Evaluation of left ventricular diastolic function in patients with coronary microvascular dysfunction via cardiovascular magnetic resonance feature tracking
Introduction
Symptoms of myocardial ischemia with no obstructive coronary artery disease (INOCA) are encountered frequently in clinical practice and thus constitute a diagnostic and therapeutic challenge (1-3). Up to two-thirds of patients with INOCA are believed to have coronary microvascular dysfunction (CMD) as the functional substrate of their ischemic symptoms (4-7). CMD is defined as the impaired vasodilation of arterioles leading to a diminished coronary flow reserve (CFR) or an insufficient increase in blood flow from rest to stress (1,5).
CMD is associated with increased mortality, even without macrovascular coronary artery disease (CAD) (8,9). Moreover, accumulating evidence suggests the presence of a correlation between CMD and left ventricular (LV) diastolic dysfunction, with CMD increasing the risk of developing heart failure with preserved ejection fraction (HFpEF) outcomes (10-12). A hypothesis is proposed that the diastolic dysfunction in CMD patients can eventually progress to HFpEF. It is worth noting that patients with CMD and diastolic dysfunction have been associated a poor prognosis, an increased rate of major adverse cardiovascular events (MACEs), and a more than 5-fold rate of HFpEF hospitalization (4,13-16).
Previous studies have reported changes in the echocardiographic indices of diastolic dysfunction in patients with CMD (17,18). Cardiac magnetic resonance (CMR) cine images are more accurate and comprehensive than is echocardiography in assessing LV morphology, LV function, and tissue characterization via CMR feature tracking (CMR-FT) (19-22). However, published research in this area is limited. Our study aimed to investigate whether patients with CMD had impaired LV diastolic function as assessed with CMR-FT.
Methods
Study population
The study participants comprised consecutive patients without a prior history of CAD who had undergone stress perfusion CMR (stress CMR) at Beijing Friendship Hospital between December 2019 and August 2021. They were referred to the cardiology department for suspected myocardial ischemia due to symptoms such as chest pain. Subsequently, they underwent diagnostic coronary computed tomography angiography (CCTA) which demonstrated no evidence of significant coronary stenosis (main coronary stenosis >50% within 1 month). A final cohort of 115 patients with preserved LV ejection fraction (LVEF) was established. Patients with known CAD, including prior revascularization and/or myocardial infarction, primary cardiomyopathy (hypertrophic, dilated, and restrictive), or a prior history of heart failure, atrial fibrillation, or severe valvular disease were excluded from the final cohort. We collected baseline demographics and clinical variables of the study population, which are summarized in Table 1. This study complied with the Declaration of Helsinki (as revised in 2013) and was approved by the Beijing Friendship Hospital Medical Ethics Committee (No. 2021-P2-418-01). All patients provided written informed consent. Figure 1 depicts the study flowchart and exclusion criteria of patients.
Table 1
Variables | Non-CMD, N=19 | CMD, N=73 | P value |
---|---|---|---|
Demographic | |||
Age (years) | 48.8±11.2 | 52.3±11.9 | 0.265 |
Sex (male) | 12 (63.2) | 40 (54.8) | 0.512 |
BMI (kg/m2) | 24.7±3.9 | 25.6±3.6 | 0.338 |
HR rest (beats/min) | 65±11 | 69±9 | 0.052 |
HR stress (beats/min) | 91±18 | 99±15 | 0.036* |
HR increase (beats/min) | 26±13 | 30±12 | 0.264 |
Systolic blood pressure (mmHg) | 123±9 | 128±17 | 0.272 |
Diastolic blood pressure (mmHg) | 73±12 | 77±10 | 0.211 |
CVD risk factor | |||
Hypertension | 7 (36.8) | 32(43.8) | 0.583 |
Diabetes | 4 (21.1) | 17 (23.3) | 0.836 |
Hypercholesterinemia | 9 (47.4) | 32 (43.8) | 0.783 |
Smoker | 6 (31.6) | 20 (27.4) | 0.718 |
Drinking | 6 (31.6) | 18 (24.7) | 0.634 |
Laboratory and echocardiographic parameter | |||
NT-proBNP (pg/mL) | 26.7±21.9 | 55.3±65.1 | 0.261 |
eGFR (mL/min/1.73 m2) | 101.8±9.1 | 95.6±15.8 | 0.198 |
cTnI (ng/mL) | 104.7 (96.6, 117.9) | 98.9 (89.3, 104.8) | 0.837 |
E velocity (cm/s) | 80.3±15.2 | 76.1±18.4 | 0.462 |
A velocity (cm/s) | 78.6±16.8 | 78.0±20.6 | 0.923 |
E/A ratio | 1.1±0.2 | 1.0±0.4 | 0.947 |
Data with a skewed distribution are expressed as median (maximum, minimum) values; otherwise, they are expressed as number (percentage) or mean ± SD. *, significant result. CMD, coronary microvascular dysfunction; BMI, body mass index; HR, heart rate; CVD, cardiovascular disease; NT-proBNP, N-terminal pro-brain natriuretic peptide; eGFR, estimated glomerular filtration rate; cTnI, cardiac troponin I; SD, standard deviation.
