Potential of unilateral combined bypass surgery to accelerate contralateral radiological progression in pediatric moyamoya disease
Introduction
Moyamoya disease (MMD) is one of the most common causes of childhood strokes (1,2). It is characterized by progressive stenosis and/or occlusion of the bilateral internal carotid artery (ICA) and/or its main branches, leading to compensatory collateral vessel development in the deep brain: the so called moyamoya vessels (2). The incidence of MMD is greatest between the ages of 40 and 45 years, with half of these patients presenting with intracranial bleeding; another peak is between 5 and 10 years of age, which is referred to as pediatric MMD, with these children usually presenting with ischemic attack (3).
Surgical cerebral revascularization is recommended as the treatment for pediatric MMD (4,5). Previous studies have reported favorable outcomes after surgery, with low ischemic risks on the operated (OP) hemisphere (6-8). Collectively, direct and indirect bypass surgery are two mainstream strategies for MMD. Direct bypass anastomosizes the donor and recipient arteries directly, resulting in increased cerebral blood flow immediately after surgery (9). Indirect bypass attaches a well-perfused tissue to the surface of ischemic hemisphere, leading to angiogenesis that alleviates ischemia. In combined bypass surgery, both strategies are applied (10).
Patients with pediatric MMD who have undergone successful unilateral revascularization do not know whether further operation will occur or when another side will be OP on, owing to the progression pace on the non-OP side being unclear (11). Some pediatric patients with MMD are unstable, and their disease course consists of a rapid progression of a steno-occlusive lesion. It is interesting to note that younger age is associated with such instability (12). Consequently, it is unknown whether the contralateral sides are stable for patients with pediatric MMD who have undergone unilateral combined bypass surgery. Currently, there is little data to indicate whether unilateral bypass surgery would accelerate disease progression on the contralateral side, particularly in pediatric patients. To address this issue, we performed a series of studies. Our previous research examined rapid radiological progression of contralateral vessels at short-term follow-up after unilateral combined bypass surgery in pediatric MMD (13). In study described here, we aimed to observe the progression pace on the non-OP side of patients with pediatric MMD and identify any related factors risk factors. We present this article in accordance with the STROBE reporting checklist (available at https://qims.amegroups.com/article/view/10.21037/qims-23-105/rc).
Methods
Study design and participants
The study was conducted in accordance with the Declaration of Helsinki (as revised in 2013) and was approved by the Institutional Review Board of Xuanwu Hospital, Capital Medical University. Written informed consent was obtained from the patients’ legal guardians. We conducted a retrospective study of an analysis of the data of patients with MMD treated in a national center for neurological disease from June 2019 to July 2021. The inclusion criteria were the following: (I) pediatric patients diagnosed with MMD with digital subtraction angiography (DSA) according to the 2012 Japanese guidelines (14); (II) patients younger than 19 years old (as applied in the International Pediatric Stroke Study) (2); (III) patients with neurological symptoms related to MMD; (IV) patients who had undergone a combined anastomosis of superficial temporal artery and middle cerebral artery (STA-MCA) bypass and encephaloduroarteriosynangiosis (EDAS); and (V) patients with postoperative follow-up between 3 and 6 months. Meanwhile, the exclusion criteria were the following: (I) history of prior bypass surgeries; (II) hemorrhagic stroke; and (III) diagnosis of moyamoya syndrome.
