRI for neurovascular applications. J Magn Reson Imaging 2017;46:102-14. [Crossref] [PubMed]
- Bissell MM, Hess AT, Biasiolli L, Glaze SJ, Loudon M, Pitcher A, Davis A, Prendergast B, Markl M, Barker AJ, Neubauer S, Myerson SG. Aortic dilation in bicuspid aortic valve disease: flow pattern is a major contributor and differs with valve fusion type. Circ Cardiovasc Imaging 2013;6:499-507. [Crossref] [PubMed]
- Ma LE, Markl M, Chow K, Huh H, Forman C, Vali A, Greiser A, Carr J, Schnell S, Barker AJ, Jin N. Aortic 4D flow MRI in 2 minutes using compressed sensing, respiratory controlled adaptive k-space reordering, and inline reconstruction. Magn Reson Med 2019;81:3675-90. [Crossref] [PubMed]
- Stankovic Z, Allen BD, Garcia J, Jarvis KB, Markl M. 4D flow imaging with MRI. Cardiovasc Diagn Ther 2014;4:173-92. [Crossref] [PubMed]
- van Ooij P, Powell AL, Potters WV, Carr JC, Markl M, Barker AJ. Reproducibility and interobserver variability of systolic blood flow velocity and 3D wall shear stress derived from 4D flow MRI in the healthy aorta. J Magn Reson Imaging 2016;43:236-48. [Crossref] [PubMed]
- Azarine A, Garçon P, Stansal A, Canepa N, Angelopoulos G, Silvera S, Sidi D, Marteau V, Zins M. Four-dimensional Flow MRI: Principles and Cardiovascular Applications. Radiographics 2019;39:632-48. [Crossref] [PubMed]
- Firmin DN, Gatehouse PD, Konrad JP, Yang GZ, Kilner PJ, Longmore DB. Rapid 7-dimensional imaging of pulsatile flow. London, UK: Proceedings of Computers in Cardiology Conference, 1993: 353-356.
- Hope MD, Sigovan M, Wrenn SJ, Saloner D, Dyverfeldt P. MRI hemodynamic markers of progressive bicuspid aortic valve-related aortic disease. J Magn Reson Imaging 2014;40:140-5. [Crossref] [PubMed]
- Markl M, Wallis W, Harloff A. Reproducibility of flow and wall shear stress analysis using flow-sensitive four-dimensional MRI. J Magn Reson Imaging 2011;33:988-94. [Crossref] [PubMed]
- Petersson S, Sigfridsson A, Dyverfeldt P, Carlhäll CJ, Ebbers T. Retrospectively gated intracardiac 4D flow MRI using spiral trajectories. Magn Reson Med 2016;75:196-206. [Crossref] [PubMed]
- Gu T, Korosec FR, Block WF, Fain SB, Turk Q, Lum D, Zhou Y, Grist TM, Haughton V, Mistretta CA PC. VIPR: a high-speed 3D phase-contrast method for flow quantification and high-resolution angiography. AJNR Am J Neuroradiol 2005;26:743-9.
- Dyvorne H, Knight-Greenfield A, Jajamovich G, Besa C, Cui Y, Stalder A, Markl M, Taouli B. Abdominal 4D flow MR imaging in a breath hold: combination of spiral sampling and dynamic compressed sensing for highly accelerated acquisition. Radiology 2015;275:245-54. [Crossref] [PubMed]
- Bane O, Peti S, Wagner M, Hectors S, Dyvorne H, Markl M, Taouli B. Hemodynamic measurements with an abdominal 4D flow MRI sequence with spiral sampling and compressed sensing in patients with chronic liver disease. J Magn Reson Imaging 2019;49:994-1005. [Crossref] [PubMed]
- Tariq U, Hsiao A, Alley M, Zhang T, Lustig M, Vasanawala SS. Venous and arterial flow quantification are equally accurate and precise with parallel imaging compressed sensing 4D phase contrast MRI. J Magn Reson Imaging 2013;37:1419-26. [Crossref] [PubMed]
- Callahan S, Singam NS, Kendrick M, Negahdar MJ, Wang H, Stoddard MF, Amini AA. Dual-V(enc) acquisition for 4D flow MRI in aortic stenosis with spiral readouts. J Magn Reson Imaging 2020;52:117-28. [Crossref] [PubMed]
- Oechtering TH, Nowak A, Sieren MM, Stroth AM, Kirschke N, Wegner F, Balks M, König IR, Jin N, Graessner J, Kooijman-Kurfuerst H, Hennemuth A, Barkhausen J, Frydrychowicz A. Repeatability and reproducibility of various 4D Flow MRI postprocessing software programs in a multi-software and multi-vendor cross-over comparison study. J Cardiovasc Magn Reson 2023;25:22. [Crossref] [PubMed]