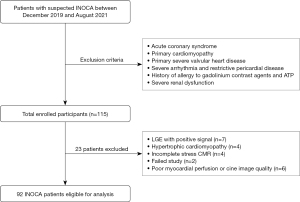
Acquisition of CMR parameters
CMR was performed using a MAGNETOM Prisma 3T magnetic resonance imaging scanner (Siemens Healthineers, Erlangen, Germany). All images were acquired using phased-array surface coils during mild expiration and electrocardiographic triggering. First, patients received adenosine triphosphate (ATP; 5 mg/mL) at an infusion rate of 0.16 mg/kg/min for approximately 4 minutes (heart rate increase ≥10 bpm or systolic blood pressure decrease ≥10 mmHg). This was followed by an intravenous administration of gadolinium (0.5 mmol/mL) at a rate of 3–4 mL/s and a subsequent 30-mL saline flush at the same flow rate to obtain the first-pass perfusion images using a T1-weighted fast gradient echo sequence for both scanners [repetition time/time to echo (TR/TE) =2.1/1.03 ms, TI =100 ms, temporal resolution =155.7 ms, flip angle =10°, voxel size =1.9×1.9×8.0 mm3]. Three perfusion short-axis slice images (base, mid, and apex) of the left ventricle were acquired. Subsequently, balanced steady-state free-precession cine images were acquired in multiple short-axis locations (every centimeter from base to apex) and 3 long-axis locations (2-, 3-, and 4-chamber views; TR/TE =3.3/1.43 ms, temporal resolution =40 ms, flip angle =50°, voxel size =1.6×1.6×6.0 mm3). Rest perfusion images were acquired in the same 3 short-axis positions as those for the stress perfusion images at least 10 minutes after termination of the ATP infusion. Late gadolinium enhancement (LGE) images were acquired at least 10 minutes after gadolinium injection. The LGE parameters were as follows: TR/TE =5.2/1.96 ms, TI =300 ms, flip angle =20°, voxel size =1.8×1.8×8.0 mm3.
LV diastolic function assessment
During image analysis, LV basic cardiac function parameters were measured. This was conducted by automatically tracing the epicardial and endocardial borders of the short-axis cine images at end diastole and end systole automatically using postprocessing software (CVI42, Circle Cardiovascular Imaging, Calgary, Canada). The automatically traced measurements were then manually adjusted as necessary. These parameters included LVEF, LV end diastolic volume (LVEDV), LV end systolic volume (LVESV), cardiac output (CO), stroke volume (SV), and cardiac index (CI).
LV diastolic function was evaluated using the time-volume curve and CMR-FT strain analysis, which enabled the quantification of LV global and regional diastolic functions (19,23,24). The former was generated by continuous the automatic tracing of endocardial borders during complete cardiac cycles with manual adjustment; the first derivative curve used to obtain the LV peak filling rate (PFR) is illustrated in Figure 2. The PFR was defined as the maximal change in the LV volume per unit time (Δ volume/Δ phase), which was the LV maximum slope of the diastolic phase. Diastolic dysfunction was characterized as a decreased peak filling rate and prolonged time-to-peak-filling rate (T-PFR) (25,26). Finally, the myocardial deformation was evaluated with CMR-FT of CMR cine images, as depicted in Figure 2. The indices reflecting LV diastolic function including LV peak longitudinal diastolic strain rate (LDSR), circumferential diastolic strain rate (CDSR), and radial diastolic strain rate (RDSR), were recorded (19,23).
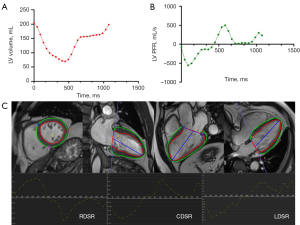
Visual assessment of CMD
The noninvasive quantification of coronary microvascular function was performed using stress CMR. Poor-quality images or incomplete data were excluded from the analysis. The presence of ischemia was evaluated according to the segmental analysis of stress perfusion images, which were recorded in 16 segments (the apical segment is typically not in the field of view) based on the American Heart Association 17-segment model (27). CMD revealed the presence of inducible myocardial ischemia in the subendocardium. Inducible ischemia was defined as the presence of a stress perfusion defect and the absence of matching LGE in ≥1 segment that persisted for ≥5 dynamics (15,28,29), as illustrated in Figure 3. The presence of dark rim artifacts (DRAs) should be excluded during the visual assessment. The characteristics of DRA in this study were considered to be the following: (I) artifacts are the most prominent when contrast arrives in the LV blood pool; (II) artifacts persist only transiently before the peak myocardial contrast enhancement; and (III) artifacts appear predominantly in the phase-encoding direction (30,31). All CMR perfusion images were analyzed by two skilled CMR readers (Cao J and He Y), with one of the readers (He Y) repeating the analyses at an interval of 1 month between analyses. Additionally, semiquantitative myocardial perfusion was analyzed using CVI42 software. This involved manually tracing contours surrounding the endocardium and epicardium to determine the LV myocardial signal intensity. The CMR-derived myocardial perfusion reserve index (MPRI), defined as the ratio of stress to rest upslope normalized to the upslope of the LV blood pool, was recorded (32,33).
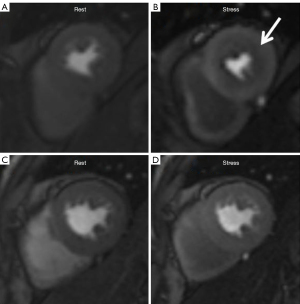
Statistical analysis
Continuous variables were assessed for normality and are presented as the mean ± standard deviation (SD). The normally and nonnormally distributed variables between groups were compared using the independent samples t-test or nonparametric test (Mann-Whitney test or Kolmogorov-Smirnov test). The categorical variables are presented as percentages and were compared using the chi-squared test. The intraclass correlation coefficient (ICC) was used to determine inter- and intraobserver reproducibility. Reproducibility was defined as poor (ICC <0.4), good (ICC 0.4–0.75), or excellent (ICC >0.75), and 95% confidence interval (95% CI) were determined in order to visualize inter- and intraobserver variability. A P value <0.05 indicated a statistically significant difference. All statistical analyses were performed with SPSS 25.0 (IBM Corp., Armonk, NY, USA).
