The effect of cartilage dehydration and rehydration on quantitative ultrashort echo time biomarkers
Introduction
Ex vivo hyaline articular cartilage samples are commonly used in magnetic resonance imaging (MRI) research to study osteoarthritis and related joint diseases. These samples facilitate destructive and invasive testing that may otherwise be prohibitive. It is widely accepted that the optimal condition of cartilage samples is immediately after harvest from a living host with extreme care to avoid dehydration. In reality, this is often not possible and even after a few minutes of dehydration in room air, measurable changes in cartilage thickness are present (1). For accurate correlation of results obtained from such in vivo scenarios, a thorough understanding of how tissue handling and preparation affect quantitative measures of interest is mandatory (2-5). To our knowledge, changes in cartilage subject to dehydration and rehydration have not been systematically studied. Researchers may be unaware of the amount of dehydration that has occurred in their samples and thusly unaware of the effect this may have on their quantitative magnetic resonance (MR) results as well.
In recent years, a range of quantitative ultrashort echo time (UTE) MRI techniques have been used to assess cartilage properties (6,7), including T1 (8), adiabatic T1ρ (AdiabT1ρ) (9-11), macromolecular fraction (MMF) (12), magnetization transfer ratio (MTR) (13), and T2* (14). The current study aimed to evaluate the effect of cartilage dehydration and rehydration on these quantitative UTE biomarkers and to investigate what type of solution is able to adequately rehydrate the cartilage without significantly confounding biomarker measurements, using results from fresh cartilage samples as the reference standard. We have the hypothesis that cartilage dehydration will affect the results of UTE biomarkers and that the changes may be reversed by rehydration with synovial fluid or normal saline.
Methods
Sample preparation
The study was conducted in accordance with the Declaration of Helsinki (as revised in 2013) and approved by the Institutional Review Board of VA San Diego Healthcare System (No. H170124). Written informed consent was obtained from all patients. Thirty-seven osteochondral cores were harvested from patients (n=5, mean age was 71±3.2 years old) who underwent total knee arthroplasty (TKA). The osseous parts were removed by a scalpel. The entire process from surgical resection to cartilage procurement was performed within 30 minutes using an air humidifier to mitigate sample dehydration. Fresh cartilage cores were weighed before baseline MR scan. Then the cartilage samples were put in syringes with perfluoropolyether immediately after they were weighed and notched to minimize dehydration. The time between cartilage collection and baseline MR scanning was within 30 minutes. Native synovial fluid was collected during surgery.
MR sequences
All cartilage wafers were piled up in a 10-mL syringe. Perfluoropolyether (Fomblin; Ausimont, Thorofare, NJ, USA) was added in the syringe to minimize air-tissue susceptibility and dehydration during MRI. Each sample was carved a small notch to keep the orientation across scans to minimize the influence of dipole effects on UTE measurements (15-18). A 3T clinical MRI scanner (MR750, GE Healthcare Technologies, Milwaukee, WI, USA) with a self-developed birdcage coil (30 mm) was used for image scanning. The imaging protocols were as follows: (I) 3D UTE-cones with actual flip angle imaging and variable flip angles (3D UTE-Cones AFI-VFA) with four flip angles (FA) of 5°, 10°, 20°, and 30°, and a TR of 20 ms (8); (II) 3D UTE-Cones with AdiabT1ρ preparation (3D UTE-Cones-AdiabT1ρ) with seven spin-locking times (TSL) of 0, 12, 24, 36, 48, 72, and 96 ms (9); (III) 3D UTE-Cones magnetization transfer (3D UTE-Cones-MT) with three saturation pulse powers of 400°, 600°, and 800° and five frequency offsets of 2, 5, 10, 20, and 50 kHz (19); (IV) 3D UTE-T2* with six TEs of 0.032, 4.1, 8.1, 12.1, 16.1, and 32 ms (Figure 1). Other imaging parameters were as follows: number of excitation (NEX) =1, receiver bandwidth (BW) =83.3 KHz, field of view (FOV) =5×5 cm2, acquisition matrix =160×160, slice thickness =0.5 mm, 60 slices, under sampling factor =1. The scan times for single T1, T1ρ, MT and T2* sequences were 2.02, 2.33, 2.05, and 1.25 min, respectively, and the total scan time was 78 min. The representative fitting curves of UTE-based T1, T1ρ, MMF and T2* are shown in Figure 2.
