Simultaneous percutaneous microwave ablation and biopsy for highly suspected malignant pulmonary nodules: a retrospective cohort study
Introduction
The incidence rate of lung cancer in China ranks first among malignant tumors (57.26/100,000), with 631,000 lung cancer deaths every year (1). A 10-year study with a large sample size showed that 85% of stage I lung cancer cases can be found by annual chest low-dose computed tomography (CT) screening (2). The National Cancer Institute (NCI) conducted a large-scale randomized controlled study that included 53,454 high-risk lung cancer patients, and the results showed that CT screening could reduce the mortality of lung cancer by 20% (3). Except in primary lung cancer, lung metastasis is prone to develop from malignant tumors, such as digestive, urogenital, and head/neck tumors, in the process of natural disease development, and the development of lung metastases means that the tumor has entered an advanced stage (4). For patients in whom there is a high suspicion of malignant pulmonary nodules (PNs), microwave ablation (MWA) can be performed directly without pathological results when the medical imaging findings and tumor histories are concordant with each other (5). However, percutaneous biopsy (PB) is necessary when there are doubts about the clinical diagnosis or when there is a need to obtain evidence of metastasis gene mutations for further treatment adjustments.
MWA is a new effective technology in which the MWA applicator is inserted into the tumor, and the heat energy generated by the MWA generator is transmitted to the tumor through the applicator and results in local tumor necrosis through thermal conduction (6). In clinical diagnosis and treatment, PB of nodules is still an important method to obtain the patient’s histopathological results before MWA. Previous research has shown that if the lesion is smaller and the solid component is smaller, the probability of bleeding during PB is higher (7). Therefore, how to reduce bleeding and synchronously complete PB has always been a great concern to clinical interventional experts. In addition, for some patients, PB is performed first, and further treatment is initiated when the pathological results are available (the routine pathological results take 2–5 days at our center), which will prolong the hospital stay, increase the total costs, and prolong patient discomfort (8).
Previous research has shown that MWA can rapidly cauterize small blood vessels and tracheal tissue through heat production to reduce local bleeding, but too high a temperature can lead to local carbonization (9). Clasen et al. (10) performed CT-guided PN radiofrequency ablation (RFA). Then, 3 days after surgical resection, a pathologic examination was performed, which found that the morphologic characteristics postablation were still visible by hematoxylin and eosin (HE) staining, but the local tissue was determined to be in regression toward coagulative necrosis. Therefore, the tumor morphology can be a feasible diagnostic test in the postablation area. However, their pilot study focused on RFA, with less attention paid to MWA. Theoretically, the frequency of RFA and WMA are 460 KHz and 2,450 MHz respectively, which means that the latter has higher heat generation efficiency. We assumed that the biopsy needle and MWA applicator puncture the PN synchronously and then fix the PN with low-power MWA (to avoid severe carbonization) to cauterize the blood vessels and tracheal tissue around the nodule (to reduce bleeding). After successful sampling, the nodule was completely damaged with another high-power MWA. This retrospective cohort study showed our initial experience. We present this article in accordance with the STROBE reporting checklist (available at https://qims.amegroups.com/article/view/10.21037/qims-23-138/rc).
Methods
Patients
The study was conducted in accordance with the Declaration of Helsinki (as revised in 2013). This retrospective study was approved by the institutional review board, and informed consent was provided by all the participants. From August 2015 to August 2022, 65 consecutive patients [48 solid nodules, 6 ground glass opacities (GGOs), 11 mixed nodules] with suspected malignant single PNs (malignant signs such as lobulation sign, hairpin sign, pleural indentation sign, bronchial inflation sign, vascular bundle sign within the PNs, or diameter enlargement during follow-up) underwent MWA and PB combination treatment at our hospital (Heyi District and Huiji District, the First Affiliated Hospital of Zhengzhou University). The 30 participants in Group A underwent synchronous PB and MWA (strategy: low power MWA-biopsy-high power MWA), and the 35 patients in Group B underwent asynchronous PB and MWA. All procedures were performed by the same 2 experienced operators (Jiao D and Du K, who had 16 and 13 years of experience in lung puncture, respectively). The inclusion criteria were as follows: (I) age ≥18 years; (II) highly suspected to have malignant PNs; (III) maximum diameter of single PN ≤30 mm; (IV) Karnofsky score ≥80; (V) platelet count ≥50×109/L and prothrombin time ≤21 s; (VI) distance from the nodule to a major vessel or a main bronchus of the lung hilar ≥2 cm; and (VII) forced vital capacity ≥50%. The exclusion criteria were as follows: (I) number of PNs ≥2; (II) severe cardiopulmonary dysfunction; and (III) severe pleural effusion on the puncture approach. All patients undergoing MWA were determined after multidisciplinary consultation about the tumor. The workflow and detailed information are listed in Figure 1 and Table 1.