- Kamphuis VP, Westenberg JJM, van der Palen RLF, Blom NA, de Roos A, van der Geest R, Elbaz MSM, Roest AAW. Unravelling cardiovascular disease using four dimensional flow cardiovascular magnetic resonance. Int J Cardiovasc Imaging 2017;33:1069-81. [Crossref] [PubMed]
- Sjöberg P, Bidhult S, Bock J, Heiberg E, Arheden H, Gustafsson R, Nozohoor S, Carlsson M. Disturbed left and right ventricular kinetic energy in patients with repaired tetralogy of Fallot: pathophysiological insights using 4D-flow MRI. Eur Radiol 2018;28:4066-76. [Crossref] [PubMed]
- Garg P, Crandon S, Swoboda PP, Fent GJ, Foley JRJ, Chew PG, Brown LAE, Vijayan S, Hassell MECJ, Nijveldt R, Bissell M, Elbaz MSM, Al-Mohammad A, Westenberg JJM, Greenwood JP, van der Geest RJ, Plein S, Dall'Armellina E. Left ventricular blood flow kinetic energy after myocardial infarction - insights from 4D flow cardiovascular magnetic resonance. J Cardiovasc Magn Reson 2018;20:61. [Crossref] [PubMed]
- Bürk J, Blanke P, Stankovic Z, Barker A, Russe M, Geiger J, Frydrychowicz A, Langer M, Markl M. Evaluation of 3D blood flow patterns and wall shear stress in the normal and dilated thoracic aorta using flow-sensitive 4D CMR. J Cardiovasc Magn Reson 2012;14:84. [Crossref] [PubMed]
- Jou LD, Lee DH, Morsi H, Mawad ME. Wall shear stress on ruptured and unruptured intracranial aneurysms at the internal carotid artery. AJNR Am J Neuroradiol 2008;29:1761-7. [Crossref] [PubMed]
- Hofman MB, Visser FC, van Rossum AC, Vink QM, Sprenger M, Westerhof N. In vivo validation of magnetic resonance blood volume flow measurements with limited spatial resolution in small vessels. Magn Reson Med 1995;33:778-84. [Crossref] [PubMed]
- Ha H, Kim GB, Kweon J, Lee SJ, Kim YH, Lee DH, Yang DH, Kim N. Hemodynamic Measurement Using Four-Dimensional Phase-Contrast MRI: Quantification of Hemodynamic Parameters and Clinical Applications. Korean J Radiol 2016;17:445-62. [Crossref] [PubMed]
- Jiang J, Kokeny P, Ying W, Magnano C, Zivadinov R, Mark Haacke E. Quantifying errors in flow measurement using phase contrast magnetic resonance imaging: comparison of several boundary detection methods. Magn Reson Imaging 2015;33:185-93. [Crossref] [PubMed]
- Long D, McMurdo C, Ferdian E, Mauger CA, Marlevi D, Nash MP, Young AA. Super-resolution 4D flow MRI to quantify aortic regurgitation using computational fluid dynamics and deep learning. Int J Cardiovasc Imaging 2023;39:1189-202. [Crossref] [PubMed]
- Vishnevskiy V, Walheim J, Kozerke S. Deep variational network for rapid 4D flow MRI reconstruction. Nature Machine Intelligence 2020;2:228-35.
- Berhane H, Scott M, Elbaz M, Jarvis K, McCarthy P, Carr J, Malaisrie C, Avery R, Barker AJ, Robinson JD, Rigsby CK, Markl M. Fully automated 3D aortic segmentation of 4D flow MRI for hemodynamic analysis using deep learning. Magn Reson Med 2020;84:2204-18. [Crossref] [PubMed]
- Bammer R, Hope TA, Aksoy M, Alley MT. Time-resolved 3D quantitative flow MRI of the major intracranial vessels: initial experience and comparative evaluation at 1.5T and 3.0T in combination with parallel imaging. Magn Reson Med 2007;57:127-40. [Crossref] [PubMed]
- Yamashita S, Isoda H, Hirano M, Takeda H, Inagawa S, Takehara Y, Alley MT, Markl M, Pelc NJ, Sakahara H. Visualization of hemodynamics in intracranial arteries using time-resolved three-dimensional phase-contrast MRI. J Magn Reson Imaging 2007;25:473-8. [Crossref] [PubMed]
- Schnell S, Wu C, Ansari SA. Four-dimensional MRI flow examinations in cerebral and extracerebral vessels - ready for clinical routine? Curr Opin Neurol 2016;29:419-28. [Crossref] [PubMed]
- Zhang M, Peng F, Tong X, Feng X, Li Y, Chen H, Niu H, Zhang B, Song G, Li Y, Liu P, Liu A, Li R. Associations between haemodynamics and wall enhancement of intracranial aneurysm. Stroke Vasc Neurol 2021;6:467-75. [Crossref] [PubMed]
