Preoperative surgical planning for biventricular repair of double outlet right ventricle by using a 3D heart model
Introduction
Double outlet right ventricle (DORV) is an abnormal ventriculoarterial connection in which both great arteries (GAs) are connected to the morphologic RV. This congenital heart disease (CHD) is characterized by extreme diversity regarding ventricular septal defect (VSD), atrioventricular connection, ventricular morphology, and GA relationship (1). The preferred method of treatment is surgical by achieving a near-normal biventricular cardiac circulation.
Traditionally, preoperative imaging consists of two-dimensional (2D) transthoracic echocardiography (TTE) and transesophageal echocardiography (TOE). It is, however, a 2D image and requires a mental reconstruction of the three-dimensional (3D) heart anatomy. Computed tomography (CT) is also routinely used for the assessment of DORV, though it provides us only with static images, due to its significance in extracting raw data for the 3D reconstruction of the whole heart (2). Cardiac magnetic resonance (CMR), is gaining ground as an imaging technique capable of providing clear images of the heart and accurate measurements of intra-cardiac shunts and ventricular volumes.
We describe a patient with a DORV in which all these imaging techniques have been used in combination with dedicated 3D printing software.
Case presentation
An 11-month-old patient with prenatal diagnosis of DORV with normally related great arteries (GAs) status post-pulmonary artery (PA) band at 16-days-of-life, was evaluated for feasibility of biventricular repair. Postnatal TTE demonstrated a large subaortic VSD, tricuspid chordal attachments to the crest of the interventricular septum (IVS) and a borderline right ventricle (RV) with a hypoplastic inlet component (Figure 1). Cross-sectional imaging via CT and CMR confirmed (Figure 2) the diagnosis and allowed for better visualization of the hypoplastic inlet portion of the RV and the anatomic relationship of the VSD with the GA. CMR volumetry revealed a left ventricle (LV):RV ratio of approximately 2:1.
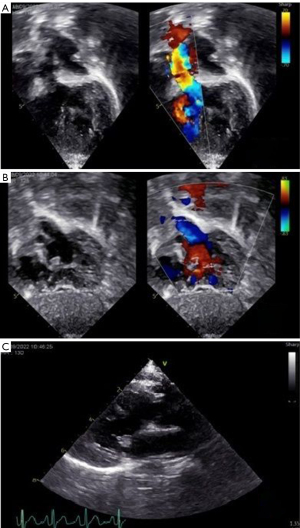
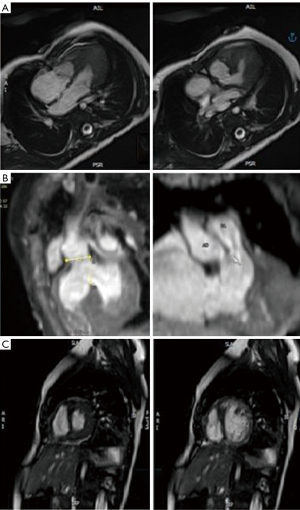
Cross-sectional imaging information combined by CT and CMR were incorporated into a dedicated 3D image processing software (Figures 3,4). Ventricular volumes obtained and the extracted 3D model helped the surgical team make an accurate plan for repair. All procedures performed in this study were in accordance with the ethical standards of the institutional and/or national research committees and with the Helsinki Declaration (as revised in 2013). Written informed consent was obtained from the patient’s parents for publication of this case report and accompanying images. A copy of the written consent is available for review by the editorial office of this journal.
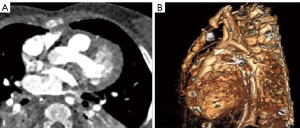
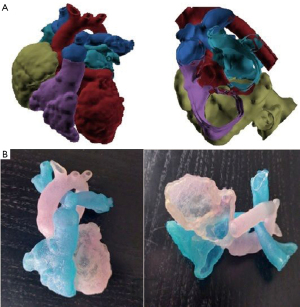
Decision was to proceed with a biventricular repair as follows: first re-attach the anomalous tricuspid valve cords to the right aspect of the IVS, then divide the hypertrophic muscle bundles of the inlet portion and place the surgical patch in a way that secured more volume to the RV. Eventually, the repair was performed as planned with the addition of PA plasty following debanding of the PA. A 4 mm atrial septal communication was deliberately left for potential decompression of the RV. Postoperative course was uneventful and patient was discharged home on postoperative day 10. The feedback for the surgeon was that the 3D model was very accurate and helpful.
