A nomogram for predicting the risk of intra-abdominal hypertension in critically ill patients based on ultrasound and clinical data
Introduction
A normal intra-abdominal pressure (IAP) is between 5 and 7 mmHg. According to the updated consensus definitions and clinical practice guidelines published by the World Society of the Abdominal Compartment Society (WSACS) in 2017 (1), the risk factors for intra-abdominal hypertension/acute compartment syndrome (IAH/ACS) are the following: (I) diminished abdominal wall compliance, including prone position, major burns, post-abdominal surgery, and major trauma; (II) increased intra-luminal contents, including gastroparesis, gastric distention, ileus, volvulus, and colonic pseudo-obstruction; (III) increased intra-abdominal contents, including acute pancreatitis, intra-abdominal infection, peritoneal dialysis, hepatic insufficiency/cirrhosis with ascites, hemoperitoneum, pneumoperitoneum, and intraperitoneal or retroperitoneal tumors; and (IV) acidosis, hypothermia, massive fluid resuscitation, or positive fluid balance. IAH is defined as a sustained or repeated pathological elevation of IAP ≥12 mmHg and can be graded as follows: grade I, 12–15 mmHg; grade II, 16–20 mmHg; grade III, 21–25 mmHg; and grade IV, >25 mmHg. ACS is defined as IAP persisting at >20 mmHg (with or without an abdominal perfusion pressure <60 mmHg) that is associated with new organ dysfunction or failure (1,2).
IAH is more common than previously thought. In a prospective study of 40 patients with septic shock who received a volume resuscitation >5 L within 24 hours, IAH occurred in 34 patients (82.7%), and 10 patients (25%) developed ACS (3). A prospective observational study screened 491 patients in 15 intensive care units (ICUs) worldwide; the investigators found that 34% of patients developed IAH on the day of admission, and 48.9% of patients developed IAH during the observation period (4). These findings suggest monitoring high-risk IAP patients and intervening in IAH are critical to reducing ACS morbidity and mortality. There are various methods for measuring IAP, with cystometry being the gold standard recommended by the WSACS in 2009 (5). This method can objectively measure IAP; however, it requires a high level of patient cooperation and coughing avoidance, which may cause data variability. Furthermore, repeated catheterization for pressure measurement is time-consuming and labor-intensive and may cause urinary tract infections (6). Therefore, efficient and accurate measuring of IAP while minimizing iatrogenic harm remains challenging.
Elevated IAP affects many organs, including the kidneys and lungs. IAH may result in significant reduction in renal artery blood flow and increase in renal vein pressure and the renal vascular resistance, which can lead to impaired glomerular and tubular function (7). Pulmonary involvement can present as decreased thoracic volumes and elevated peak pressures from the compression of the diaphragm (8). Since the diaphragm and abdomen are arranged in series (9), any upward or downward motion of the diaphragm represents a sign of IAP change.
Ultrasound has been proven to be a valuable tool for observing organ morphology and blood flow, providing continuous and dynamic evaluation of various body and target organ pathologies. B-mode ultrasound and 2-dimensional shear wave elastography are widely employed by researchers worldwide to assess diaphragm function (10-12). Additionally, shear wave elastography has emerged as an effective technique for diagnosing renal fibrosis (13). Numerous studies have emphasized the prognostic significance of the renal resistive index (RRI) measured through Doppler ultrasound in kidney disease (14,15). Notably, a study conducted by Candan et al. (16) revealed that in comparison to the left RRI, the right RRI (RRRI) serves as an independent risk predictor for IAH. More recently, See et al. (17) introduced a novel, convenient, noninvasive IAP assessment technique for patients in the ICU, but the small sample size (n=21) limited the application in patients with ACS or IAP above 15 mmHg, thus rendering it valid only for grade I IAH. Therefore, this study aimed to noninvasively evaluate the kidneys and diaphragms of critically ill patients using ultrasound and incorporate clinical indicators to construct a nomogram to provide quantitative indicators for predicting IAH and facilitating timely intervention. We present this article in accordance with the STROBE reporting checklist (available at https://qims.amegroups.com/article/view/10.21037/qims-23-325/rc).