Combined revascularization
Patients underwent with general anesthesia and tracheal intubation in the supine position. The head was placed on a headrest, with the body turned to the side opposite the operation site. After disinfection and placement of surgical drapes in the operative area, the frontal and parietal branches of the STA as a donor vessel on the surgical side were exposed and separated under microscopy. The proximal end of the parietal branch was temporarily blocked, and then the distal end of the parietal branch was cut. Following this, the temporalis muscle was arcuately incised, and a bone window was formed with a drill and milling cutter. The dura mater was exposed, and the arachnoid was incised along with a branch of the MCA (M4 segment) that matched the diameter of the parietal branch of STA. The branch of the M4 segment was blocked, and an incision was made along the long axis of the artery wall with a diameter matching that of the donor vessel. After flushing with heparin saline and staining with methylene blue were completed, the parietal branch was intermittently sutured to the M4 segment of the MCA using 10-0 sutures for a total of 8–10 stitches, and the temporary blocking clamp was removed after completion of the anastomosis. The anastomotic site and the STA were carefully hemostasized, and EDAS was performed. The intact frontal branch of the STA was attached to the brain surface with a strip of galea. The dura mater was divided into an outer and inner layer, with the meningeal artery left intact as much as possible. The inter layer of dura mater was turned over and attached to the brain surface, while the outer layer of dura mater was in situ sutured to maintain the integrity of dura mater. Finally, the skull, temporalis muscle, and scalp were closed. The patient was transferred back to the ward and received treatment for blood pressure control, fluid replacement, and prophylaxis against seizures.
Variables and radiological progression evaluation
A number of demographic and clinical variables, including age, sex, surgical side, angiographic findings, hemodynamic data, perioperative complications, and follow-up outcomes, were collected. Angiographic data including Suzuki stage, moyamoya vessel grade, and degree of neovascularization were evaluated independently by two neuroradiologists (Y Ma and X Lu), and any disagreement was settled by discussion with another neuroradiologist (B Yang) (13).
Radiological progression was evaluated using two methods on the non-OP side, the Suzuki angiographic staging system (15) and a grading system of moyamoya vessels (Table 1). According to the system proposed by Morioka et al. (16) and Yamamoto et al. (17), the development of moyamoya vessels can be classified into three grades. Due to the connection between the advancement of moyamoya vessels and medullary perforating artery, the grading system of Morioka et al. and Yamamoto et al. was eventually modified by adding a fourth grade: visualization of medullary arteries on the basis of grade 3 (18). Since the direct bypass graft itself connects the extracranial and intracranial regions and could subsequently interfere with the Suzuki staging evaluation, the change of the Suzuki stage in the OP side upon follow-up was not evaluated.
Table 1
Grade | Content |
---|---|
The Suzuki angiographic staging | |
I | Narrowed ICA bifurcation |
II | Narrowed ICA bifurcation, dilated ACA and MCA, and moyamoya vessels |
III | Narrowed ICA bifurcation, ACA and MCA, and further increase in moyamoya vessels |
IV | Occluded ICA, tenuous ACA and MCA, obvious extra-intracranial collaterals from ECA, and decrease in moyamoya vessels |
V | Occluded ICA, ACA, and MCA, along with further decrease in moyamoya vessels |
VI | Brain totally supplied by the ECA |
Grading system of moyamoya vessels | |
0 | The perforating arteries do not dilate |
1 | The perforating arteries dilate but are restricted within their original territory |
2 | The perforating arteries dilate abnormally and provide collateral blood flow to the regions beyond their original territory, while the medullary artery remains invisible |
3 | The medullary arteries are visible on the basis of grade 2 |
Classification of neovascularization on the OP side | |
Poor | Bypass vessels supply less than one-third of the MCA territory |
Good | Bypass vessels supply more than one-third of the MCA territory |
ICA, internal carotid artery; ACA, anterior cerebral artery; MCA, middle cerebral artery; ECA, external carotid artery; OP, operated.
Degree of neovascularization and hemodynamic evaluation
According to the scheme proposed by Matsushima et al. (19), the amount of neovascularization on the OP hemisphere was classified into three levels. We divided the neovascularization grade into two categories, poor and good, to improve the consistency of evaluation (Table 1).
To assess regional cerebral blood flow (rCBF) qualitatively and quantitatively, magnetic resonance imaging (MRI) with arterial spin labeling (ASL) was used (20) with a 3.0-T MRI scanner (Discovery MR750, GE HealthCare, Saint Louis, MO, USA) and a standard 8-channel head coil. Image acquisition of ASL was completed via the pulsed ASL (pASL) technique. The specific parameters were as follows: repetition time/echo time (TR/TE) =5,335/10.7 ms, slice thickness =4 mm, slice number =36, the voxel size =1.88×1.88×4.00 mm3, and scan time =5.10 min. The conversion and quantitative calculation of rCBF images were based on formulae described in the literature (21). In pediatric patients, MRI-ASL is preferred since it does not involve radiation exposure or contrast agents (22). We have previously discussed the reliability of MRI-ASL in pediatric patients (23). rCBF was measured in all enrolled children prior to surgery and at follow-up.