Discussion
In cases such as DORV, it is of paramount importance for the imaging to be as accurate as possible. Surgical outcome is irrevocably connected to the preparation of the surgical team with images as close to reality as possible (3).
3D imaging and printing of the whole heart seems to be a new milestone for the surgery of DORV. This was achieved by using data from CMR and CT and reconstructing a 3D model, which provides information such as baffle size in relation to the GAs, muscle bundle location and atrioventricular valve anatomy. More studies are needed in this direction, though there are limitations due to the comparatively low number of patients and the extreme diversity of the disease (4).
These 3D data can be used by specialized software and simulate the surgery in virtual reality, making it possible to plan, trim, and edit the baffle patch as well as exporting the result in 3D images and print. This allows us to study the patient’s anatomy and plan for the optimal surgical design (5).
CT showed us the great vessel anatomy and offered us the raw data for the 3D printing. CMR allowed us to study the hypoplastic RV morphology in order the biventricular repair to be feasible. The arrangement of the arterial roots is such that the distance between the tricuspid and pulmonary valve measures (17 mm) almost equal to the diameter of the aortic root (16 mm). When this criterion is satisfied, it is usually possible to create the required unrestrictive tunnel to the aortic root without causing any obstruction to the residual connection between RV and pulmonary valve according to the suggested literature (6). With the aid of 3D printing and its software analysis confirmed the subaortic type of the DORV, thus making the septation plane optimal by indicating the location of the cutting point of the muscle bundles in the inlet portion of the RV, allowing for near-optimal size of the RV (Figure 5).
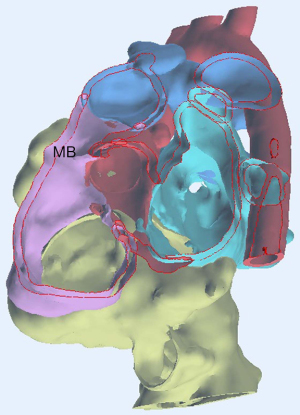
The 3D measurements of the distance from the aorta to the crest of the septum were different from the 2D measurements. This clearly shows that the 3D measurements were more accurate and it is a valuable tool for patch planning in cases for DORV correction.
3D printing allowed the heart team to have access to the anatomy of DORV and provided insights and a multidisciplinary approach.
Lastly, specialized software for 3D reconstruction helped the experienced surgeons to be prepared of the patient’s specific anatomy, while for juniors it became an irreplaceable tool for surgical training. Surgeons of varying expertise are to be benefited from the use of such software, though it can by no means replace live patient surgical experience (7,8).
The automatic reconstruction and segmentation of the hearts was conducted employing various open-source libraries in Python. In addition, the VR environment was developed via the Unity platform. Stratasys 3D Printer Connex Objet 260 is a latest technology 3D printer with 16 micro precision has been used for better anatomical model accuracy and presentation, able to print in a variety of colors. A latest technology software (Materialise Mimics Software) has also been used for segmentation and digital model preparation. 3D life uses resin and acrylonitrile butadiene styrene (ABS)-based 3D models. Resin can be created as a soft and hard material, according to the color that the 3D model will have and the anatomic structures that should be demonstrated. The darker the color, the harder the material is (9).
Conclusions
Accurate 3D heart models derived from cross-sectional imaging data combined with preoperative imaging can assist in the surgical planning for DORV repair. Imaging techniques, no matter how advanced, need proficient doctors to comprehend them and apply them in practice.