Methods
Patients
The study was performed on 89 patients hospitalized in the ICU of Zhangzhou Affiliated Hospital of Fujian Medical University, from February 2022 to June 2022. Among these patients, 44 had normal IAP and 45 were diagnosed with IAH. There were 73 males and 16 females, aged 29–90 years, with a quartile of 65.0 (range, 48.0, 77.5) years. The median (Q25, Q75) of IAP measured in 89 patients was 12 (Q25, Q75: 9, 16) mmHg (Table 1). The study was conducted in accordance with the Declaration of Helsinki (as revised in 2013), and its protocol was approved by the ethics committee of Zhangzhou Affiliated Hospital of Fujian Medical University (No. 2022LWB252). Written informed consent was obtained from all patients.
Table 1
Variable | Values |
---|---|
Age (years) | 65 (48, 77.5) |
Gender | |
Male | 73 (82.0) |
Female | 16 (18.0) |
Admission diagnosis | |
Postabdominal surgery | 19 (21.3) |
Ileus | 9 (10.1) |
Acute pancreatitis | 19 (21.3) |
Intra-abdominal infection | 8 (9.0) |
Intraabdominal bleeding | 6 (6.7) |
Intraperitoneal or retroperitoneal tumors | 13 (14.6) |
Miscellaneous | 15 (16.9) |
IAP (mmHg) | 12 (9, 16) |
Normal (<12 mmHg) | 44 (49.4) |
Grade I (12–15 mmHg) | 22 (24.7) |
Grade II (16–20 mmHg) | 18 (20.2) |
Grade III (21–25 mmHg) | 4 (4.5) |
Grade IV (>25 mmHg) | 1 (1.1) |
Data are presented as median (Q25, Q75) or frequency (%). IAP, intra-abdominal pressure.
The general inclusion and exclusion processes are shown in Figure 1. Patients who met the following criteria were included: (I) any risk factor mentioned in the updated consensus definitions and clinical practice guidelines published by WSACS in 2017, (II) availability of IAP measured via the bladder and other clinical data, and (III) ultrasound examination for the kidney and diaphragm on the day of admission to the ICU. Patients were excluded if they met any of the following criteria: (I) more than 10 years of chronic hypertension (16,18), (II) with renal vascular anomalies, (III) age younger than 18 years, and (IV) treatment with mechanical ventilation.
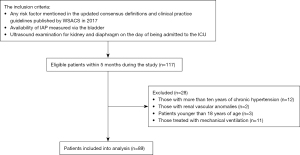
Instruments and methods
IAP was measured using a disposable pressure transducer (DBPT-0103; Zhejiang Haisheng Medical Device Co., Ltd., Shaoxing, China) (Figure 2). We fixed the 3-way pipe at the front end of the pressure sensor to the outflow end of the indwelling needle placed in the urinary catheter and connected the other end to the electrocardiogram monitoring module. IAP was measured via the bladder with a maximal instillation volume of 25 mL of sterile saline at end-expiration with the patient in the supine position after we had ensured that abdominal muscle contractions were absent and with the transducer zeroed at the level of the midaxillary line. Lactic acid (Lac), Sequential Organ Failure Assessment (SOFA) score [PaO2/FiO2, bilirubin, mean arterial pressure (MAP), Glasgow Coma Scale (GCS), creatinine], breathing, heart rate, age, gender, and admission diagnosis were obtained from the medical record system.
The patient was placed in supine position, and all ultrasonic examinations were performed with the same ultrasonic technician and the Mindray Nuewa I9 system (Mindray BioMedical, Shenzhen, China) coupled with linear probe (frequency: 1.2–5 MHz) and a convex probe (frequency: 3–14 MHz) on the day the patient was admitted to the ICU.