Table 1
Parameters | Group A (n=30) | Group B (n=35) | P value |
---|---|---|---|
Age (years) | 56.3±11.6 | 56.7±10.1 | 0.93 |
Sex (male/female) | 18/12 | 22/13 | 0.81 |
Primary malignant tumor history (yes/no) | 13/17 | 14/21 | 0.79 |
Tobacco smoker (active/former/never) | 16/12/2 | 19/14/2 | 0.90 |
Nodule diameter (cm) | 2.3±0.5 | 2.2±0.5 | 0.52 |
Diameter stratification (≤2.0/>2.0 cm) | 7/23 | 11/24 | 0.47 |
Image characteristic (solid/partial solid/GGO) | 23/4/3 | 25/7/3 | |
Nodule lung location (left/right) | 21/9 | 20/15 | 0.28 |
Nodule location (central/peripheral) | 6/24 | 6/29 | 0.77 |
ECOG score (0/1/2) | 23/5/2 | 18/14/3 | 0.10 |
COPD (yes/no) | 14/16 | 13/22 | 0.88 |
Pathologic results | |||
Adenocarcinoma | 10 (33.3) | 16 (45.7) | 0.45 |
Metastasis | 11 (36.7) | 14 (40.0) | 0.80 |
Atypical adenomatous hyperplasia | 2 (6.7) | 4 (11.4) | 0.68 |
SCLC | 1 (3.3) | 1 (2.9) | 0.91 |
Data are presented as mean ± standard deviation or number (frequency)/n (%). Group A underwent synchronous PB and MWA (strategy: low-power MWA-PB-high-power MWA). Group B underwent asynchronous PB and MWA. GGO, ground glass opacities; ECOG, Eastern Cooperative Oncology Group; COPD, chronic obstructive pulmonary disease; SCLC, small cell lung cancer; PB, percutaneous biopsy; MWA, microwave ablation.
The procedures of Group A
The patient’s body position was adjusted according to the PN position to ensure a suitable puncture pathway. Dexmedetomidine (0.5 µg/kg) and dezocine (10 mg) were administered intravenously to achieve a satisfactory sedation state, and general anesthesia could be used if the patients (especially those with very small lesions close to the pleura) could not cooperate with the breathing requirements.
Step 1 (position): the nodule was positioned by chest CT (Brilliance16; Philips, Best, The Netherlands; current: 90 mA; voltage: 120 kV and thickness: 3 mm), and the puncture path was designed according to the operator’s experience. Considering that the biopsy needle and MWA applicator will be inserted into the PN synchronously, it is necessary to set 2 puncture paths with a certain angle between the puncture paths to reduce the risk of pneumothorax. After local disinfection, 2% lidocaine (5–10 mL) was used for local puncture site anesthesia.
Step 2 (puncture): the MWA applicator with a diameter of 1.6 mm (150 mm in length; Eco Co., Ltd., Nanjing, China) was inserted into the PN “step by step” for the guidance of CT.
Step 3 (solidification): when the MWA applicator punctures the nodule, ablation can be performed with low power/short time mode (20–25 W/30–60 s) to fix the nodule.
Step 4 (biopsy): the 17G biopsy needle (Argon, Athens, TX, USA) was inserted into the PN “step by step” according to the treatment plan. Then, the 18G biopsy gun was used to insert the needle core for tissue sampling, which was performed 1–3 times to obtain enough tissue (length 1.3 or 2.3 cm), and specimens were preserved in 10% formalin for pathological examination. Further immunohistochemistry and gene mutation tests (GMTs) were carried out according to the patients’ requirements.