Results
Study demographics
A total of 80% (92/115) patients (52.5±11.8 years; 57% male) were included in the data analysis. Of the 115 patients, 23 patients were excluded for the following reasons: 7 patients had positive LGE, 4 patients had hypertrophic cardiomyopathy, 6 patients failed to complete the study, and 6 patients had poor image quality.
Of these, 73 (79%) patients (52.3±11.9 years; 54.8% male) had inducible myocardial ischemia in the subendocardium. The remaining 19 (21%) patients (48.8±11.2 years; 63.2% male) without myocardial ischemia were classified as non-CMD. Women (33/40, 82.5%) had a greater burden of CMD than did men (40/52, 77%).
At the per-segment level, 160 (11%) segments were excluded from the initial 1,472 myocardial segments due to various factors. These factors included the involvement of the outflow tract involved, scanning plane beyond the mitral valve, scanning plane of stress and rest perfusion mismatch, and inadequate image quality. Hence, the study finally included 1,312 (89%) myocardial segments. Among these segments, 665 (51%) segments were classified as CMD and 647 (49%) as non-CMD. The characteristics of the study population are presented in Table 1.
For the reproducibility of CMD diagnosis by visual assessment, our study showed that the ICC for inter- and intraobserver agreement were 0.906 (95% CI: 0.861–0.937) and 0.874 (95% CI: 0.815–0.915), respectively.
LV diastolic function evaluated using CMR
The LV basic cardiac function parameters revealed a lower MPRI and SV in the CMD group compared with the non-CMD group (MPRI: 1.49±0.3 vs. 1.86±0.6, P<0.005; SV: 71.21±15.4 vs. 76.96±9.3 mL, P=0.045; Table 2). All patients had a preserved LVEF of ≥50%. No difference between the CMD and non-CMD groups was noted in terms of LVEF (60.42±7.4 vs. 62.61±8.3%; P=0.266) and LVEDV (118.09±22.2 vs. 124.59±20.8 mL; P=0.253). The PFR and T-PFR values were also not significantly different between the 2 groups (both P values >0.05; Table 2).
Table 2
Variables | Non-CMD, N=19 | CMD, N=73 | P value |
---|---|---|---|
LV basic function | |||
MPRI | 1.86±0.6 | 1.49±0.3 | <0.005* |
LVEF (%) | 62.61±8.3 | 60.42±7.4 | 0.266 |
LVEDV (mL) | 124.59±20.8 | 118.09±22.2 | 0.253 |
LVEDV/BSA | 65.69±11.9 | 61.0±10.1 | 0.292 |
LVESV (mL) | 47.63±17.3 | 46.88±13.7 | 0.842 |
LVESV/BSA | 25.85±8.6 | 25.83±7.2 | 0.990 |
CO (L/min) | 5.5±1.4 | 6.1±7.0 | 0.740 |
SV (mL) | 76.96±9.3 | 71.21±15.4 | 0.045* |
CI (L/min/m2) | 3.11±0.9 | 3.15±0.8 | 0.832 |
LV global diastolic function | |||
PFR (mL/s) | 372.03±107.1 | 382.41±127.6 | 0.757 |
T-PFR (s) | 484.88±126.8 | 457.77±54.9 | 0.374 |
Global LDSR (s−1) | 1.06±0.4 | 1.19±0.4 | 0.192 |
Global CDSR (s−1) | 0.91±0.3 | 0.98±0.4 | 0.423 |
Global RDSR (s−1) | −1.69±0.9 | −1.67±1.3 | 0.946 |
Data are expressed as mean ± SD. *, significant result. LV, left ventricular; CMD, coronary microvascular dysfunction; MPRI, myocardial perfusion reserve index; LVEF, LV ejection fraction; LVEDV, LV end diastolic volume; BSA, body surface area; LVESV, LV end systolic volume; CO, cardiac output; SV, stroke volume; CI, cardiac index; PFR, peak filling rate; T-PFR, time-to-peak-filling rate; LDSR, longitudinal diastolic strain rate; CDSR, circumferential diastolic strain rate; RDSR, radial diastolic strain rate; SD, standard deviation.
In addition, the LV global diastolic strain rates were not considerably distinct between the 2 groups (Table 2). However, when LV was divided into 16 segments for analysis and at the per-segment level, the ischemic segments revealed a remarkably lower regional CDSR {median 1.26 [interquartile range (IQR), 1.01–1.57] vs. median 1.32 (IQR, 1.00–1.65), P=0.019}, and regional RDSR [median −2.28 (IQR, −3.06 to −1.65) vs. median −2.45 (IQR, −3.41 to −1.64), P=0.006] compared with the non-CMD segments. Furthermore, the regional LDSR was substantially higher in the CMD segments compared with the non-CMD segments [median 1.76 (IQR, 1.21–2.48) vs. median 1.55 (IQR, 1.18–2.26), P=0.003] (Table 3).
Table 3
Variables | Non-CMD | CMD | P value |
---|---|---|---|
Segments | 647 | 665 | |
Regional LDSR (s−1) | 1.55 (1.18–2.26) | 1.76 (1.21–2.48) | 0.003* |
Regional CDSR (s−1) | 1.32 (1.00–1.65) | 1.26 (1.01–1.57) | 0.019* |
Regional RDSR (s−1) | −2.45 (−3.41 to −1.64) | −2.28 (−3.06 to −1.65) | 0.006* |
Data with a skewed distribution are expressed as median (IQR) values. *, significant result. LV, left ventricular; CMR-FT, cardiovascular magnetic resonance feature tracking; CMD, coronary microvascular dysfunction; LDSR, longitudinal diastolic strain rate; CDSR, circumferential diastolic strain rate; RDSR, radial diastolic strain rate; IQR, inter-quartile range.