The convex probe was used to observe the internal structure of the kidney and the renal blood vessels to measure the RRRI of the interlobar artery (Figure 3A). The point shear wave was then used to measure the average value of the right kidney elasticity (RKE) (Figure 3B). The linear probe was used to observe the diaphragm in the right intercostal space to obtain a 2-dimensional coronal image of the diaphragm. We measured the thickness of the diaphragm at the end of expiration (DTee) (Figure 4A) and at the end of inspiration (Dtei) in spontaneously breathing patients (Figure 4B). The right diaphragm thickening rate (RDTR) was calculated as follows: RDTR = (Dtei − Dtee)/Dtee. We further engaged the 2-dimensional shear wave mode and adjusted the diameter of the sampling box to 0.1–0.2 cm, and then the average value of the right diaphragm elasticity (RDE) was recorded (Figure 4C).
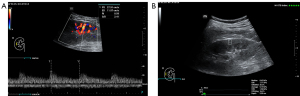
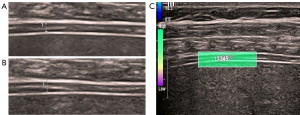
Statistical analysis
All statistical analyses were performed using R version 4.0.5 (http://www.r-project.org/; The R Foundation for Statistical Computing, Vienna, Austria). The mean ± standard deviation is used to present normally distributed data. The median (Q25, Q75) value is used to present skewed distributed data. Categorical data are expressed as frequency and percentage. Comparisons between the 2 groups of patients with and without IAH were made for continuous variables using the Student t-test, the Mann-Whitney test was used to compare differences in nonparametric data, and the Fisher exact test or chi-squared was used for categorical data according to the characteristics of data.
Ultrasound features and clinical data that were significant (P<0.05) in the univariate analysis were included in the multivariate logistic regression model to obtain the odds ratio (OR), 95% confidence interval (CI), and the beta coefficient (β). The correlation between the variables and IAP was analyzed with Spearman correlation analysis. A nomogram was established based on the multivariate logistic regression model. The nomogram was subjected to 1,000 bootstrap resamples for internal validation to assess its predictive accuracy, and a calibration curve was drawn simultaneously. The diagnostic efficacy of the prediction model was evaluated using the area under the curve (AUC) calculated by the receiver operating characteristic (ROC) curve. P<0.05 was considered statistically significant.
Results
Comparison of ultrasound features and clinical data
The RRRI and Lac in the IAH group were higher than those in the non-IAH group, while RDTR was significantly lower. There were no significant differences in SOFA score, age, gender, RKE, RDE, heart rate, or breathing between the non-IAH group and the IAH group (Table 2).
Table 2
Variable | non-IAH group (n=44) | IAH group (n=45) | P |
---|---|---|---|
Age (years) | 71 [56, 79] | 58 [44, 73] | 0.079 |
Gender | |||
Male | 36 (81.82) | 37 (82.22) | 0.960 |
Female | 8 (18.18) | 8 (17.78) | |
SOFA score | 2 [2, 3] | 3 [2, 4] | 0.146 |
PaO2/FiO2 (mmHg) | 308.00 [242.75, 335.25] | 269.00 [244.00, 314.00] | 0.088 |
Platelet (109/L) | 211.00 [140.75, 262.50] | 177.00 [145.50, 272.50] | 0.436 |
Bilirubin (μmol/L) | 16.40 [11.90, 24.53] | 17.70 [13.25, 45.10] | 0.114 |
MAP (mmHg) | 80.50±12.38 | 82.89±12.49 | 0.367 |
GCS | 15 [15, 15] | 15 [15, 15] | 0.337 |
Creatinine (μmol/L) | 81.20 [57.45, 126.88] | 73.40 [60.35, 104.90] | 0.611 |
RRRI | 0.63 [0.58, 0.67] | 0.71 [0.70, 0.73] | <0.001 |
RKE (kPa) | 6.99 [5.90, 9.36] | 6.59 [5.65, 9.12] | 0.478 |
RDTR | 0.20 [0.17, 0.23] | 0.12 [0.10, 0.14] | <0.001 |
RDE (kPa) | 20.58 [18.25, 27.34] | 24.10 [20.43, 29.60] | 0.062 |
Lac (mmol/L) | 1.10 [0.73, 1.50] | 1.70 [0.95, 1.50] | <0.001 |
Heart rate (bpm) | 95.18±17.85 | 91.76±18.39 | 0.375 |
Breathing (bpm) | 18.00 [16.00, 20.75] | 19.00 [16.00, 22.50] | 0.230 |
Data are presented as median [Q25, Q75], mean ± standard deviation or frequency (%). IAH, intra-abdominal hypertension; SOFA score, Sequential Organ Failure Assessment score; MAP, mean arterial pressure; GCS, Glasgow Coma Scale; RRRI, right renal resistance index; RKE, right kidney elasticity; RDTR, right diaphragm thickening rate; RDE, right diaphragm elasticity; Lac, lactic acid.