Statistical analyses
Continuous variables with a normal distribution are expressed as the mean ± standard deviation (SD) and analyzed using Student t-test. The Mann-Whitney test was used to assess nonnormally distributed variables which are expressed as medians with interquartile range (IQR). Categorical variables were compared with paired Wilcoxon rank-sum tests and χ2 test. Kaplan-Meier analysis was employed to draw a survival curve. To explore factors associated with radiological progression, univariate analysis was conducted. All analyses were carried out using SPSS 23.0 (IBM Corp., Armonk, NY, USA). Statistical significance was set at a two-sided P value <0.05.
Results
Patient characteristics
The process of patient selection is presented in Figure S1. Ultimately, 27 patients with a median age of 8 (IQR, 5–14) years old were identified, among whom 12 (44.4%) were male and 16 (59.3%) were first OP on the left side. On the OP side, the majority of patients were Suzuki stage 3 (40.74%) or 4 (48.15%) before the operation (Table 2). The rCBFs on the OP side were lower than those on the non-OP side. No patient experienced complications during the perioperative period. The median interval between unilateral combined bypass surgery and a subsequent postoperative reevaluation was 4.7 (IQR, 3.7–5.7) months. Details are presented in Table S1.
Table 2
Characteristics | Pre-OP (n=27) | FU (n=27) | P value |
---|---|---|---|
OP side | |||
rCBF (mL/100 g/min) | 41.50 (37.90–46.90) | 60.20 (50.50–66.00) | <0.001† |
Moyamoya vessel grade (points) | <0.001‡ | ||
0 | 1 (3.70) | 2 (7.41) | |
1 | 5 (18.52) | 21 (77.78) | |
2 | 6 (22.22) | 3 (11.11) | |
3 | 15 (55.56) | 1 (3.70) | |
Suzuki stage | |||
I | 1 (3.70) | ||
II | 2 (7.41) | ||
III | 11 (40.74) | ||
IV | 13 (48.15) | ||
Non-OP side | |||
rCBF (mL/100 g/min) | 50.20 (43.50–59.30) | 49.60 (42.90–61.10) | 0.445† |
Moyamoya vessel grade (points) | 0.005‡ | ||
0 | 3 (11.11) | 1 (3.70) | |
1 | 8 (29.63) | 8 (29.63) | |
2 | 12 (44.44) | 7 (25.93) | |
3 | 4 (14.81) | 11 (40.74) | |
Suzuki stage | 0.034‡ | ||
I | 4 (14.81) | 2 (7.41) | |
II | 5 (18.52) | 6 (22.22) | |
III | 10 (37.04) | 8 (20.63) | |
IV | 8 (29.63) | 11 (40.74) |
Data are presented as median (IQR) or n (%). †, analyzed with Mann-Whitney test; ‡, analyzed with paired Wilcoxon rank-sum test. Pre-OP, preoperative; FU, follow-up; OP, operated; rCBF, regional cerebral blood flow; IQR, interquartile range.
Angiographic and hemodynamic results
Angiographic and hemodynamic changes are summarized in Table 2. On the OP side, the moyamoya vessel grade decreased significantly (P<0.001) and rCBF increased significantly after surgery as compared with before surgery [preoperative (pre-OP) vs. follow-up: median (IQR), 60.20 (50.50–66.00) vs. 41.50 (37.90–46.90) mL/100 g/min, P<0.001]. On the non-OP side, compared with those before surgery, moyamoya vessel grade (P=0.005) and Suzuki stage (P=0.034) increased significantly after surgery, while the rCBF barely changed after contralateral surgery [pre-OP vs. follow-up: median (IQR), 50.20 (43.50–59.30) vs. 49.60 (42.90–61.10) mL/100 g/min, P=0.445]. Moreover, 11 patients (40.7%) experienced progression on the non-OP side. The cumulative risk of contralateral progression (Figure 1) indicated a high risk of radiological progression on the non-OP side over time. Among these 11 patients, all experienced an increase in moyamoya vessel grade, and 5 progressed in both moyamoya vessel grade and Suzuki stage.