Discussion
In this study, CMR parameters were used to verify the altered LV diastolic function in patients with CMD. First, the incidence of CMD was found to be higher in patients with signs and symptoms of ischemia but not in those with signs of obstructive CAD. Second, LV global diastolic function did not decrease in patients with CMD. Third, the regional LV diastolic function of CMD patients decreased.
Our findings are consistent with those of previous studies in terms of the clinical characteristics of CMD. Waheed et al. (34) reported that women typically had a greater burden of CMD, which was also reflected in our study. Of the female patients in this group, 82.5% (33/40) had CMD, whereas only 77% (40/52) of men had CMD. Moreover, all recruited patients with INOCA in both the CMD and non-CMD groups exhibited cardiovascular (CV) risk factors (hypertension, diabetes, hypercholesterolemia, smoking, and drinking). Differences in LV diastolic parameters between the 2 groups could still be observed, suggesting that the impaired LV diastolic function in patients with CMD cannot be attributable to the CV risk factors. As for the incidence of CMD, several studies have reported CMD in a large proportion (one-half to two-thirds) of patients with INOCA (35,36). Interestingly, in Wei et al.’s study, CMD was present in 83% of women (25). The aforementioned CV risk factors eventually led to the development of CMD, indicating that the prevalence of CMD in this study population was relatively high.
Regarding regional CMD, previous studies (37,38) have confirmed that microcirculation abnormalities have a patchy distribution in the myocardial wall rather than a diffuse distribution, which is consistent with our visual evaluation. According to The European Society of Cardiology (ESC) Working Group on Coronary Pathophysiology and Microcirculation position paper (39), the mechanisms underlying CMD in patients nonobstructive CAD are still not completely understood, but they likely involve both functional and structural changes. Additionally, endothelial dysfunction is a key mediator in the pathogenesis of CMD, and it is believed that with advancement in medical technology, the future study of CMD will be more thorough and clearer.
Evaluation of LV global diastolic function using LV filling curves
Notably, no PFR or T-PFR differences were observed between the patients with and without CMD in this study. In the study by Mendoza et al., reduced PFR and prolonged T-PFR were found to reflect one aspect of LV global diastolic dysfunction, and LV filling curves could be used to discern the volumetric filling changes that occurred with graded severity of diastolic dysfunction. Moreover, the PFR decreased during the progression from normal diastolic function to grade 1 diastolic dysfunction and then increased during the progression from grade 1 diastolic dysfunction to grade 3 diastolic dysfunction (40). Therefore, even if PFR does not decrease, this does not necessarily mean that LV diastolic function is normal. In our study, the PFR in the CMD group did not decrease, which is in line with the findings of Wei et al.’s study (25). Moreover, the same results were obtained even in patients with HFpEF (26). In our study, the LV global diastolic function evaluated using LV filling curves did not decrease in patients with CMD, indicating that these parameters were not sufficiently sensitive to detect early LV diastolic dysfunction.
Evaluation of LV diastolic function using CMR-FT diastolic strain rates
The CMR strain technique to assess LV diastolic function was proposed several years ago (41) and has been used in a variety of diseases (17,19,24-26). The Women’s Ischemia Syndrome Evaluation (WISE) study (42) demonstrated that diastolic function is impaired in women with signs and symptoms of ischemia without CAD, as assessed by CMR tissue tagging. Our study further stratified patients with INOCA into a CMD group and a non-CMD group, with decreased LV diastolic function being found to be more pronounced in CMD myocardial segments. Although our study demonstrated no reduction in CMR parameters indicating LV global diastolic function, this could be explained by the lack of ischemia in the myocardial segment during the early compensatory stage in patients with CMD.
In contrast to the studies by Taqueti et al. (16) and Shah et al. (10), in which stress myocardial perfusion positron emission tomography (PET) and adenosine stress transthoracic Doppler echocardiography were examined, respectively, our study used the CMR-FT technique to characterize cardiac morphology and diastolic function. The findings from the studies that patients with CMD have more impaired LV diastolic dysfunction than do non-CMD individuals were in concordance with our results. The aforementioned studies reported that the patients with impaired CFR or CMD a worse diastolic function, including both a lower e¢ and higher E/e¢, as compared with the patients with preserved CFR or an absence of CMD. The LV ejection fractions in both groups were preserved, and there were no statistically significant differences in this regard. However, the pathophysiological mechanism underlying this phenomenon is still unclear (43).
CMD leading to increased LV diastolic stiffening has recently been proposed as the mechanism underlying the pathogenesis of HFpEF (44-47) due to the fact that the prevalence of CMD is high in both patients with HFpEF and patients with INOCA (10,12,16). We found that the prevalence of CMD assessed using stress CMR was 79% in the population of patients with INOCA. Thus, coronary microvascular ischemia caused by CMD may lead to myocardial injury, worsen LV global mechanics, and result in LV dysfunction. In particular, microvascular endothelial dysfunction and increased profibrotic cytokine may contribute to reduced coronary microvascular density and increased myocardial fibrosis, eventually progressing to HFpEF (16,44,48). Meanwhile, a recent PET study (16) reported a link between CMD and diastolic dysfunction in patients with angina and no obstructive CAD. Our study also demonstrated that CMD segments had a slightly impaired LV diastolic function compared with non-CMD segments. These findings thus suggest that one of the pathophysiological mechanisms underlying HFpEF is LV diastolic dysfunction and stiffness due to coronary microvascular ischemia.