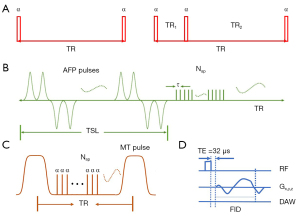
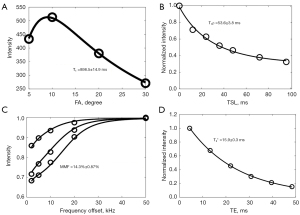
Dehydration and rehydration process
After baseline MRI scanning, samples were exposed to air and allowed to dehydrate for 2 hours. Sample weights were recorded before and after dehydration. Then samples were stacked back into the syringe filled with perfluoropolyether in the same order and orientation as the baseline for repeated MR scanning using the same imaging protocol. After the second-time MR scanning, samples from individual patients were then randomly divided into two rehydration groups, with one soaking in native synovial fluid (n=17) and the other soaking in normal saline (n=20). After 4 hours of rehydration, samples were removed from the solution and their surfaces were wiped with Kimwipes. Kimwipes were used to wipe the surface each time the cartilage samples were taken from the solution before weighing. Weights were recorded before and after rehydration. The third-time MR scanning was performed following the rehydration process using the same method as the baseline and the second-time MR scanning.
Imaging data analysis
The DICOM images obtained by the MR sequences described above were used for imaging data analysis using MATLAB (The MathWorks Inc., Natick, MA, USA). Regions of interests (ROIs) were drawn on the first image of UTE series manually, then copied to the subsequent images. Non-linear least-squares curve fitting was based on the mean intensity within each ROI using the Levenberg-Marquardt method. Single-component fitting method was used for T1 measurement by the 3D UTE-AFI and UTE-VTR sequences. A fitting algorithm based on the equation:
where α accounts for flip angle was used for T1ρ analysis (20). A two-pool model (19) was used for MTR (21) and MMF calculation based on UTE-MT data set. A single-component fitting model, , was utilized for T2* decay analyses where C accounts for background noise (22).
Three consecutive layers in the center of each cartilage sample were manually drawn for global ROI analysis (The mean ROI size was 18.6±1.3 mm2) (Figure 3). UTE-based biomarkers, including T1, AdiabT1ρ, MMF, MTR, and T2*, were measured for all cartilage samples at fresh, dehydration, and rehydration time points. Representative T1, T1ρ, and MMF maps of fresh, dehydrated, and rehydrated cartilage samples are shown in Figure 3.
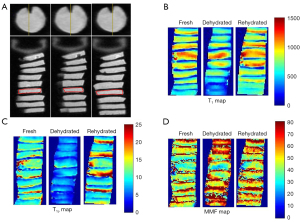
Statistical analysis
Statistical analyses were performed using SPSS software (SPSS 24.0, IBM, New York, NY, USA). The normality of the data distribution was evaluated by the Kolmogorov-Smirnov test. A two-sided paired t-test with Bonferroni correction (to calibrate type I errors, where P values less than 0.0167 were considered statistically significant) was performed to evaluate biomarker differences between fresh, dehydrated, and rehydrated samples. Repeated measures ANOVA analysis was used to determine the effects of dehydration and rehydration by synovial fluid and normal saline on UTE-based biomarkers. A P value less than 0.05 was statistically significant. The agreement of the UTE biomarkers between the rehydrated and fresh samples was accessed by Bland-Altman analysis.