Model construction and verification
The multivariate logistic regression model included ultrasound features and clinical data with a P<0.05 in the univariate analysis (RRRI, RDTR, and Lac). RRRI, RDTR. and Lac were independent risk factors for IAH, and their correlation with IAP was significant (Table 3).
Table 3
Variable | Multivariate logistic regression | Correlation analysis | ||||
---|---|---|---|---|---|---|
β | OR (95% CI) | P | r | P | ||
RRRI | 31.125 | 7.217 (2.197, 19.727) | 0.001 | 0.741 | <0.001 | |
RDTR | −29.075 | 0.142 (0.047, 0.434) | 0.001 | −0.774 | <0.001 | |
Lac | 0.621 | 3.451 (1.067, 11.206) | 0.039 | 0.396 | <0.001 | |
Constant | −17.274 | – | 0.006 | – | – |
Constant: a part of the logistic regression results since this ensures that the model is unbiased. β, beta coefficient; OR, odds ratio; CI, confidence interval; RRRI, right renal resistance index; RDTR, right diaphragm thickening rate; Lac, lactic acid.
An IAH predictive model was then constructed as follows: Y = −17.274 + 31.125 × RRRI − 29.075 × RDTR + 0.621 × Lac, where Y is the predictive score of IAH. The nomogram (Figure 5) based on this model was established to predict IAH with an AUC of 0.956 (95% CI: 0.909–1.000) (Figure 6A). A calibration curve of the nomogram corrected by bootstrapping (B =1,000 repetitions) (Figure 6B) indicated that the IAH probability predicted by the nomogram agreed with the actual probability (Hosmer-Lemeshow test; P=0.864).
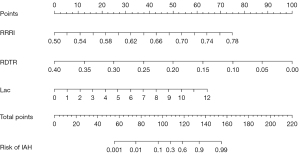
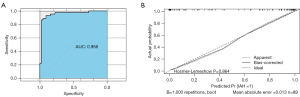
Clinical effectiveness of the nomogram
Decision curve analysis (DCA) showed that the nomogram had good clinical utility when thresholds ranged between 0.20 and 0.90 (Figure 7A). The clinical impact curve showed that the nomogram was feasible for making useful judgments within a wide threshold probability range, especially higher than 0.40 (Figure 7B).
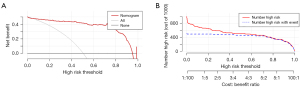
Discussion
IAH, directly and indirectly, adversely affects various organs, with the kidney being particularly susceptible (7,19). At an IAP of 20 mmHg, renal vascular resistance increases by 555%, which is 15 times greater than systemic vascular resistance (20). As IAP increases, renal function can be compromised, with a decrease in renal artery blood flow, resulting in oliguria at an IAP of 15 mmHg and anuria at an IAP over 30 mmHg (21,22). Thus, the kidney may be a potential marker of the adverse physiological effects of IAH. Our study used ultrasound to evaluate renal arteries in critically ill patients with IAH/ACS risk factors. The results showed that RRRI was significantly higher in the IAH group and was an independent risk factor for IAH (P=0.001). These findings are consistent with previous studies (16) although our study had a broader base, as it included all critically ill patients with IAH/ACS risk factors, increasing our results’ scope of application and promotional value.
RDTR was an independent risk factor for IAH (P=0.001). Increases in IAP may lead to a decrease in thoracic compliance, affecting respiratory muscle function. The diaphragm is the primary inspiratory muscle during breathing, and its movement is restricted with decreased compliance (23-25). Ultrasound has emerged as a widely used method for monitoring diaphragm function in patients with chronic obstructive pulmonary disease (26-28). Our study is the first to evaluate diaphragmatic muscle function using ultrasound as a proxy for changes in IAP.