Our study also a few limitations that should be noted. First, a single-center study design was employed with relatively small cohorts. Thus, future multicenter studies with larger cohorts are needed to confirm our findings. Second, we did not include a normal healthy population as a control group. Third, strain indicators, such as global longitudinal strain (GLS), which can serve as the marker of subclinical LV dysfunction, were not included in this article (49). This issue was addressed in our other studies in which CMD myocardial segments exhibited remarkably lower longitudinal strain (LS) and circumferential strain (CS). Moreover, biomarkers such as brain natriuretic peptide and cardiac troponin can serve as markers of LV dysfunction. However, heart failure biomarkers were not examined in this study, which limits scope of clinical applicability. However, we will collect this patient information in later studies. Finally, in this study we did not employ CFR, the gold standard for CMD. However, the technology is invasive and complex to operate, so our study did not examine this technology. Moreover, as confirmed by several studies (50-54), the sensitivity and specificity of stress CMR for the diagnosis of CMD are comparable to those of the invasive or noninvasive gold standard, but with CMR involving less pain, radiation dose, and cost to patients.
Conclusions
Our study found CMD myocardial segments to have decreased LV diastolic function but normal LVEF. These findings point to the early diastolic impairment in CMD and establish a link between CMD and HFpEF. However, LV global diastolic function did not decrease in patients with CMD in the early compensatory phase. Further studies are warranted to confirm whether there is an association between CMD and LV diastolic function in those patients with INOCA.
Acknowledgments
Funding: This study received funding from
Footnote
Conflicts of Interest: All authors have completed the ICMJE uniform disclosure form (available at https://qims.amegroups.com/article/view/10.21037/qims-23-47/coif). The authors have no conflicts of interest to declare.
Ethical Statement: The authors are accountable for all aspects of the work in ensuring that questions related to the accuracy or integrity of any part of the work are appropriately investigated and resolved. The study was conducted in accordance with the Declaration of Helsinki (as revised in 2013) and was approved by the Beijing Friendship Hospital Medical Ethics Committee (No. 2021-P2-418-01). Written informed consent was obtained from all patients in this study.
Open Access Statement: This is an Open Access article distributed in accordance with the Creative Commons Attribution-NonCommercial-NoDerivs 4.0 International License (CC BY-NC-ND 4.0), which permits the non-commercial replication and distribution of the article with the strict proviso that no changes or edits are made and the original work is properly cited (including links to both the formal publication through the relevant DOI and the license). See: https://creativecommons.org/licenses/by-nc-nd/4.0/.
References
- Marinescu MA, Löffler AI, Ouellette M, Smith L, Kramer CM, Bourque JM. Coronary microvascular dysfunction, microvascular angina, and treatment strategies. JACC Cardiovasc Imaging 2015;8:210-20. [Crossref] [PubMed]
- Bugiardini R, Bairey Merz CN. Angina with "normal" coronary arteries: a changing philosophy. JAMA 2005;293:477-84. [Crossref] [PubMed]
- Patel MR, Peterson ED, Dai D, Brennan JM, Redberg RF, Anderson HV, Brindis RG, Douglas PS. Low diagnostic yield of elective coronary angiography. N Engl J Med 2010;362:886-95. [Crossref] [PubMed]
- Murthy VL, Naya M, Foster CR, Hainer J, Gaber M, Di Carli G, Blankstein R, Dorbala S, Sitek A, Pencina MJ, Di Carli MF. Improved cardiac risk assessment with noninvasive measures of coronary flow reserve. Circulation 2011;124:2215-24. [Crossref] [PubMed]
- Rahman H, Ryan M, Lumley M, Modi B, McConkey H, Ellis H, Scannell C, Clapp B, Marber M, Webb A, Chiribiri A, Perera D. Coronary Microvascular Dysfunction Is Associated With Myocardial Ischemia and Abnormal Coronary Perfusion During Exercise. Circulation 2019;140:1805-16. [Crossref] [PubMed]
- Schindler TH, Dilsizian V. Coronary Microvascular Dysfunction: Clinical Considerations and Noninvasive Diagnosis. JACC Cardiovasc Imaging 2020;13:140-55. [Crossref] [PubMed]
- Tonet E, Pompei G, Faragasso E, Cossu A, Pavasini R, Passarini G, Tebaldi M, Campo G. Coronary Microvascular Dysfunction: PET, CMR and CT Assessment. J Clin Med 2021;10:1848. [Crossref] [PubMed]
- Brainin P, Frestad D, Prescott E. The prognostic value of coronary endothelial and microvascular dysfunction in subjects with normal or non-obstructive coronary artery disease: A systematic review and meta-analysis. Int J Cardiol 2018;254:1-9. [Crossref] [PubMed]