Step 5 (radical ablation): MWA was performed again to obtain radical ablation. The ablation parameter (35–40 W/3–6 min) was selected according to the PN characteristics. It is recommended that the ablation range should cover the nodule margin by more than 5–10 mm on postablation CT (Figures 2-4).
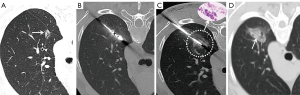
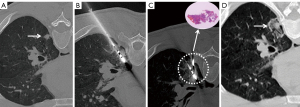
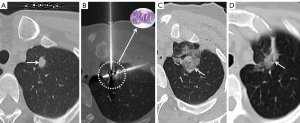
Step 6 (verification): another CT was performed to exclude immediate complications such as pneumothorax and hemoptysis. Chest CT or X-ray examination was performed regularly 6–8 hours later to monitor for the occurrence of pneumothorax, and if the patient had any signs of exacerbation of dyspnea, CT or X-ray examination was performed immediately.
The procedures of Group B
The steps of nodule positioning, puncture, biopsy, and complication treatment processes were the same as Group A. The difference is that PB and PMA were done asynchronously and at a different time, and the ablation parameters (35–40 W/3–6 min) were selected directly to fulfill radical ablation. The abovementioned Step 3 to fix the nodule using the lowpower/short time strategy was not included.
Definition and follow-up
Technical success was defined as successful completion of PB and MWA in both groups. Complete ablation (CA) was defined as imaging changes such as fibrotic progression, solid nodule involution, cavitation, disappearance, and atelectasis, and there were contrast-enhanced signs on CT. Incomplete ablation was defined as remaining enhanced solid residues, partial fibrosis with solid residues, and increased size with irregular peripheral enhancement on subsequent CT (11). Total procedure time (TPT) was defined as the time from Step 1 to Step 6.
The complications included intrapulmonary hemorrhage (a small amount of bleeding: the bleeding was limited to 2 cm from the edge of the lesion; moderate amount of bleeding: the bleeding range exceeded 2 cm from the edge of the lesion or extended into other lung lobes); and hemoptysis (a small amount of hemoptysis: hemoptysis ≤10 mL; moderate amount of hemoptysis: 10 mL < hemoptysis ≤100 mL; large amount of hemoptysis: hemoptysis >100 mL); pneumothorax (a small amount of pneumothorax: pulmonary compression ≤20%; moderate amount of pneumothorax: 20% < pulmonary compression ≤40%; large amount of pneumothorax: pulmonary compression >40%). The following conditions were met for the patients in need of GMT: DNA extraction ≥30 ng/case, average sequencing depth ≥1,000×, and sequence reply ratio/coverage ≥95%. Combined with the above parameters, the overall quality of the sample was comprehensively evaluated and divided into 3 levels: qualified, alert, and unqualified. Follow-up was performed 1, 3, 6, 9, 12, 15, and 18 months after MWA. Since the patients in Group B needed to complete PB and MWA at different time points, the complications were recorded if any pneumothorax or hemorrhage occurred, and the complication analysis was based on a total of 70 patients. Patient exposure dose (PED; mSV) was calculated as follows: PED = κDLP × dose-length product (mGy·cm), κDLP = 0.015 mSv/mGy·cm.
Statistical analysis
The software SPSS 22.0 (IBM Corp., Armonk, NY, USA) was used for statistical analysis. All data were expressed as numbers (mean ± standard deviation), and percentages. All parameters between both groups were compared by an independent samples t-test, χ2 test or Fisher’s exact test. Statistical significance was considered when P<0.05.
Results
From August 2015 to August 2022, a total of 164 patients underwent wet lung PB combined with MWA treatments, of whom 65 patients met the retrospective study criteria, including 48 solid nodules, 6 GGOs, and 11 mixed nodules with highly suspected malignant PNs. There was no significant difference between the 2 groups in terms of age, sex, characteristics, nodule location, Eastern Cooperative Oncology Group (ECOG) score and so on (P>0.05) (Table 1). All the patients in Group B underwent direct MWA when those patients were first confirmed to have malignancy by PB. The technical success rate of both groups was 100%, and the malignant result rate of biopsy pathology in the synchronous group was 80.0% (24/30). The results are listed in Table 1. Among them, 11 patients required GMT, and the qualified rate of the samples was 81.8% (9/11).