We found Lac to be an independent risk factor for IAH (P=0.039). Lac is an end product of the anaerobic metabolism of glucose and glycogen. When patients experience hypoxia due to tissue hypoperfusion, aerobic oxidation capacity is impaired, and the glycolytic pathway is activated, leading to the formation of Lac through anaerobic metabolism, resulting in increased circulating Lac. Lac is used clinically to evaluate tissue perfusion status (29,30). Patients with severe IAH often experience microcirculatory disturbances, leading to hypoperfusion and hypoxia and in turn, the increased production and accumulation of Lac. Reduced hepatic perfusion retards Lac metabolism, further increasing blood levels, as verified in a porcine model of pneumoperitoneum-induced IAH (31).
Finally, to our knowledge, our study is the first to generate a nomogram to predict the probability of IAH in critically ill patients. The nomogram showed good discrimination (AUC =0.956) and calibration (Hosmer-Lemeshow test, P=0.864). Compared to computed tomography, with an AUC of 0.82 (32), we believe our model has superior diagnostic efficiency and is more economical, convenient, and suitable for critically ill patients. Furthermore, kidney and diaphragm ultrasound examination techniques can be quickly learned by well-trained ICU physicians through short-term training.
The DCA of the nomogram showed a satisfactory net benefit for thresholds between 0.20 and 0.90, which also suggested that it can be used clinically. IAH/ACS is a common complication with high morbidity and mortality rates in critically ill patients (33,34). Therefore, it is essential to monitor the IAP of patients with IAH risk factors (35). This nomogram is a noninvasive alternative for calculating the risk of IAH in critically ill patients. It can be used as a reference for guiding timely clinical interventions, such as adjustment of fluid replacement, body position change, gastrointestinal decompression, paracentesis, and laparotomy to reduce IAH/ACS morbidity and mortality (36-40).
The limitations of our study include the diagnostic angle affecting the detection of color Doppler flow imaging and its inability to reflect the impact of IAH on renal microcirculation perfusion accurately. However, renal contrast-enhanced ultrasound may solve this problem (41,42). Furthermore, our study included a relatively limited number of patients; although the internal validation of the model yielded optimal discrimination and excellent calibration, the generalizability of this nomogram still requires external validation using additional databases. Therefore, our next step in research will involve a large-sample, multicenter, prospective study to obtain more robust conclusions. Despite these limitations, point-of-care ultrasound will be increasingly valuable in guiding clinical interventions to reduce IAH/ACS morbidity and mortality.
Conclusions
This study represents a seminal effort in devising a noninvasive nomogram for predicting the risk of IAH. This newly developed tool provides clinicians with real-time, dynamic insights in a timely manner.
Acknowledgments
We would like to thank Xiaobo Li, Jianhua Chen, and Lipeng Wan from the Ultrasound Imaging System Development Department of Shenzhen Mindray Bio-Medical Electronics Co., Ltd. for their technical support.
Funding: None.
Footnote
Reporting Checklist: The authors have completed the STROBE reporting checklist. Available at https://qims.amegroups.com/article/view/10.21037/qims-23-325/rc
Conflicts of Interest: All authors have completed the ICMJE uniform disclosure form (available at https://qims.amegroups.com/article/view/10.21037/qims-23-325/coif). The authors have no conflicts of interest to declare.
Ethical Statement: The authors are accountable for all aspects of the work in ensuring that questions related to the accuracy or integrity of any part of the work are appropriately investigated and resolved. The study was conducted in accordance with the Declaration of Helsinki (as revised in 2013) and was approved by the ethics committee of Zhangzhou Affiliated Hospital of Fujian Medical University (No. 2022LWB252). Written informed consent was obtained from all patients.