Results
Figure 4 depicts the significant weight loss observed in samples after dehydration in air for 2 hours. With 4 hours of submersion, no differences were detected between the fresh and rehydrated cartilage samples in both synovial fluid and normal saline groups (all P>0.0167). Across samples, significant changes were observed in all evaluated UTE biomarkers after dehydration, with T1, AdiabT1ρ, and T2* values decreasing, and MMF and MTR values increasing. There are no significant differences observed in T1, AdiabT1ρ, MMF, and MTR values after rehydrating in synovial fluid, whereas T2* values still exhibited significant post-rehydration differences compared to fresh samples. On the other hand, all the UTE biomarkers, including T1, AdiabT1ρ, MMF, MTR, and T2* values after rehydrating with normal saline had non-significant differences with the measurements taken when samples were still fresh.
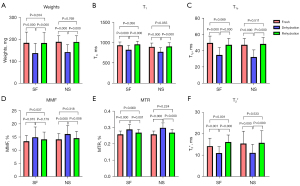
The results of repeated measures ANOVA indicate that all the P values were <0.001 for weights, T1, AdiabT1ρ, MMF, MTR, and T2* when only the factor of time points was taken into consideration, indicating that the differences at each time point were statistically significant for both synovial fluid and normal saline groups. All P values were >0.05 for weights (P=0.811), T1 (P=0.255), AdiabT1ρ (P=0.117), MMF (P=0.543), MTR (P=0.720), and T2* (P=0.091) when both time points and group factors were taken into account, indicating that there is no interaction between time points and group, which means the weights and UTE biomarkers of cartilage samples at different time points (fresh, dehydration, and rehydration) did not vary by groups (synovial fluid and normal saline groups).
The Bland-Altman plots of all biomarkers, including T1, AdiabT1ρ, MMF, MTR, and T2* for cartilage samples in the synovial fluid group and the normal saline group are shown in Figures 5,6, respectively, with measurements taken from the fresh samples considered as baseline. The differences between baseline and the rehydration state are depicted on the vertical axis, with their averages depicted on the horizontal axis. In the synovial fluid group, only 1/17 was outside the 95% limit of agreement (LoA) for T1, AdiabT1ρ, and MTR, while all data points were within the 95% LoA for MMF and T2*. In comparison, the Bland-Altman plots of the normal saline group (Figure 6) showed that all data points were within the 95% LoA for T1 and MMF, while only 1/20 data points was outside the 95% LoA for AdiabT1ρ, MTR, and T2*. These findings suggest that there is high reproducibility of UTE biomarkers after the rehydration process either with synovial fluid or normal saline and that cartilage samples rehydrated in normal saline demonstrate no differences in reproducibility with samples rehydrated using synovial fluid.
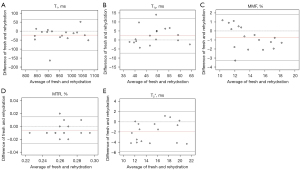
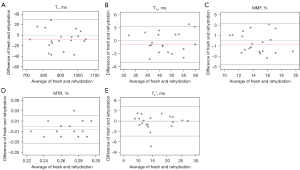
Discussion
The purpose of this study was to evaluate the effects of dehydration and rehydration with synovial fluid and normal saline on quantitative UTE biomarkers in an ex vivo cartilage study. In this study, we found that MR imaging of dehydrated cartilage rehydrated with normal saline did not significantly alter UTE-based T1, AdiabT1ρ, MMF, MTR, and T2* measurements. The findings of our current study are important as they suggest that rehydration of the dehydrated cartilage samples with normal saline can be used effectively in an MR study of imaging characteristics of cartilage ex vivo.
Our results demonstrate that while there was significant sample dehydration after exposure to typical ambient air for 2 hours, both synovial fluid and normal saline brought weights of cartilage back to normal without causing the samples to swell, which is consistent with the result of a previous literature that the mass of 40% dehydrated cartilage appeared to be steady after 5 hours of submersion in normal saline and was not statistically different from that level of rehydration after 16 hours (23). Cartilage dehydration resulted in significant changes in all the evaluated UTE biomarkers, with T1, AdiabT1ρ, and T2* values decreased while MMF and MTR values increased. One possible explanation for this is that cartilage moisture loss during dehydration results in relative increases in proteoglycan (PG) and collagen concentration (2). Previous studies have reported that T1 and AdiabT1ρ increase with PG loss (24-26), so it is reasonable that significant decreases were observed in T1 and AdiabT1ρ after dehydration in our study. MMF and MTR value increases with cartilage dehydration, consistent with previous studies where MMF and MTR were directly proportional to collagen concentration (10,12,27). The unexpected increase in T2* values after rehydrating in synovial fluid was observed. More research is still needed to investigate whether rehydration with synovial fluid affects cartilage microstructure (e.g., via polarized light microscopy), glycosaminoglycans (GAGs) content (e.g., via dimethylmethylene blue assay), and other macromolecules (and thereby, tissue osmolarity).