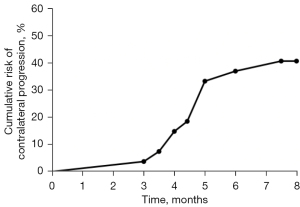
Univariate analysis for progression on non-OP side
A summary of the principal results of the univariate analysis for demographic and clinical factors affecting progression on the non-OP side in provided in Table 3. An additional analysis of the non-OP side with the angiographic data of OP side is presented in Table S2. The earlier the stage of pre-OP Suzuki stage on the non-OP side was associated with an increased risk of non-OP progression (P=0.007). At follow-up, 15 patients (55.6%) experienced ischemic attacks or recent infarction in the non-OP hemisphere. Neither the occurrence of ischemic attacks on the non-OP side (P=0.816) nor the degree of neovascularization on the OP side (P=1.000) was associated with non-OP radiological progression.
Table 3
Characteristics | Non-OP side progression | P value | |
---|---|---|---|
Yes (n=11) | No (n=16) | ||
Age (years) | 8 (6.0–11.5) | 8 (7.8–12.0) | 0.484† |
Follow-up (months) | 5.0 (4.0–5.0) | 4.0 (4.0–5.3) | 0.544† |
Sex, male | 4 (36.4) | 8 (50.0) | 0.696‡ |
OP side, left | 7 (43.8) | 9 (56.3) | >0.99‡ |
Pre-OP PCA stenosis/occlusion | |||
OP side | 0 (0.0) | 6 (37.5) | 0.054‡ |
Non-OP side | 1 (9.1) | 2 (12.5) | >0.99‡ |
Pre-OP rCBF (mL/100 g/min) | |||
OP side | 39.6 (37.9–41.9) | 42.8 (40.2–40.1) | 0.247† |
Non-OP side | 50.2 (47.1–67.3) | 49.7 (42.2–57.1) | 0.387† |
OP/non-OP | 0.767±0.125 | 0.878±0.164 | 0.071§ |
Pre-OP Suzuki stage | |||
Non-OP side | 0.007‡ | ||
I | 3 (27.3) | 1 (6.3) | |
II | 4 (36.4) | 1 (6.3) | |
III | 4 (36.4) | 6 (37.5) | |
IV | 0 (0.0) | 8 (50.0) | |
Pre-OP moyamoya vessel grade (points) | |||
Non-OP side | 0.123‡ | ||
0 | 2 (18.2) | 1 (6.3) | |
1 | 2 (18.3) | 6 (37.5) | |
2 | 7 (63.6) | 5 (31.3) | |
3 | 0 (0.0) | 4 (25.0) | |
Degree of neovascularization at follow-up | |||
OP side | >0.99‡ | ||
Poor | 1 (9.1) | 2 (12.5) | |
Good | 10 (90.9) | 14 (87.5) | |
Clinical outcome at follow-up | |||
Non-OP side | |||
Recent infarction | 1 (9.1) | 2 (12.5) | >0.99‡ |
Ischemic attacks | 6 (54.5) | 8 (50.0) | 0.816¶ |
Data are presented as median (IQR), n (%), or mean ± SD. †, analyzed with Mann-Whitney test; ‡, analyzed with Fisher exact test; §, analyzed with independent samples t-test; ¶, χ2 test. OP, operated; pre-OP, preoperative; PCA, posterior cerebral artery; rCBF, regional cerebral blood flow; IQR, interquartile range; SD, standard deviation.
Cases illustration
Case presentation 1
A 4-year-old girl had been experiencing paroxysmal weakness of the right hand for 7 months and blurred speech for 2 months. She was diagnosed with pediatric MMD and underwent a successful STA-MCA bypass combined with EDAS on the left side. Three months later, she was admitted due to weakness of her left upper extremity. The DSA revealed no increase in terms of the Suzuki stage (stage 2), but the moyamoya vessel grade increased from 2 to 3 on the non-OP side (Figure 2A,2B), indicating progression. Meanwhile, her pre-OP Suzuki stage was 3 (Figure 2C). The grade of moyamoya vessels decreased from 2 to 1 (Figure 2D,2E), and the degree of neovascularization was good (Figure 2F).