Open Access Statement: This is an Open Access article distributed in accordance with the Creative Commons Attribution-NonCommercial-NoDerivs 4.0 International License (CC BY-NC-ND 4.0), which permits the non-commercial replication and distribution of the article with the strict proviso that no changes or edits are made and the original work is properly cited (including links to both the formal publication through the relevant DOI and the license). See: https://creativecommons.org/licenses/by-nc-nd/4.0/.
References
- Kirkpatrick AW, Sugrue M, McKee JL, Pereira BM, Roberts DJ, De Waele JJ, Leppaniemi A, Ejike JC, Reintam Blaser A, D'Amours S, De Keulenaer B, Malbrain MLNG. Update from the Abdominal Compartment Society (WSACS) on intra-abdominal hypertension and abdominal compartment syndrome: past, present, and future beyond Banff 2017. Anaesthesiol Intensive Ther 2017;49:83-7. [Crossref] [PubMed]
- Kirkpatrick AW, Roberts DJ, De Waele J, Jaeschke R, Malbrain ML, De Keulenaer B, et al. Intra-abdominal hypertension and the abdominal compartment syndrome: updated consensus definitions and clinical practice guidelines from the World Society of the Abdominal Compartment Syndrome. Intensive Care Med 2013;39:1190-206. [Crossref] [PubMed]
- Daugherty EL, Liang Hongyan, Taichman D, Hansen-Flaschen J, Fuchs BD. Abdominal compartment syndrome is common in medical intensive care unit patients receiving large-volume resuscitation. J Intensive Care Med 2007;22:294-9. [Crossref] [PubMed]
- Reintam Blaser A, Regli A, De Keulenaer B, Kimball EJ, Starkopf L, Davis WA, Greiffenstein P, Starkopf J. Incidence, Risk Factors, and Outcomes of Intra-Abdominal Hypertension in Critically Ill Patients-A Prospective Multicenter Study (IROI Study). Crit Care Med 2019;47:535-42.
- De Waele JJ, Cheatham ML, Malbrain ML, Kirkpatrick AW, Sugrue M, Balogh Z, Ivatury R, De Keulenaer B, Kimball EJ. Recommendations for research from the International Conference of Experts on Intra-abdominal Hypertension and Abdominal Compartment Syndrome. Acta Clin Belg 2009;64:203-9. [Crossref] [PubMed]
- Malbrain ML. Different techniques to measure intra-abdominal pressure (IAP): time for a critical re-appraisal. Intensive Care Med 2004;30:357-71. [Crossref] [PubMed]
- Rajasurya V, Surani S. Abdominal compartment syndrome: Often overlooked conditions in medical intensive care units. World J Gastroenterol 2020;26:266-78. [Crossref] [PubMed]
- Newman RK, Dayal N, Dominique E. Abdominal Compartment Syndrome. Treasure Island, FL, USA: StatPearls Publishing; 2023.
- Tagliabue G, Ji M, Suneby Jagers JV, Zuege DJ, Kieser TM, Easton PA. Expiratory and inspiratory action of transversus abdominis during eupnea and hypercapnic ventilation. Respir Physiol Neurobiol 2022;306:103951. [Crossref] [PubMed]
- do Nascimento IB, Fleig R. Mobility impact and methods of diaphragm monitoring in patients with chronic obstructive pulmonary disease: a systematic review. Clinics (Sao Paulo) 2020;75:e1428. [Crossref] [PubMed]