The local CA rates in Group A and Group B were 100% and 97.1%, respectively, which showed no significance (P=0.26). The TPT and PED were 41.6±7.9 and 57.3±8.8 min and 12.9±1.4 and 19.4±2.3 mSv in Groups A and B, respectively, which showed significance (P<0.001). The hospitalization stay and cost were 4.7±1.3 and 9.1±2.1 days and 3,768.8±652.9 and 4,508.0±514.1 USD, respectively, and both parameters showed significance (P<0.001). More detailed information is listed in Table 2.
Table 2
Parameters | Group A (n=30) | Group B (n=35) | P value |
---|---|---|---|
Technical success rate | 100% | 100% | – |
Patient position (supine/prone/lateral position) | 18/9/3 | 20/10/5 | 0.87 |
Distance from nodule to pleural (cm) | 6.5±1.6 | 6.1±1.5 | 0.32 |
Total procedure time (min) | 41.6±7.9 | 57.3±8.8 | <0.001 |
Radical ablation cycle | 1.3±0.5 | 1.3±0.4 | 0.51 |
Radical ablation power (W) | 37.5±2.5 | 37.5±2.8 | 0.45 |
Radical ablation time (min) | 4.2±0.8 | 4.3±0.8 | 0.70 |
Irradiation dose (mSv) | 12.9±1.4 | 19.4±2.3 | <0.001 |
Complete ablation rate | 100% | 97.1% | 0.26 |
Hospitalization stay (days) | 4.7±1.3 | 9.1±2.1 | <0.001 |
Hospitalization cost (USD) | 3,768.8±652.9 | 4,508.0±514.1 | <0.001 |
Follow up time (months) | 43.6±17.2 | 39.2±18.7 | 0.33 |
Follow up treatments (C/R/others) | 12/4/18 | 14/5/20 | 0.99 |
Data are presented as mean ± standard deviation or number (frequency)/n (%). Group A underwent synchronous PB and MWA (strategy: low-power MWA-PB-high-power MWA). Group B underwent asynchronous PB and MWA. C, chemotherapy; R, radiotherapy; PB, percutaneous biopsy; MWA, microwave ablation.
The incidences of intrapulmonary hemorrhage and hemoptysis in Groups A and B were 16.7% and 41.4% and 0% and 8.6%, respectively; there was a significant difference between the 2 groups (P<0.05). The incidence of pneumothorax was 30% and 17.1%, respectively, and the incidence of pleural effusion was 26.7% and 17.1%, respectively; there were no significant differences between the 2 groups (P>0.05) (Table 3). No serious complications, such as air embolism, fatal massive hemorrhage, bronchopleural fistula, pulmonary pseudoaneurysm, or needle implantation metastasis, occurred in either group.
Table 3
Parameters | Group A (n=30) | Group B (n=70) | P value | |
---|---|---|---|---|
PB stage (n=35) | MWA stage (n=35) | |||
Intrapulmonary hemorrhage | 5 (16.7) | 24 (68.6) | 5 (14.3) | 0.02 |
Hemoptysis | 0 (0.0) | 5 (14.3) | 1 (2.9) | 0.04 |
Pneumothorax | 9 (30.0) | 8 (22.9) | 4 (11.4) | 0.16 |
Pleural effusion | 8 (26.7) | 7 (20.0) | 5 (14.3) | 0.28 |
Data are presented as n (%). Group A underwent synchronous PB and MWA (strategy: low-power MWA-PB-high-power MWA). Group B underwent asynchronous PB and MWA. PB, percutaneous biopsy; MWA, microwave ablation.
Discussion
With the improvement of people’s health awareness and the high incidence rate of malignant tumors, more PNs have been found by the widespread use of CT scans, and a large number of early lung adenocarcinomas or metastases are suspected according to the imaging morphological characteristics and the patient’s medical history (12). Therefore, how to accurately diagnose PNs and make appropriate treatment strategies has become an urgent problem to be solved. In the former, the pathological sensitivity needs to be optimized, and the treatment needs to be followed. For the latter, the nature needs to clarified to determine the tumor stage, and the GMT needs to be understood to adjust further treatment plans (13). Although the treatment and pathological results can be obtained by one-time surgery, many patients cannot undergo or refuse surgery because of subjective or objective reasons (advanced age, poor cardiopulmonary function, late tumor staging, etc.); therefore, percutaneous ablation can become an effective alternative because of its high efficiency, repeatability, fast recovery, and low cost.