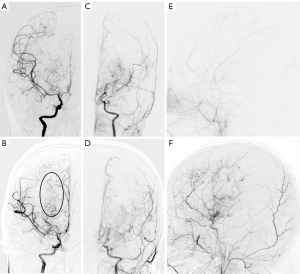
Case presentation 2
A 5-year-old girl had been experiencing paroxysmal numbness of left hand after crying for 6 months. She was diagnosed with pediatric MMD and underwent a successful STA-MCA bypass combined with EDAS on the right side. Four months later, the DSA revealed that the stenosis in the ICA and MCA had progressed to occlusion (Figure 3A,3B). At follow-up examination, the MMD stage on the non-OP side was found to have progressed from Suzuki stage 1 to 3 (Figure 3A,3B). As for the OP side, the grade of moyamoya vessels decreased from 2 to 1 (Figure 3C,3D), and the degree of neovascularization was good (Figure 3E,3F).
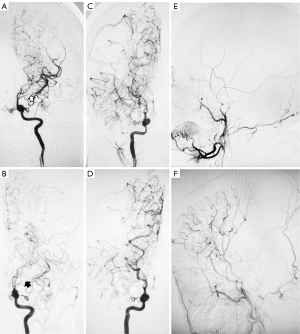
Discussion
This study investigated the changes before and after unilateral combined bypass surgery in patients with pediatric MMD. We found that combined surgical revascularization effectively increased the rCBF and decreased the moyamoya vessel grade of the OP side. Furthermore, within short-term follow-up, the rCBF on the non-OP side changed minimally, but the Suzuki stage or moyamoya vessel grade deteriorated in 40.7% of the patients. The radiological progression preceded the decrease in rCBF.
The radiological progression pace is relatively slow in patients with MMD over the course of their natural history. Ge et al. (24) retrospectively investigated 110 patients with MMD and reported that only 5 (4.5%) cases progressed in Suzuki stage or the posterior cerebral artery collaterals during a median follow-up of 6 months in the natural course. Among the 5 cases, only 1 was pediatric and progressed after 29 months of follow-up. In the study of Lee et al. (25), contralateral disease progression, as assessed by newly developed stenosis at the terminal ICA, the proximal MCA, or anterior cerebral artery, was evident in 43 (29.5%) patients with MMD with unilateral stenosis after a mean follow-up of 4.3±2.4 years. They also found pediatric patients were more likely to develop contralateral progression. To examine the relationship between surgical treatment and MMD progression, Park et al. conducted a retrospective study focusing on patients with pediatric MMD treated by indirect revascularization and found that 20 of 34 patients (58.8%) had contralateral progression following surgery, and the mean follow-up duration was long (35.3 months, IQR, 18.1–100 months) (26). Our previous studies have demonstrated that unilateral combined bypass surgery is associated with rapid radiological progression of contralateral vessels at short-term follow-up and can result in the rapid aggravation of main trunk stenosis and decreased cerebral blood flow on the non-OP side (11,13). In the current study, we identified contralateral radiological progression in 11 pediatric patients (40.7%) within 3 to 6 months following unilateral combined bypass surgery. As compared to that reported in other literature, the pace of contralateral progression was rapid when pediatric MMD patients treated by combined cerebral revascularization (24-26). There is a strong possibility that combined cerebral revascularization plays a significant role in MMD progression.