- Xu JH, Wu ZZ, Tao FY, Zhu ST, Chen SP, Cai C, Liang ZH, Shi BB, Chen B, Xie YP. Ultrasound Shear Wave Elastography for Evaluation of Diaphragm Stiffness in Patients with Stable COPD: A Pilot Trial. J Ultrasound Med 2021;40:2655-63. [Crossref] [PubMed]
- Şendur HN, Cerit MN, Şendur AB, Özhan Oktar S, Yücel C. Evaluation of Diaphragm Thickness and Stiffness Using Ultrasound and Shear-Wave Elastography. Ultrasound Q 2022;38:89-93. [Crossref] [PubMed]
- Cao H, Ke B, Lin F, Xue Y, Fang X. Shear Wave Elastography for Assessment of Biopsy-Proven Renal Fibrosis: A Systematic Review and Meta-analysis. Ultrasound Med Biol 2023;49:1037-48. [Crossref] [PubMed]
- Fu Y, He C, Jia L, Ge C, Long L, Bai Y, Zhang N, Du Q, Shen L, Zhao H. Performance of the renal resistive index and usual clinical indicators in predicting persistent AKI. Ren Fail 2022;44:2028-38. [Crossref] [PubMed]
- Romano G, Mioni R, Danieli N, Bertoni M, Croatto E, Merla L, Alcaro L, Pedduzza A, Metcalf X, Rigamonti A, Catena C, Sechi LA, Colussi G. Elevated Intrarenal Resistive Index Predicted Faster Renal Function Decline and Long-Term Mortality in Non-Proteinuric Chronic Kidney Disease. J Clin Med 2022;11:2995. [Crossref] [PubMed]
- Candan Y, Akinci M, Eraslan O, Yilmaz KB, Karabacak H, Dural HI, Tatar IG, Kaya IO. The Correlation of Intraabdominal Pressure With Renal Resistive Index. J Surg Res 2020;252:240-6. [Crossref] [PubMed]
- See KC, Tayebi S, Sum CL, Phua J, Stiens J, Wise R, Mukhopadhyay A, Malbrain MLNG. Feasibility analysis of a novel non-invasive ultrasonographic method for the measurement of intra-abdominal pressure in the intensive care unit. J Clin Monit Comput 2023; Epub ahead of print. [Crossref]
- Gigante A, Perrotta AM, De Marco O, Rosato E, Lai S, Cianci R. Sonographic evaluation of hypertension: Role of atrophic index and renal resistive index. J Clin Hypertens (Greenwich) 2022;24:955-7. [Crossref] [PubMed]
- Pereira BM. Abdominal compartment syndrome and intra-abdominal hypertension. Curr Opin Crit Care 2019;25:688-96.
- Harman PK, Kron IL, McLachlan HD, Freedlender AE, Nolan SP. Elevated intra-abdominal pressure and renal function. Ann Surg 1982;196:594-7. [Crossref] [PubMed]
- Barnes GE, Laine GA, Giam PY, Smith EE, Granger HJ. Cardiovascular responses to elevation of intra-abdominal hydrostatic pressure. Am J Physiol 1985;248:R208-13. [Crossref] [PubMed]
- Mohmand H, Goldfarb S. Renal dysfunction associated with intra-abdominal hypertension and the abdominal compartment syndrome. J Am Soc Nephrol 2011;22:615-21. [Crossref] [PubMed]
- Regli A, De Keulenaer BL, Palermo A, van Heerden PV. Positive end-expiratory pressure adjusted for intra-abdominal pressure - A pilot study. J Crit Care 2018;43:390-4. [Crossref] [PubMed]
- Kirkpatrick AW, Pelosi P, De Waele JJ, Malbrain ML, Ball CG, Meade MO, Stelfox HT, Laupland KB. Clinical review: Intra-abdominal hypertension: does it influence the physiology of prone ventilation? Crit Care 2010;14:232. [Crossref] [PubMed]
- Pelosi P, Quintel M, Malbrain ML. Effect of intra-abdominal pressure on respiratory mechanics. Acta Clin Belg 2007;62:78-88.