However, the PN malignant probability correlates with different densities on CT. Among them, the malignant probability of some solid nodules is the highest, followed by ground glass density nodules and solid nodules (14). However, all these morphological characteristics cannot replace the gold standard status of PB. The conventional process is that PB is followed by ablation to avoid unnecessary treatment to some extent (15), but the above relatively separate puncture processes may not only increase puncture-related complications, such as hemoptysis and pneumothorax, but also increase the patient’s radiation exposure dose and hospitalization costs, which may result in long waiting times and psychological burdens. Therefore, how to combine PB with ablation has always been the key to improving efficiency.
A recent meta-analysis from Heerink et al. (6) showed that the incidence of pneumothorax and intrapulmonary hemorrhage after CT-guided PN biopsy was 34.3–43.5% and 18.2–30.8%, respectively, and the incidence of intrapulmonary hemorrhage of GGOs may exceed 60%. As the focus of PNs (especially GGOs) is small during needle biopsy, a violent cough may cause blood or air to enter the patient’s bronchus and cause hemoptysis or a gas embolism. Theoretically, the thermal ablation technology represented by MWA or RFA can occlude the blood vessels in the ablation area, which has a certain hemostatic effect (16). The combination of the 2 techniques can theoretically reduce the risk of pulmonary hemorrhage or hemoptysis, truncate the operation process, and shorten the hospital stay, so the extent to which ablation will affect the biopsy results is clinically unknown. The most recent 5 years of papers on PB followed by ablation synchronously for PNs were retrieved from the PubMed website (Table 4). All those studies (17-22) showed that the technical success rate was 100%, with rates of pneumothorax and pulmonary hemorrhage of 25–60.8% and 5–72.7%, respectively. Tselikas et al. (21) showed that 90% of patients have a malignant pathology by PB after RFA. Wang et al. (19) performed PB before and after MWA, and the diagnostic sensitivity was 85.1% and 74.3%, reaching 90.5% when 2 biopsy results were combined. Hasegawa et al. (22) performed GMT analysis using biopsy specimens obtained after RFA, and 74% of the patients benefited. The antigens weakened but did not disappear, which should be considered when performing immunohistochemistry. Chi et al. (23) reported that 17G coaxial punctures were performed on 31 cases of GGOs (mean diameter 12 mm), and biopsies were performed before and after low-power (20 W) MWA. The pathological diagnosis of biopsy after MWA was significantly different from that before MWA (100% vs. 75%, P=0.008). Low-power ablation can improve the biopsy sensitivity of GGOs by 25%. Therefore, low-power MWA at the same time may play a dual role in GGO lesions by providing hemostasis and improving the biopsy sensitivity due to local cell aggregation.
Table 4
References | Design | Sample | Mean age (years) | Mean diameter (cm) | Nodule characteristics | Biopsy sensitivity (%) | Complications | Complete ablation rate (%) |
---|---|---|---|---|---|---|---|---|
Kong et al., 2021, China (17) | Rs | 66 | 64.1 | 1.38 | Ground-glass opacities | 69.7 | Pneumothorax (36.4%)/ hemorrhage (72.7%) |
100.0 |
Wang et al., 2019, China (18) | Rs | 74 | 61.3 | 1.71 | Ground-glass opacities | 85.1 (Group A1)/ 74.3 (Group B1) |
Pneumothorax (60.8%)/ hemoptysis (32.4%)/ pleural effusion (52.7%)/ pulmonary infection (13.5%) |
– |
Wang et al., 2019, China (19) | Rs | 54 | 65.5 | 2.1 (Group A2)/ 2.2 (Group B2) |
NA | 88.9 (Group A2)/ 100.0 (Group B2) |
Pneumothorax (29.6% vs. 57.1%)/ hemoptysis (22.2% vs. 20.0%) |
100.0 |
Wei et al., 2018, China (20) | Rs | 68 | 67.0 | NA | NA | 85.3 (Group A3)/ 69.1 (Group B3) |
Major complications (25.0%)/ minor complications (35.3%) |
78.9 (Group A3)/ 71.4 (Group B3) |
Tselikas et al., 2017, France (21) | Rs | 20 | 62.7 | 1.73 | Solid nodules | 90.0 | Pneumothorax (25.0%)/ hemoptysis (5.0%) |
95.0 |
Hasegawa et al., 2016, Japan (22) | Rs | 3 | 69.3 | 1.93 | Solid nodules | 100.0 | Pneumothorax (66.7%) | 66.7 |
Rs, retrospective study; Group A1, pre-microwave ablation; Group B1, post-microwave ablation; Group A2, synchronous mode; Group B2, asynchronous mode; NA, not applicable; Group A3, post-microwave ablation pathology as pre-microwave ablation; Group B3, paired histology as pre-microwave ablation biopsy.