Acknowledgments
The 3D model was developed in the frames of the 3D4Kardia Project that has been co-financed by the European Union and Greek national funds through the Operational Program Competitiveness, Entrepreneurship and Innovation, under the call RESEARCH-CREATE-INNOVATE (project code: T2EDK-04012). The authors would like to thank Paschalis Charalampous, Ioannis Kostavelis, Dimitrios Tzovaras [Centre for Research and Technology Hellas, Information Technologies Institute (CERTH/ITI)] and Gregory Krinos, George Tzanakis (3D LIfe GR) for their collaboration in the frames of the 3D4Kardia. This case was presented as a poster in ECHSA Annual Meeting 2023, 20–22 April 2023, Athens-Greece.
Funding: None.
Footnote
Conflicts of Interest: All authors have completed the ICMJE uniform disclosure form (available at https://qims.amegroups.com/article/view/10.21037/qims-23-1191/coif). The authors have no conflicts of interest to declare.
Ethical Statement: The authors are accountable for all aspects of the work in ensuring that questions related to the accuracy or integrity of any part of the work are appropriately investigated and resolved. All procedures performed in this study were in accordance with the ethical standards of the institutional and/or national research committees and with the Helsinki Declaration (as revised in 2013). Written informed consent was obtained from the patient’s parents for publication of this case report and accompanying images. A copy of the written consent is available for review by the editorial office of this journal.
Open Access Statement: This is an Open Access article distributed in accordance with the Creative Commons Attribution-NonCommercial-NoDerivs 4.0 International License (CC BY-NC-ND 4.0), which permits the non-commercial replication and distribution of the article with the strict proviso that no changes or edits are made and the original work is properly cited (including links to both the formal publication through the relevant DOI and the license). See: https://creativecommons.org/licenses/by-nc-nd/4.0/.
References
- Goo HW. Double Outlet Right Ventricle: In-Depth Anatomic Review Using Three-Dimensional Cardiac CT Data. Korean J Radiol 2021;22:1894-908. [Crossref] [PubMed]
- Priya S, Nagpal P, Sharma A, Pandey NN, Jagia P. Imaging Spectrum of Double-Outlet Right Ventricle on Multislice Computed Tomography. J Thorac Imaging 2019;34:W89-99. [Crossref] [PubMed]
- Villemain O, Belli E, Ladouceur M, Houyel L, Jalal Z, Lambert V, Ly M, Vouhé P, Bonnet D. Impact of anatomic characteristics and initial biventricular surgical strategy on outcomes in various forms of double-outlet right ventricle. J Thorac Cardiovasc Surg 2016;152:698-706.e3. [Crossref] [PubMed]
- Milano EG, Pajaziti E, Sauvage E, Cook A, Schievano S, Mortensen KH, Taylor AM, Marek J, Kostolny M, Capelli C. Taking Surgery Out of Reality. Circ Cardiovasc Imaging 2019;12:e009297. [Crossref] [PubMed]
- Liang J, Lu B, Zhao X, Wang J, Zhao D, Zhang G, Zhu B, Ma Q, Pan G, Li D. Feasibility analyses of virtual models and 3D printing for surgical simulation of the double-outlet right ventricle. Med Biol Eng Comput 2022;60:3029-40. [Crossref] [PubMed]
- Corno AF, Durairaj S, Skinner GJ. Narrative review of assessing the surgical options for double outlet right ventricle. Transl Pediatr 2021;10:165-76. [Crossref] [PubMed]
- Ong CS, Krishnan A, Huang CY, Spevak P, Vricella L, Hibino N, Garcia JR, Gaur L. Role of virtual reality in congenital heart disease. Congenit Heart Dis 2018;13:357-61. [Crossref] [PubMed]
- Charalampous P, Kladovasilakis N, Zoumaki M, Kostavelis I, Votis K, Petsios K, Tzetzis D, Tzovaras D. Examination of a Human Heart Fabricating Its 3D-Printed Cardiovascular Model and Employing Computational Technologies. Appl Sci 2023;13:10362.
- Yoo SJ, van Arsdell GS. 3D Printing in Surgical Management of Double Outlet Right Ventricle. Front Pediatr 2017;5:289. [Crossref] [PubMed]