Our results showed no statistical differences in UTE biomarkers between fresh samples and samples rehydrated with normal saline in comparison to samples rehydrated with synovial fluid. The most likely reason for this is that cartilage dehydration is mainly a loss of water content without changes to the internal microstructure of the tissue [as opposed to storage at a low temperature such as −20 ℃ or −80 ℃ without cryopreservation, which might result in a significant loss of GAGs in cartilage (3)]. Not only does normal saline contain more water than synovial fluid, but the osmolarity of native synovial fluid obtained from TKA patients may also change as a result of disease (28). Additionally, normal saline is relatively easy to obtain, while the collection of native synovial fluid during surgery is more complicated and sometimes the amount of synovial fluid might be small. Therefore, in the case of cartilage dehydration, it is feasible to rehydrate with normal saline instead of synovial fluid in practice.
There are several limitations to our study. First, the sample size of this study is relatively small, especially in each sub-group with 17 cartilage samples in the synovial fluid group and 20 in the normal saline group. Second, the cartilage samples only came from five donors, which inherently limits the variability of the data. Third, histological or immunohistochemical tests were not performed but might have been useful in demonstrating the biochemical changes in cartilage samples during the dehydration-rehydration process. Fourth, only the effects of dehydration and rehydration were evaluated in this current study. Given that freezing samples at −80 ℃ is one of the most common sample processing methods, it is important to investigate the effects of freezing on the quantitative MRI measures of cartilage samples. Fifth, cartilage samples in our study were obtained from patients who underwent TKA, so samples were of varying levels of degeneration, with some relatively healthy and some degenerated tissues. It remains to be investigated whether degeneration of cartilage may affect its overall ability to be rehydrated. Finally, the values of fresh cartilage samples were used as the reference standard for rehydrated and dehydrated samples, but they were also subject to measurement error and bias.
Conclusions
Based on our preliminary results, cartilage dehydration caused significant changes in the quantitative results of UTE biomarkers with a significant decrease in T1, AdiabT1ρ, and T2* values and an increase in MMF and MTR values. Rehydrating with synovial fluid or normal saline had non-significant effect on all the evaluated UTE biomarkers except T2* values, which still had significant differences compared with fresh samples after rehydrating with synovial fluid. No significant difference was observed in the rehydration capabilities in native synovial fluid and normal saline.
Acknowledgments
Funding: This work was supported by
Footnote
Conflicts of Interest: All authors have completed the ICMJE uniform disclosure form (available at https://qims.amegroups.com/article/view/10.21037/qims-23-359/coif). JD serves as an unpaid editorial board member of Quantitative Imaging in Medicine and Surgery. The other authors have no conflicts of interest to declare.
Ethical Statement: The authors are accountable for all aspects of the work in ensuring that questions related to the accuracy or integrity of any part of the work are appropriately investigated and resolved. The study was conducted in accordance with the Declaration of Helsinki (as revised in 2013) and approved by the Institutional Review Board of VA San Diego Healthcare System (No. H170124). Written informed consent was obtained from all patients.
Open Access Statement: This is an Open Access article distributed in accordance with the Creative Commons Attribution-NonCommercial-NoDerivs 4.0 International License (CC BY-NC-ND 4.0), which permits the non-commercial replication and distribution of the article with the strict proviso that no changes or edits are made and the original work is properly cited (including links to both the formal publication through the relevant DOI and the license). See: https://creativecommons.org/licenses/by-nc-nd/4.0/.