The present study showed that the technical success and CA rate of PB and MWA synchronously were both 100%, with a 3.3% hemoptysis rate and a 16.7% intrapulmonary hemorrhage rate. The biopsy sensitivity and GMT were 80% and 81.8%, respectively, which is equivalent to the relevant data reported in previous CT-guided needle biopsies (73–96%) (24,25).
Excessive ablation may lead to local tissue carbonization and destruction of the double strand structure of tissue DNA. Low power/short time thermal MWA was used to solidify local tissues without destroying the tumor cell target antigen. Biopsy immediately after thermal ablation will still allow the tissue to retain the original cell morphology. Therefore, pathological and genetic examination of biopsy tissues after thermal ablation is feasible. The experience of our center is as follows (26,27): (I) it is very important to reasonably control the temperature ablation time during MWA. The local temperature of the low power/short time mode generally does not exceed 80°, and the DNA activity in the tissue can still be retained (II). The temperature destroys the fourth-order folding structure of proteins, but the first-order amino acid structure remains. The antigenicity might lead to the weakening of immunohistochemistry, but it does not affect the diagnostic results (III). The tumor components in the PNs are uneven. Low-power WMA is characterized by shrinkage of the cell components and an increase in the local cell components. For mixed nodules and GGO biopsy, the tissue sample content can be increased theoretically.
Pneumothorax and hemorrhage are the most common complications of PB. This study shows that intraoperative PB and MWA do not increase the incidence of pneumothorax but significantly reduce the risk of bleeding, especially hemoptysis. The TPT and PED in Group A decreased by 27.4% and 33.5%, respectively, because the positioning and puncture of the MWA applicator and biopsy needle can be carried out synchronously. The hospitalization stay and costs in Group A were decreased by 48.4% and 16.4%, respectively, which showed that synchronous MWA and PB are feasible to improve the efficiency of the treatment plan for PNs.
The study also had some limitations. The sample size of this study was small, with a high case bias limiting the accuracy of statistical results, a retrospective design, and a single-center experience, and patient cost comparison between both groups only referred to the direct hospital cost without considering different regions medical charges, insurance payment, and indirect patient costs during hospitalization. Although all case enrollments were from 2 different periods, past ordinary operation experience suggests that the more punctures, the higher the incidence of pneumothorax. Therefore, subjective intention of the operator may have contributed to the selection bias. Further prospective, multicenter, randomized controlled research evidence is needed.
Acknowledgments
Funding: This work was supported by
Footnote
Reporting Checklist: The authors have completed the STROBE reporting checklist. Available at https://qims.amegroups.com/article/view/10.21037/qims-23-138/rc
Conflicts of Interest: All authors have completed the ICMJE uniform disclosure form (available at https://qims.amegroups.com/article/view/10.21037/qims-23-138/coif). The authors have no conflicts of interest to declare.
Ethical Statement: The authors are accountable for all aspects of the work in ensuring that questions related to the accuracy or integrity of any part of the work are appropriately investigated and resolved. The study was conducted in accordance with the Declaration of Helsinki (as revised in 2013). This study was approved by the institutional review board, and informed consent was provided by all the participants.
Open Access Statement: This is an Open Access article distributed in accordance with the Creative Commons Attribution-NonCommercial-NoDerivs 4.0 International License (CC BY-NC-ND 4.0), which permits the non-commercial replication and distribution of the article with the strict proviso that no changes or edits are made and the original work is properly cited (including links to both the formal publication through the relevant DOI and the license). See: https://creativecommons.org/licenses/by-nc-nd/4.0/.