- Suhrs HE, Schroder J, Bové KB, Mygind ND, Frestad D, Michelsen MM, Lange T, Gustafsson I, Kastrup J, Prescott E. Inflammation, non-endothelial dependent coronary microvascular function and diastolic function-Are they linked? PLoS One 2020;15:e0236035. [Crossref] [PubMed]
- Shah SJ, Lam CSP, Svedlund S, Saraste A, Hage C, Tan RS, Beussink-Nelson L, Ljung Faxén U, Fermer ML, Broberg MA, Gan LM, Lund LH. Prevalence and correlates of coronary microvascular dysfunction in heart failure with preserved ejection fraction: PROMIS-HFpEF. Eur Heart J 2018;39:3439-50. [Crossref] [PubMed]
- D'Amario D, Migliaro S, Borovac JA, Restivo A, Vergallo R, Galli M, Leone AM, Montone RA, Niccoli G, Aspromonte N, Crea F. Microvascular Dysfunction in Heart Failure With Preserved Ejection Fraction. Front Physiol 2019;10:1347. [Crossref] [PubMed]
- Rush CJ, Berry C, Oldroyd KG, Rocchiccioli JP, Lindsay MM, Touyz RM, Murphy CL, Ford TJ, Sidik N, McEntegart MB, Lang NN, Jhund PS, Campbell RT, McMurray JJV, Petrie MC. Prevalence of Coronary Artery Disease and Coronary Microvascular Dysfunction in Patients With Heart Failure With Preserved Ejection Fraction. JAMA Cardiol 2021;6:1130-43. [Crossref] [PubMed]
- Sammut EC, Villa ADM, Di Giovine G, Dancy L, Bosio F, Gibbs T, Jeyabraba S, Schwenke S, Williams SE, Marber M, Alfakih K, Ismail TF, Razavi R, Chiribiri A. Prognostic Value of Quantitative Stress Perfusion Cardiac Magnetic Resonance. JACC Cardiovasc Imaging 2018;11:686-94. [Crossref] [PubMed]
- Heitner JF, Kim RJ, Kim HW, Klem I, Shah DJ, Debs D, Farzaneh-Far A, Polsani V, Kim J, Weinsaft J, Shenoy C, Hughes A, Cargile P, Ho J, Bonow RO, Jenista E, Parker M, Judd RM. Prognostic Value of Vasodilator Stress Cardiac Magnetic Resonance Imaging: A Multicenter Study With 48 000 Patient-Years of Follow-up. JAMA Cardiol 2019;4:256-64. [Crossref] [PubMed]
- Zhou W, Lee JCY, Leung ST, Lai A, Lee TF, Chiang JB, Cheng YW, Chan HL, Yiu KH, Goh VK, Pennell DJ, Ng MY. Long-Term Prognosis of Patients With Coronary Microvascular Disease Using Stress Perfusion Cardiac Magnetic Resonance. JACC Cardiovasc Imaging 2021;14:602-11. [Crossref] [PubMed]
- Taqueti VR, Solomon SD, Shah AM, Desai AS, Groarke JD, Osborne MT, Hainer J, Bibbo CF, Dorbala S, Blankstein R, Di Carli MF. Coronary microvascular dysfunction and future risk of heart failure with preserved ejection fraction. Eur Heart J 2018;39:840-9. [Crossref] [PubMed]
- Nelson MD. Left ventricular diastolic dysfunction in women with nonobstructive ischemic heart disease: insights from magnetic resonance imaging and spectroscopy. Am J Physiol Regul Integr Comp Physiol 2017;313:R322-9.
- Lam JH, Quah JX, Davies T, Boos CJ, Nel K, Anstey CM, Stanton T, Greaves K. Relationship between coronary microvascular dysfunction and left ventricular diastolic function in patients with chest pain and unobstructed coronary arteries. Echocardiography 2020;37:1199-204. [Crossref] [PubMed]
- Chamsi-Pasha MA, Zhan Y, Debs D, Shah DJ. CMR in the Evaluation of Diastolic Dysfunction and Phenotyping of HFpEF: Current Role and Future Perspectives. JACC Cardiovasc Imaging 2020;13:283-96. [Crossref] [PubMed]
- Nagel E, Chandrashekhar Y. Stress-Only CMR. JACC Cardiovasc Imaging 2020;13:1296-8. [Crossref] [PubMed]
- Qu YY, Paul J, Li H, Ma GS, Buckert D, Rasche V. Left ventricular myocardial strain quantification with two- and three-dimensional cardiovascular magnetic resonance based tissue tracking. Quant Imaging Med Surg 2021;11:1421-36. [Crossref] [PubMed]
- Wang F, Xu X, Wang Q, Yu D, Lv L, Wang Q. Comparison of left ventricular global and segmental strain parameters by cardiovascular magnetic resonance tissue tracking in light-chain cardiac amyloidosis and hypertrophic cardiomyopathy. Quant Imaging Med Surg 2023;13:449-61. [Crossref] [PubMed]
- Nagueh SF, Shah DJ. Echocardiography First, But Here Comes CMR for Grading Left Ventricular Diastolic Function. JACC Cardiovasc Imaging 2020;13:2543-5. [Crossref] [PubMed]
- Bojer AS, Soerensen MH, Gaede P, Myerson S, Madsen PL. Left Ventricular Diastolic Function Studied with Magnetic Resonance Imaging: A Systematic Review of Techniques and Relation to Established Measures of Diastolic Function. Diagnostics (Basel) 2021;11:1282. [Crossref] [PubMed]
- Wei J, Mehta PK, Shufelt C, Yang Y, Gill E, Kahlon R, Cook-Wiens G, Minissian M, Kar S, Thomson L, Berman D, Merz CN. Diastolic dysfunction measured by cardiac magnetic resonance imaging in women with signs and symptoms of ischemia but no obstructive coronary artery disease. Int J Cardiol 2016;220:775-80. [Crossref] [PubMed]