References
- Pham A, Hull ML. Dehydration rates of meniscus and articular cartilage in vitro using a fast and accurate laser-based coordinate digitizing system. J Biomech 2007;40:3223-9. [Crossref] [PubMed]
- Fishbein KW, Canuto HC, Bajaj P, Camacho NP, Spencer RG. Optimal methods for the preservation of cartilage samples in MRI and correlative biochemical studies. Magn Reson Med 2007;57:866-73. [Crossref] [PubMed]
- Zheng S, Xia Y, Bidthanapally A, Badar F, Ilsar I, Duvoisin N. Damages to the extracellular matrix in articular cartilage due to cryopreservation by microscopic magnetic resonance imaging and biochemistry. Magn Reson Imaging 2009;27:648-55. [Crossref] [PubMed]
- Chang EY, Bae WC, Statum S, Du J, Chung CB. Effects of repetitive freeze-thawing cycles on T2 and T2 of the Achilles tendon. Eur J Radiol 2014;83:349-53. [Crossref] [PubMed]
- Chang EY, Du J, Bae WC, Statum S, Chung CB. Effects of Achilles tendon immersion in saline and perfluorochemicals on T2 and T2*. J Magn Reson Imaging 2014;40:496-500. [Crossref] [PubMed]
- Shao H, Yang J, Ma Y, Su X, Tang G, Jiang J, Du J, Liu J. Evaluation of cartilage degeneration using multiparametric quantitative ultrashort echo time-based MRI: an ex vivo study. Quant Imaging Med Surg 2022;12:1738-49. [Crossref] [PubMed]
- Su X, Zhang Y, Gao Q, Liang Z, Wan L, Zhang L, Tang G. Preliminary study on the assessment of early cartilage degeneration by quantitative ultrashort echo time magnetic resonance imaging in vivo. Quant Imaging Med Surg 2022;12:3803-12. [Crossref] [PubMed]
- Ma YJ, Zhao W, Wan L, Guo T, Searleman A, Jang H, Chang EY, Du J. Whole knee joint T(1) values measured in vivo at 3T by combined 3D ultrashort echo time cones actual flip angle and variable flip angle methods. Magn Reson Med 2019;81:1634-44. [Crossref] [PubMed]
- Ma YJ, Carl M, Searleman A, Lu X, Chang EY, Du J. 3D adiabatic T(1ρ) prepared ultrashort echo time cones sequence for whole knee imaging. Magn Reson Med 2018;80:1429-39. [Crossref] [PubMed]
- Wan L, Cheng X, Searleman AC, Ma YJ, Wong JH, Meyer RS, Du J, Tang G, Chang EY. Evaluation of enzymatic proteoglycan loss and collagen degradation in human articular cartilage using ultrashort echo time-based biomarkers: A feasibility study. NMR Biomed 2022;35:e4664. [Crossref] [PubMed]
- Wu M, Ma YJ, Liu M, Xue Y, Gong L, Wei Z, Jerban S, Jang H, Chang DG, Chang EY, Ma L, Du J. Quantitative assessment of articular cartilage degeneration using 3D ultrashort echo time cones adiabatic T(1ρ) (3D UTE-Cones-AdiabT(1ρ)) imaging. Eur Radiol 2022;32:6178-86.