- Crimi C, Heffler E, Augelletti T, Campisi R, Noto A, Vancheri C, Crimi N. Utility of ultrasound assessment of diaphragmatic function before and after pulmonary rehabilitation in COPD patients. Int J Chron Obstruct Pulmon Dis 2018;13:3131-9. [Crossref] [PubMed]
- Evrin T, Korkut S, Ozturk Sonmez L, Szarpak L, Katipoglu B, Smereka J, Guven R, Akpinar EE. Evaluating Stable Chronic Obstructive Pulmonary Disease by Ultrasound. Emerg Med Int 2019;2019:5361620. [Crossref] [PubMed]
- McCool FD, Tzelepis GE. Tissue Doppler Imaging of the Diaphragm: A New Kid on the Block? Am J Respir Crit Care Med 2020;202:921-2. [Crossref] [PubMed]
- Nguyen HB, Rivers EP, Knoblich BP, Jacobsen G, Muzzin A, Ressler JA, Tomlanovich MC. Early lactate clearance is associated with improved outcome in severe sepsis and septic shock. Crit Care Med 2004;32:1637-42. [Crossref] [PubMed]
- Kim YA, Ha EJ, Jhang WK, Park SJ. Early blood lactate area as a prognostic marker in pediatric septic shock. Intensive Care Med 2013;39:1818-23. [Crossref] [PubMed]
- Párraga Ros E, Correa-Martín L, Sánchez-Margallo FM, Candanosa-Aranda IE, Malbrain MLNG, Wise R, Latorre R, López Albors O, Castellanos G. Intestinal histopathological changes in a porcine model of pneumoperitoneum-induced intra-abdominal hypertension. Surg Endosc 2018;32:3989-4002. [Crossref] [PubMed]
- Bouveresse S, Piton G, Badet N, Besch G, Pili-Floury S, Delabrousse E. Abdominal compartment syndrome and intra-abdominal hypertension in critically ill patients: diagnostic value of computed tomography. Eur Radiol 2019;29:3839-46. [Crossref] [PubMed]
- Sosa G, Gandham N, Landeras V, Calimag AP, Lerma E. Abdominal compartment syndrome. Dis Mon 2019;65:5-19. [Crossref] [PubMed]
- Cothren CC, Moore EE, Johnson JL, Moore JB. Outcomes in surgical versus medical patients with the secondary abdominal compartment syndrome. Am J Surg 2007;194:804-7; discussion 807-8. [Crossref] [PubMed]
- Murphy PB, Parry NG, Sela N, Leslie K, Vogt K, Ball I. Intra-Abdominal Hypertension Is More Common Than Previously Thought: A Prospective Study in a Mixed Medical-Surgical ICU. Crit Care Med 2018;46:958-64. [Crossref] [PubMed]
- He L, Yi C, Hou Z, Hak DJ. Intraabdominal hypertension/abdominal compartment syndrome after pelvic fractures: How they occur and what can be done? Injury 2019;50:919-25. [Crossref] [PubMed]
- Yi M, Leng Y, Bai Y, Yao G, Zhu X. The evaluation of the effect of body positioning on intra-abdominal pressure measurement and the effect of intra-abdominal pressure at different body positioning on organ function and prognosis in critically ill patients. J Crit Care 2012;27:222.e1-6. [Crossref] [PubMed]
- Lu Z, Zhu X, Hua T, Zhang J, Xiao W, Jia D, Yang M. Efficacy and safety of abdominal paracentesis drainage on patients with acute pancreatitis: a systematic review and meta-analysis. BMJ Open 2021;11:e045031. [Crossref] [PubMed]
- Wen Y, Zhuo WQ, Liang HY, Huang Z, Cheng L, Tian FZ, Wang T, Tang LJ, Luo ZL. Abdominal paracentesis drainage improves outcome of acute pancreatitis complicated with intra-abdominal hypertension in early phase. Am J Med Sci 2023;365:48-55. [Crossref] [PubMed]
- De Laet IE, Ravyts M, Vidts W, Valk J, De Waele JJ, Malbrain ML. Current insights in intra-abdominal hypertension and abdominal compartment syndrome: open the abdomen and keep it open! Langenbecks Arch Surg 2008;393:833-47. [Crossref] [PubMed]
- Cao W, Cui S, Yang L, Wu C, Liu J, Yang F, Liu Y, Bin J, Hou FF. Contrast-Enhanced Ultrasound for Assessing Renal Perfusion Impairment and Predicting Acute Kidney Injury to Chronic Kidney Disease Progression. Antioxid Redox Signal 2017;27:1397-411. [Crossref] [PubMed]
- Yoon HE, Kim DW, Kim D, Kim Y, Shin SJ, Shin YR. A pilot trial to evaluate the clinical usefulness of contrast-enhanced ultrasound in predicting renal outcomes in patients with acute kidney injury. PLoS One 2020;15:e0235130. [Crossref] [PubMed]