The factors affecting MMD progression have received widespread attention (26-28). Younger age has been reported to be associated with faster progression (11,25). Our study found that the earlier Suzuki stage on the non-OP side was associated with contralateral rapid progression. Based on these findings, we speculate that two mechanisms may contribute to this progression. First, the initial Suzuki stage should be viewed as a starting point of disease with greater potential for progression. According to Kim et al. (29), cortical microvascularization can be observed even at the very early stage of MMD before main trunk occlusion, indicating that active neovascularization is occurring at this stage. In our cohort, all 11 patients with non-OP side progression showed an increase in moyamoya vessel grade. Only 5 of these patients progressed in the Suzuki stage. Cortical microvascularization and moyamoya vessels represent angiogenesis, which refers to the remodeling of an existing artery to increase its luminal diameter in response to maintaining cerebral blood flow. This capacity for vascular change and remodeling might be powerful at the early stage of the disease. Second, a mechanism involving molecular signals should be considered. Nitric oxide relaxes smooth muscle cells and reduces the muscular tone of arterial walls (30). Suzuki et al. reported that successful combined bypass surgery suppressed nitric oxide release from the endothelium (31). In addition, Noda et al. found that the concentrations of nitric oxide metabolites were significantly reduced after the second combined bypass surgery as compared to the first operation (32). Therefore, combined revascularization on 1 side could increase the tension of the arterial wall on the contralateral side, deteriorating the arterial stenosis via nitric oxide-mediated vasodilation and control of cerebral circulation. Other cytokines, such as ring finger protein 213 (RNF213) and cellular retinoic acid-binding protein 1 (CRABP1), may also participate in this process, but their roles in disease progression require further investigation (33,34).
Our study has some limitations. First, a retrospective, single-center design was employed. There are inherent biases associated with a retrospective study, including potential selection bias. Given the small sample size, multivariate analysis was not possible, and this should be conducted in a future study. Second, more detailed serial DSA studies could not be performed in pediatric patients. To demonstrate the changes in the main trunk and moyamoya vessels, superselective serial DSA may be needed. Third, we used published data that included both adult and pediatric patients and selected the data of the pediatric patients for comparison, as the natural history of MMD progression in pediatric patients was less clear.
Conclusions
In pediatric MMD, unilateral combined bypass surgery might accelerate the radiological progression on the non-OP side, which occurs prior to the decline of rCBF. Earlier pre-OP Suzuki stage on the non-OP side may be risk factor for rapid radiological progression. Therefore, following unilateral combined revascularization in patients with pediatric MMD, surgeons should be alert to the possibility of rapid progression on the contralateral side.
Acknowledgments
The authors would like to thank Dr. Peng Sun, Dr. Yang Liu, and N. P. Man Li (Department of Neurosurgery, Xuanwu Hospital, Capital Medical University, Beijing, China) for their help in clinical data collection as well as Dr. Bixiao Cui (Department of Radiology and Nuclear Medicine, Xuanwu Hospital, Capital Medical University, Beijing, China) for his contribution in collecting the MRI-ASL data.
Funding: This work was supported by
Footnote
Reporting Checklist: The authors have completed the STROBE reporting checklist. Available at https://qims.amegroups.com/article/view/10.21037/qims-23-105/rc
Conflicts of Interest: All authors have completed the ICMJE uniform disclosure form (available at https://qims.amegroups.com/article/view/10.21037/qims-23-105/coif). The authors have no conflicts of interest to declare.
Ethical Statement: The authors are accountable for all aspects of the work in ensuring that questions related to the accuracy or integrity of any part of the work are appropriately investigated and resolved. The study was conducted in accordance with the Declaration of Helsinki (as revised in 2013) and was approved by the Institutional Review Board of Xuanwu Hospital, Capital Medical University. Written informed consent was obtained from the patients’ legal guardians.
Open Access Statement: This is an Open Access article distributed in accordance with the Creative Commons Attribution-NonCommercial-NoDerivs 4.0 International License (CC BY-NC-ND 4.0), which permits the non-commercial replication and distribution of the article with the strict proviso that no changes or edits are made and the original work is properly cited (including links to both the formal publication through the relevant DOI and the license). See: https://creativecommons.org/licenses/by-nc-nd/4.0/.