References
- Wu F, Wang L, Zhou C. Lung cancer in China: current and prospect. urrO pin Oncol 2021;33:40-6.
- Henschke CI, Yankelevitz DF, Libby DM, Pasmantier MW, Smith JP, Miettinen OS. Survival of patients with stage I lung cancer detected on CT screening. N Engl J Med 2006;355:1763-71. [Crossref] [PubMed]
- Aberle DR, Adams AM, Berg CD, Black WC, Clapp JD, Fagerstrom RM, Gareen IF, Gatsonis C, Marcus PM, Sicks JD. Reduced lung-cancer mortality with low-dose computed tomographic screening. N Engl J Med 2011;365:395-409. [Crossref] [PubMed]
- Jiao D, Ren K, Li Z, Shui S, Han X. Clinical role of guidance by C-arm CT for (125)I brachytherapy on pulmonary tumors. Radiol Med 2017;122:829-36. [Crossref] [PubMed]
- Li Z, Hao D, Jiao D, Zhang W, Han X. Transcatheter Arterial Chemoembolization Combined with Simultaneous Cone-beam Computed Tomography-guided Microwave Ablation in the Treatment of Small Hepatocellular Carcinoma: Clinical Experiences From 50 Procedures. Acad Radiol 2021;28:S64-70.
- Heerink WJ, de Bock GH, de Jonge GJ, Groen HJ, Vliegenthart R, Oudkerk M. Complication rates of CT-guided transthoracic lung biopsy: meta-analysis. Eur Radiol 2017;27:138-48. [Crossref] [PubMed]
- Sachdeva M, Ronaghi R, Mills PK, Peterson MW. Complications and Yield of Computed Tomography-Guided Transthoracic Core Needle Biopsy of Lung Nodules at a High-Volume Academic Center in an Endemic Coccidioidomycosis Area. Lung 2016;194:379-85. [Crossref] [PubMed]
- Kong F, Wang C, Li Y, Li X. Advances in study of the sequence of lung tumor biopsy and thermal ablation. Thorac Cancer 2021;12:279-86. [Crossref] [PubMed]
- Gao X, Tian Z, Cheng Y, Geng B, Chen S, Nan Q. Experimental and numerical study of microwave ablation on ex-vivo porcine lung. Electromagn Biol Med 2019;38:249-61. [Crossref] [PubMed]
- Clasen S, Krober SM, Kosan B, Aebert H, Fend F, Bomches A, Claussen CD, Pereira PL. Pathomorphologic evaluation of pulmonary radiofrequency ablation: proof of cell death is characterized by DNA fragmentation and apoptotic bodies. Cancer 2008;113:3121-9. [Crossref] [PubMed]
- Ye X, Fan W, Wang Z, Wang J, Wang H, Wang J, et al. Expert consensus on thermal ablation therapy of pulmonary subsolid nodules (2021 Edition). J Cancer Res Ther 2021;17:1141-56. [Crossref] [PubMed]
- Guo X, Li Y, Yang C, Hu Y, Zhou Y, Wang Z, Zhang L, Hu H, Wu Y. Deep Learning-Based Computed Tomography Imaging to Diagnose the Lung Nodule and Treatment Effect of Radiofrequency Ablation. J Healthc Eng 2021;2021:6556266. [Crossref] [PubMed]
- Bao F, Yu F, Wang R, Chen C, Zhang Y, Lin B, Wang Y, Hao X, Gu Z, Fang W. Electromagnetic bronchoscopy guided microwave ablation for early stage lung cancer presenting as ground glass nodule. Transl Lung Cancer Res 2021;10:3759-70. [Crossref] [PubMed]
- Blackmon SH, Sterner RM, Eiken PW, Vogl TJ, Pua BB, Port JL, Dupuy DE, Callstrom MR. Technical and safety performance of CT-guided percutaneous microwave ablation for lung tumors: an ablate and resect study. J Thorac Dis 2021;13:6827-37. [Crossref] [PubMed]
- Callister ME, Baldwin DR, Akram AR, Barnard S, Cane P, Draffan J, Franks K, Gleeson F, Graham R, Malhotra P, Prokop M, Rodger K, Subesinghe M, Waller D, Woolhouse I. British Thoracic Society guidelines for the investigation and management of pulmonary nodules. Thorax 2015;70:ii1-ii54. [Crossref] [PubMed]