- Futami K, Misaki K, Uno T, Nambu I, Tsutsui T, Kamide T, Nakada M. Minimum wall shear stress points and their underlying intra-aneurysmal flow structures of unruptured cerebral aneurysms on 4D flow MRI. J Neuroradiol 2023;50:302-8. [Crossref] [PubMed]
- Brindise MC, Rothenberger S, Dickerhoff B, Schnell S, Markl M, Saloner D, Rayz VL, Vlachos PP. Multi-modality cerebral aneurysm haemodynamic analysis: in vivo 4D flow MRI, in vitro volumetric particle velocimetry and in silico computational fluid dynamics. J R Soc Interface 2019;16:20190465. [Crossref] [PubMed]
- van Ooij P, Potters WV, Nederveen AJ, Allen BD, Collins J, Carr J, Malaisrie SC, Markl M, Barker AJ. A methodology to detect abnormal relative wall shear stress on the full surface of the thoracic aorta using four-dimensional flow MRI. Magn Reson Med 2015;73:1216-27. [Crossref] [PubMed]
- Brunsing RL, Brown D, Almahoud H, Kono Y, Loomba R, Vodkin I, Sirlin CB, Alley MT, Vasanawala SS, Hsiao A. Quantification of the Hemodynamic Changes of Cirrhosis with Free-Breathing Self-Navigated MRI. J Magn Reson Imaging 2021;53:1410-21. [Crossref] [PubMed]
- Stankovic Z, Csatari Z, Deibert P, Euringer W, Blanke P, Kreisel W, Abdullah Zadeh Z, Kallfass F, Langer M, Markl M. Normal and altered three-dimensional portal venous hemodynamics in patients with liver cirrhosis. Radiology 2012;262:862-73. [Crossref] [PubMed]
- Motosugi U, Roldán-Alzate A, Bannas P, Said A, Kelly S, Zea R, Wieben O, Reeder SB. Four-dimensional Flow MRI as a Marker for Risk Stratification of Gastroesophageal Varices in Patients with Liver Cirrhosis. Radiology 2019;290:101-7. [Crossref] [PubMed]
- Houriez-Gombaud-Saintonge S, Mousseaux E, Bargiotas I, De Cesare A, Dietenbeck T, Bouaou K, Redheuil A, Soulat G, Giron A, Gencer U, Craiem D, Messas E, Bollache E, Chenoune Y, Kachenoura N. Comparison of different methods for the estimation of aortic pulse wave velocity from 4D flow cardiovascular magnetic resonance. J Cardiovasc Magn Reson 2019;21:75. [Crossref] [PubMed]
- Jarvis K, Scott MB, Soulat G, Elbaz MSM, Barker AJ, Carr JC, Markl M, Ragin A. Aortic Pulse Wave Velocity Evaluated by 4D Flow MRI Across the Adult Lifespan. J Magn Reson Imaging 2022;56:464-73. [Crossref] [PubMed]
- Kenyhercz WE, Raterman B, Illapani VS, Dowell J, Mo X, White RD, Kolipaka A. Quantification of aortic stiffness using magnetic resonance elastography: Measurement reproducibility, pulse wave velocity comparison, changes over cardiac cycle, and relationship with age. Magn Reson Med 2016;75:1920-6. [Crossref] [PubMed]
- Ruiz-Muñoz A, Guala A, Rodriguez-Palomares J, Dux-Santoy L, Servato L, Lopez-Sainz A, La Mura L, Granato C, Limeres J, Gonzalez-Alujas T, Galián-Gay L, Gutiérrez L, Johnson K, Wieben O, Sao-Aviles A, Ferreira-Gonzalez I, Evangelista A, Teixido-Tura G. Aortic flow dynamics and stiffness in Loeys-Dietz syndrome patients: a comparison with healthy volunteers and Marfan syndrome patients. Eur Heart J Cardiovasc Imaging 2022;23:641-9. [Crossref] [PubMed]
- Rivera-Rivera LA, Cody KA, Eisenmenger L, Cary P, Rowley HA, Carlsson CM, Johnson SC, Johnson KM. Assessment of vascular stiffness in the internal carotid artery proximal to the carotid canal in Alzheimer's disease using pulse wave velocity from low rank reconstructed 4D flow MRI. J Cereb Blood Flow Metab 2021;41:298-311. [Crossref] [PubMed]
- Galian-Gay L, Rodríguez-Palomares J, Guala A, Michelena HI, Evangelista A. Multimodality imaging in bicuspid aortic valve. Prog Cardiovasc Dis 2020;63:442-51. [Crossref] [PubMed]
- Kolipaka A, Illapani VS, Kalra P, Garcia J, Mo X, Markl M, White RD. Quantification and comparison of 4D-flow MRI-derived wall shear stress and MRE-derived wall stiffness of the abdominal aorta. J Magn Reson Imaging 2017;45:771-8. [Crossref] [PubMed]