References
- Fullerton HJ, Wintermark M, Hills NK, Dowling MM, Tan M, Rafay MF, Elkind MS, Barkovich AJ, deVeber GA. VIPS Investigators. Risk of Recurrent Arterial Ischemic Stroke in Childhood: A Prospective International Study. Stroke 2016;47:53-9. [Crossref] [PubMed]
- Lee S, Rivkin MJ, Kirton A, deVeber G, Elbers J. International Pediatric Stroke Study. Moyamoya Disease in Children: Results From the International Pediatric Stroke Study. J Child Neurol 2017;32:924-9. [Crossref] [PubMed]
- Kuroda S, Houkin K. Moyamoya disease: current concepts and future perspectives. Lancet Neurol 2008;7:1056-66. [Crossref] [PubMed]
- Wakai K, Tamakoshi A, Ikezaki K, Fukui M, Kawamura T, Aoki R, Kojima M, Lin Y, Ohno Y. Epidemiological features of moyamoya disease in Japan: findings from a nationwide survey. Clin Neurol Neurosurg 1997;99:S1-5. [Crossref] [PubMed]
- Ibrahimi DM, Tamargo RJ, Ahn ES. Moyamoya disease in children. Childs Nerv Syst 2010;26:1297-308. [Crossref] [PubMed]
- Piao J, Wu W, Yang Z, Yu J. Research Progress of Moyamoya Disease in Children. Int J Med Sci 2015;12:566-75. [Crossref] [PubMed]
- Ong JA, Low SY, Seow WT, Goh CP, Yeo TT, Chou N, Low DC, Nga V. Revascularisation surgery for paediatric moyamoya disease: The Singapore experience. J Clin Neurosci 2020;82:207-13. [Crossref] [PubMed]
- Vilela MD, Newell DW. Superficial temporal artery to middle cerebral artery bypass: past, present, and future. Neurosurg Focus 2008;24:E2. [Crossref] [PubMed]
- Choi JU, Kim DS, Kim EY, Lee KC. Natural history of moyamoya disease: comparison of activity of daily living in surgery and non surgery groups. Clin Neurol Neurosurg 1997;99:S11-8. [Crossref] [PubMed]
- Ma Y, Yang B, Lu X, Gao P, Jiao L, Ling F. Safety and Blood-Flow Outcomes for Hybrid Recanalization in Symptomatic Refractory Long-Segmental Vertebral Artery Occlusion-Results of a Pilot Study. Front Neurol 2020;11:387. [Crossref] [PubMed]
- Ma Y, Li M, Jiao LQ, Zhang HQ, Ling F. Contralateral cerebral hemodynamic changes after unilateral direct revascularization in patients with moyamoya disease. Neurosurg Rev 2011;34:347-53; discussion 353-4. [Crossref] [PubMed]
- Funaki T, Takahashi JC, Takagi Y, Kikuchi T, Yoshida K, Mitsuhara T, Kataoka H, Okada T, Fushimi Y, Miyamoto S. Unstable moyamoya disease: clinical features and impact on perioperative ischemic complications. J Neurosurg 2015;122:400-7. [Crossref] [PubMed]
- Ma Y, Lu X, Zeng G, Yang B, Jiao L, Ling F. Vascular Architecture Characters and Changes of Pediatric Moyamoya Disease after Combined Bypass Surgery. Neuropediatrics 2022;53:188-94. [Crossref] [PubMed]
- Research Committee on the Pathology and Treatment of Spontaneous Occlusion of the Circle of Willis. Guidelines for diagnosis and treatment of moyamoya disease (spontaneous occlusion of the circle of Willis). Neurol Med Chir (Tokyo) 2012;52:245-66.