- Zhong CH, Fan MY, Xu H, Jin RG, Chen Y, Chen XB, Tang CL, Su ZQ, Li SY. Feasibility and Safety of Radiofrequency Ablation Guided by Bronchoscopic Transparenchymal Nodule Access in Canines. Respiration 2021;100:1097-104. [Crossref] [PubMed]
- Kong F, Bie Z, Li Y, Li B, Guo R, Wang C, Peng J, Xu S, Li X. Synchronous microwave ablation followed by core-needle biopsy via a coaxial cannula for highly suspected malignant lung ground-glass opacities: A single-center, single-arm retrospective study. Thorac Cancer 2021;12:3216-22. [Crossref] [PubMed]
- Wang J, Ni Y, Yang X, Huang G, Wei Z, Li W, Han X, Meng M, Ye X, Lei J. Diagnostic ability of percutaneous core biopsy immediately after microwave ablation for lung ground-glass opacity. J Cancer Res Ther 2019;15:755-9. [Crossref] [PubMed]
- Wang D, Li B, Bie Z, Li Y, Li X. Synchronous core-needle biopsy and microwave ablation for highly suspicious malignant pulmonary nodule via a coaxial cannula. J Cancer Res Ther 2019;15:1484-9. [Crossref] [PubMed]
- Wei Z, Wang Q, Ye X, Yang X, Huang G, Li W, Wang J, Han X, Meng M, Yang N, Li Q. Microwave ablation followed by immediate biopsy in the treatment of non-small cell lung cancer. Int J Hyperthermia 2018;35:262-8. [Crossref] [PubMed]
- Tselikas L, de Baere T, Deschamps F, Hakimé A, Besse B, Teriitehau C, de Montpreville V, Adam J. Diagnostic yield of a biopsy performed immediately after lung radiofrequency ablation. Eur Radiol 2017;27:1211-7. [Crossref] [PubMed]
- Hasegawa T, Kondo C, Sato Y, Inaba Y, Yamaura H, Kato M, Murata S, Onoda Y, Kuroda H, Sakao Y, Yatabe Y. Diagnostic Ability of Percutaneous Needle Biopsy Immediately After Radiofrequency Ablation for Malignant Lung Tumors: An Initial Experience. Cardiovasc Intervent Radiol 2016;39:1187-92. [Crossref] [PubMed]
- Chi J, Ding M, Wang Z, Hu H, Shi Y, Cui D, Zhao X, Zhai B. Pathologic Diagnosis and Genetic Analysis of Sequential Biopsy Following Coaxial Low-Power Microwave Thermal Coagulation For Pulmonary Ground-Glass Opacity Nodules. Cardiovasc Intervent Radiol 2021;44:1204-13. [Crossref] [PubMed]
- Han Y, Kim HJ, Kong KA, Kim SJ, Lee SH, Ryu YJ, Lee JH, Kim Y, Shim SS, Chang JH. Diagnosis of small pulmonary lesions by transbronchial lung biopsy with radial endobronchial ultrasound and virtual bronchoscopic navigation versus CT-guided transthoracic needle biopsy: A systematic review and meta-analysis. PloS One 2018;13:e0191590. [Crossref] [PubMed]
- Zhang JH, Xia FF, Yang XS, Li Y. Computed tomography-guided lung biopsy for molecular tests: a meta-analysis. Kardiochir Torakochirurgia Pol 2022;19:96-101. [Crossref] [PubMed]
- Huang G, Yang X, Li W, Wang J, Han X, Wei Z, Meng M, Ni Y, Zou Z, Wen Q, Dai J, Zhang T, Ye X. A feasibility and safety study of computed tomography-guided percutaneous microwave ablation: a novel therapy for multiple synchronous ground-glass opacities of the lung. Int J Hyperthermia 2020;37:414-22. [Crossref] [PubMed]
- Li X, Ye X. Computed tomography-guided percutaneous core-needle biopsy after thermal ablation for lung ground-glass opacities: Is the method sound? J Cancer Res Ther 2019;15:1427-9. [Crossref] [PubMed]