- Gao C, Tao Y, Pan J, Shen C, Zhang J, Xia Z, Wan Q, Wu H, Gao Y, Shen H, Lu Z, Wei M. Evaluation of elevated left ventricular end diastolic pressure in patients with preserved ejection fraction using cardiac magnetic resonance. Eur Radiol 2019;29:2360-8. [Crossref] [PubMed]
- Cerqueira MD, Weissman NJ, Dilsizian V, Jacobs AK, Kaul S, Laskey WK, Pennell DJ, Rumberger JA, Ryan T, Verani MSAmerican Heart Association Writing Group on Myocardial Segmentation and Registration for Cardiac Imaging. Standardized myocardial segmentation and nomenclature for tomographic imaging of the heart. A statement for healthcare professionals from the Cardiac Imaging Committee of the Council on Clinical Cardiology of the American Heart Association. Circulation 2002;105:539-42. [Crossref] [PubMed]
- Pezel T, Sanguineti F, Kinnel M, Hovasse T, Garot P, Unterseeh T, Champagne S, Louvard Y, Morice MC, Garot J. Prognostic value of dipyridamole stress perfusion cardiovascular magnetic resonance in elderly patients >75 years with suspected coronary artery disease. Eur Heart J Cardiovasc Imaging 2021;22:904-11. [Crossref] [PubMed]
- Rahman H, Scannell CM, Demir OM, Ryan M, McConkey H, Ellis H, Masci PG, Perera D, Chiribiri A. High-Resolution Cardiac Magnetic Resonance Imaging Techniques for the Identification of Coronary Microvascular Dysfunction. JACC Cardiovasc Imaging 2021;14:978-86. [Crossref] [PubMed]
- Ferreira P, Gatehouse P, Kellman P, Bucciarelli-Ducci C, Firmin D. Variability of myocardial perfusion dark rim Gibbs artifacts due to sub-pixel shifts. J Cardiovasc Magn Reson 2009;11:17. [Crossref] [PubMed]
- Schulz-Menger J, Bluemke DA, Bremerich J, Flamm SD, Fogel MA, Friedrich MG, Kim RJ, von Knobelsdorff-Brenkenhoff F, Kramer CM, Pennell DJ, Plein S, Nagel E. Standardized image interpretation and post-processing in cardiovascular magnetic resonance - 2020 update: Society for Cardiovascular Magnetic Resonance (SCMR): Board of Trustees Task Force on Standardized Post-Processing. J Cardiovasc Magn Reson 2020;22:19. [Crossref] [PubMed]
- Shehata ML, Basha TA, Hayeri MR, Hartung D, Teytelboym OM, Vogel-Claussen J. MR myocardial perfusion imaging: insights on techniques, analysis, interpretation, and findings. Radiographics 2014;34:1636-57. [Crossref] [PubMed]
- Mygind ND, Pena A, Mide Michelsen M, Ali Qayyum A, Frestad D, Emil Christensen T, Ali Ghotbi A, Hasbak P, Kjaer A, Vejlstrup N, Gustafsson I, Riis Hansen P, Steen Hansen H, Prescott E, Kastrup J. Myocardial first pass perfusion assessed by cardiac magnetic resonance and coronary microvascular dysfunction in women with angina and no obstructive coronary artery disease. Scand J Clin Lab Invest 2019;79:238-46. [Crossref] [PubMed]
- Waheed N, Elias-Smale S, Malas W, Maas AH, Sedlak TL, Tremmel J, Mehta PK. Sex differences in non-obstructive coronary artery disease. Cardiovasc Res 2020;116:829-40. [Crossref] [PubMed]
- Ong P, Athanasiadis A, Borgulya G, Mahrholdt H, Kaski JC, Sechtem U. High prevalence of a pathological response to acetylcholine testing in patients with stable angina pectoris and unobstructed coronary arteries. The ACOVA Study (Abnormal COronary VAsomotion in patients with stable angina and unobstructed coronary arteries). J Am Coll Cardiol 2012;59:655-62. [Crossref] [PubMed]
- Reis SE, Holubkov R, Conrad Smith AJ, Kelsey SF, Sharaf BL, Reichek N, Rogers WJ, Merz CN, Sopko G, Pepine CJ. WISE Investigators. Coronary microvascular dysfunction is highly prevalent in women with chest pain in the absence of coronary artery disease: results from the NHLBI WISE study. Am Heart J 2001;141:735-41. [Crossref] [PubMed]
- Panting JR, Gatehouse PD, Yang GZ, Grothues F, Firmin DN, Collins P, Pennell DJ. Abnormal subendocardial perfusion in cardiac syndrome X detected by cardiovascular magnetic resonance imaging. N Engl J Med 2002;346:1948-53. [Crossref] [PubMed]
- Thomson LE, Wei J, Agarwal M, Haft-Baradaran A, Shufelt C, Mehta PK, Gill EB, Johnson BD, Kenkre T, Handberg EM, Li D, Sharif B, Berman DS, Petersen JW, Pepine CJ, Bairey Merz CN. Cardiac magnetic resonance myocardial perfusion reserve index is reduced in women with coronary microvascular dysfunction. A National Heart, Lung, and Blood Institute-sponsored study from the Women's Ischemia Syndrome Evaluation. Circ Cardiovasc Imaging 2015; [Crossref]
- Padro T, Manfrini O, Bugiardini R, Canty J, Cenko E, De Luca G, Duncker DJ, Eringa EC, Koller A, Tousoulis D, Trifunovic D, Vavlukis M, de Wit C, Badimon L. ESC Working Group on Coronary Pathophysiology and Microcirculation position paper on 'coronary microvascular dysfunction in cardiovascular disease'. Cardiovasc Res 2020;116:741-55. [Crossref] [PubMed]