- Xue YP, Ma YJ, Wu M, Jerban S, Wei Z, Chang EY, Du J. Quantitative 3D Ultrashort Echo Time Magnetization Transfer Imaging for Evaluation of Knee Cartilage Degeneration In Vivo. J Magn Reson Imaging 2021;54:1294-302. [Crossref] [PubMed]
- Wan L, Ma Y, Yang J, Jerban S, Searleman AC, Carl M, Le N, Chang EY, Tang G, Du J. Fast quantitative three-dimensional ultrashort echo time (UTE) Cones magnetic resonance imaging of major tissues in the knee joint using extended sprial sampling. NMR Biomed 2020;33:e4376. [Crossref] [PubMed]
- Chu CR, Williams AA, West RV, Qian Y, Fu FH, Do BH, Bruno S. Quantitative Magnetic Resonance Imaging UTE-T2* Mapping of Cartilage and Meniscus Healing After Anatomic Anterior Cruciate Ligament Reconstruction. Am J Sports Med 2014;42:1847-56. [Crossref] [PubMed]
- Du J, Pak BC, Znamirowski R, Statum S, Takahashi A, Chung CB, Bydder GM. Magic angle effect in magnetic resonance imaging of the Achilles tendon and enthesis. Magn Reson Imaging 2009;27:557-64. [Crossref] [PubMed]
- Shao H, Pauli C, Li S, Ma Y, Tadros AS, Kavanaugh A, Chang EY, Tang G, Du J. Magic angle effect plays a major role in both T1rho and T2 relaxation in articular cartilage. Osteoarthritis Cartilage 2017;25:2022-30. [Crossref] [PubMed]
- Wu M, Ma Y, Wan L, Jerban S, Jang H, Chang EY, Du J. Magic angle effect on adiabatic T(1ρ) imaging of the Achilles tendon using 3D ultrashort echo time cones trajectory. NMR Biomed 2020;33:e4322. [Crossref] [PubMed]
- Namiranian B, Jerban S, Ma Y, Dorthe EW, Masoud-Afsahi A, Wong J, Wei Z, Chen Y, D'Lima D, Chang EY, Du J. Assessment of mechanical properties of articular cartilage with quantitative three-dimensional ultrashort echo time (UTE) cones magnetic resonance imaging. J Biomech 2020;113:110085. [Crossref] [PubMed]
- Ma YJ, Chang EY, Carl M, Du J. Quantitative magnetization transfer ultrashort echo time imaging using a time-efficient 3D multispoke Cones sequence. Magn Reson Med 2018;79:692-700. [Crossref] [PubMed]
- Ma YJ, Carl M, Shao H, Tadros AS, Chang EY, Du J. Three-dimensional ultrashort echo time cones T(1ρ) (3D UTE-cones-T1ρ) imaging. NMR Biomed 2017; [Crossref]
- Springer F, Martirosian P, Machann J, Schwenzer NF, Claussen CD, Schick F. Magnetization transfer contrast imaging in bovine and human cortical bone applying an ultrashort echo time sequence at 3 Tesla. Magn Reson Med 2009;61:1040-8. [Crossref] [PubMed]
- Chang EY, Du J, Iwasaki K, Biswas R, Statum S, He Q, Bae WC, Chung CB. Single- and Bi-component T2* analysis of tendon before and during tensile loading, using UTE sequences. J Magn Reson Imaging 2015;42:114-20. [Crossref] [PubMed]
- Meyer JP, McAvoy KE, Jiang J. Rehydration capacities and rates for various porcine tissues after dehydration. PLoS One 2013;8:e72573. [Crossref] [PubMed]
- Akella SV, Regatte RR, Gougoutas AJ, Borthakur A, Shapiro EM, Kneeland JB, Leigh JS, Reddy R. Proteoglycan-induced changes in T1rho-relaxation of articular cartilage at 4T. Magn Reson Med 2001;46:419-23. [Crossref] [PubMed]
- Nissi MJ, Salo EN, Tiitu V, Liimatainen T, Michaeli S, Mangia S, Ellermann J, Nieminen MT. Multi-parametric MRI characterization of enzymatically degraded articular cartilage. J Orthop Res 2016;34:1111-20. [Crossref] [PubMed]
- Lin PC, Reiter DA, Spencer RG. Sensitivity and specificity of univariate MRI analysis of experimentally degraded cartilage. Magn Reson Med 2009;62:1311-8. [Crossref] [PubMed]
- Zhang X, Ma YJ, Wei Z, Wu M, Ashir A, Jerban S, Li S, Chang EY, Du J. Macromolecular fraction (MMF) from 3D ultrashort echo time cones magnetization transfer (3D UTE-Cones-MT) imaging predicts meniscal degeneration and knee osteoarthritis. Osteoarthritis Cartilage 2021;29:1173-80.
- Newman PJ, Grana WA. The changes in human synovial fluid osmolality associated with traumatic or mechanical abnormalities of the knee. Arthroscopy 1988;4:179-81. [Crossref] [PubMed]