- Mendoza DD, Codella NC, Wang Y, Prince MR, Sethi S, Manoushagian SJ, Kawaji K, Min JK, LaBounty TM, Devereux RB, Weinsaft JW. Impact of diastolic dysfunction severity on global left ventricular volumetric filling - assessment by automated segmentation of routine cine cardiovascular magnetic resonance. J Cardiovasc Magn Reson 2010;12:46. [Crossref] [PubMed]
- Caudron J, Fares J, Bauer F, Dacher JN. Evaluation of left ventricular diastolic function with cardiac MR imaging. Radiographics 2011;31:239-59. [Crossref] [PubMed]
- Nelson MD, Szczepaniak LS, Wei J, Haftabaradaren A, Bharadwaj M, Sharif B, Mehta P, Zhang X, Thomson LE, Berman DS, Li D, Bairey Merz CN. Diastolic dysfunction in women with signs and symptoms of ischemia in the absence of obstructive coronary artery disease: a hypothesis-generating study. Circ Cardiovasc Imaging 2014;7:510-6. [Crossref] [PubMed]
- Nelson MD, Wei J, Bairey Merz CN. Coronary microvascular dysfunction and heart failure with preserved ejection fraction as female-pattern cardiovascular disease: the chicken or the egg? Eur Heart J 2018;39:850-2. [Crossref] [PubMed]
- Paulus WJ, Tschöpe C. A novel paradigm for heart failure with preserved ejection fraction: comorbidities drive myocardial dysfunction and remodeling through coronary microvascular endothelial inflammation. J Am Coll Cardiol 2013;62:263-71. [Crossref] [PubMed]
- Franssen C, Chen S, Unger A, Korkmaz HI, De Keulenaer GW, Tschöpe C, Leite-Moreira AF, Musters R, Niessen HW, Linke WA, Paulus WJ, Hamdani N. Myocardial Microvascular Inflammatory Endothelial Activation in Heart Failure With Preserved Ejection Fraction. JACC Heart Fail 2016;4:312-24. [Crossref] [PubMed]
- Lam CS, Lund LH. Microvascular endothelial dysfunction in heart failure with preserved ejection fraction. Heart 2016;102:257-9. [Crossref] [PubMed]
- Crea F, Bairey Merz CN, Beltrame JF, Kaski JC, Ogawa H, Ong P, Sechtem U, Shimokawa H, Camici PGCoronary Vasomotion Disorders International Study Group (COVADIS). The parallel tales of microvascular angina and heart failure with preserved ejection fraction: a paradigm shift. Eur Heart J 2017;38:473-7. [Crossref] [PubMed]
- Mohammed SF, Hussain S, Mirzoyev SA, Edwards WD, Maleszewski JJ, Redfield MM. Coronary microvascular rarefaction and myocardial fibrosis in heart failure with preserved ejection fraction. Circulation 2015;131:550-9. [Crossref] [PubMed]
- Ishikawa H, Otsuka K, Kono Y, Hojo K, Yamaura H, Hirata K, Kasayuki N, Izumiya Y, Fukuda D. Extent of coronary atherosclerosis is associated with deterioration of left ventricular global longitudinal strain in patients with preserved ejection fraction undergoing coronary computed tomography angiography. Int J Cardiol Heart Vasc 2023;44:101176. [Crossref] [PubMed]
- Schwitter J, Nanz D, Kneifel S, Bertschinger K, Büchi M, Knüsel PR, Marincek B, Lüscher TF, von Schulthess GK. Assessment of myocardial perfusion in coronary artery disease by magnetic resonance: a comparison with positron emission tomography and coronary angiography. Circulation 2001;103:2230-5. [Crossref] [PubMed]
- Schwitter J, Wacker CM, van Rossum AC, Lombardi M, Al-Saadi N, Ahlstrom H, Dill T, Larsson HB, Flamm SD, Marquardt M, Johansson L. MR-IMPACT: comparison of perfusion-cardiac magnetic resonance with single-photon emission computed tomography for the detection of coronary artery disease in a multicentre, multivendor, randomized trial. Eur Heart J 2008;29:480-9. [Crossref] [PubMed]
- Schwitter J, Wacker CM, Wilke N, Al-Saadi N, Sauer E, Huettle K, Schönberg SO, Debl K, Strohm O, Ahlstrom H, Dill T, Hoebel N, Simor TMR-IMPACT investigators. Superior diagnostic performance of perfusion-cardiovascular magnetic resonance versus SPECT to detect coronary artery disease: The secondary endpoints of the multicenter multivendor MR-IMPACT II (Magnetic Resonance Imaging for Myocardial Perfusion Assessment in Coronary Artery Disease Trial). J Cardiovasc Magn Reson 2012;14:61. [Crossref] [PubMed]
- Schwitter J, Wacker CM, Wilke N, Al-Saadi N, Sauer E, Huettle K, Schönberg SO, Luchner A, Strohm O, Ahlstrom H, Dill T, Hoebel N, Simor T. MR-IMPACT Investigators. MR-IMPACT II: Magnetic Resonance Imaging for Myocardial Perfusion Assessment in Coronary artery disease Trial: perfusion-cardiac magnetic resonance vs. single-photon emission computed tomography for the detection of coronary artery disease: a comparative multicentre, multivendor trial. Eur Heart J 2013;34:775-81. [Crossref] [PubMed]
- Foley JRJ, Kidambi A, Biglands JD, Maredia N, Dickinson CJ, Plein S, Greenwood JP. A comparison of cardiovascular magnetic resonance and single photon emission computed tomography (SPECT) perfusion imaging in left main stem or equivalent coronary artery disease: a CE-MARC substudy. J Cardiovasc Magn Reson 2017;19:84. [Crossref] [PubMed]