- Suzuki J, Takaku A. Cerebrovascular "moyamoya" disease. Disease showing abnormal net-like vessels in base of brain. Arch Neurol 1969;20:288-99. [Crossref] [PubMed]
- Morioka M, Hamada J, Kawano T, Todaka T, Yano S, Kai Y, Ushio Y. Angiographic dilatation and branch extension of the anterior choroidal and posterior communicating arteries are predictors of hemorrhage in adult moyamoya patients. Stroke 2003;34:90-5. [Crossref] [PubMed]
- Yamamoto S, Hori S, Kashiwazaki D, Akioka N, Kuwayama N, Kuroda S. Longitudinal anterior-to-posterior shift of collateral channels in patients with moyamoya disease: an implication for its hemorrhagic onset. J Neurosurg 2018;130:884-90. [Crossref] [PubMed]
- Funaki T, Takahashi JC, Houkin K, Kuroda S, Takeuchi S, Fujimura M, Tomata Y, Miyamoto S. Angiographic features of hemorrhagic moyamoya disease with high recurrence risk: a supplementary analysis of the Japan Adult Moyamoya Trial. J Neurosurg 2018;128:777-84. [Crossref] [PubMed]
- Matsushima T, Inoue T, Suzuki SO, Fujii K, Fukui M, Hasuo K. Surgical treatment of moyamoya disease in pediatric patients--comparison between the results of indirect and direct revascularization procedures. Neurosurgery 1992;31:401-5. [Crossref] [PubMed]
- Agarwal V, Singh P, Ahuja CK, Gupta SK, Aggarwal A, Narayanan R. Non-invasive assessment of cerebral microvascular changes for predicting postoperative cerebral hyperperfusion after surgical revascularisation for moyamoya disease: an arterial spin labelling MRI study. Neuroradiology 2021;63:563-72. [Crossref] [PubMed]
- Alsop DC, Detre JA, Golay X, Günther M, Hendrikse J, Hernandez-Garcia L, Lu H, MacIntosh BJ, Parkes LM, Smits M, van Osch MJ, Wang DJ, Wong EC, Zaharchuk G. Recommended implementation of arterial spin-labeled perfusion MRI for clinical applications: A consensus of the ISMRM perfusion study group and the European consortium for ASL in dementia. Magn Reson Med 2015;73:102-16. [Crossref] [PubMed]
- Zaharchuk G, Do HM, Marks MP, Rosenberg J, Moseley ME, Steinberg GK. Arterial spin-labeling MRI can identify the presence and intensity of collateral perfusion in patients with moyamoya disease. Stroke 2011;42:2485-91. [Crossref] [PubMed]
- Cui B, Zhang T, Ma Y, Chen Z, Ma J, Ma L, Jiao L, Zhou Y, Shan B, Lu J. Simultaneous PET-MRI imaging of cerebral blood flow and glucose metabolism in the symptomatic unilateral internal carotid artery/middle cerebral artery steno-occlusive disease. Eur J Nucl Med Mol Imaging 2020;47:1668-77. [Crossref] [PubMed]
- Ge P, Zhang Q, Ye X, Liu X, Deng X, Wang J, Wang R, Zhang Y, Zhang D, Zhao JZ. Digital subtraction angiographic characteristics of progression of moyamoya disease 6 months prior to surgical revascularisation. Stroke Vasc Neurol 2020;5:97-102. [Crossref] [PubMed]
- Lee WJ, Jeong SK, Han KS, Lee SH, Ryu YJ, Sohn CH, Jung KH. Impact of Endothelial Shear Stress on the Bilateral Progression of Unilateral Moyamoya Disease. Stroke 2020;51:775-83. [Crossref] [PubMed]
- Park EK, Lee YH, Shim KW, Choi JU, Kim DS. Natural history and progression factors of unilateral moyamoya disease in pediatric patients. Childs Nerv Syst 2011;27:1281-7. [Crossref] [PubMed]
- Malakar S, Datta AK, Chakraborty U, Chaudhury J, Kumar S, Chandra A, Ray BK. Moyamoya disease: a spectrum of clinical and radiological findings in a series of eight paediatric patients. Acta Neurol Belg 2021;121:1165-72. [Crossref] [PubMed]
- Yeon JY, Shin HJ, Kong DS, Seol HJ, Kim JS, Hong SC, Park K. The prediction of contralateral progression in children and adolescents with unilateral moyamoya disease. Stroke 2011;42:2973-6. [Crossref] [PubMed]
- Kim SJ, Son TO, Kim KH, Jeon P, Hyun SH, Lee KH, Yeon JY, Kim JS, Hong SC, Shin HJ, Bang OY. Neovascularization precedes occlusion in moyamoya disease: angiographic findings in 172 pediatric patients. Eur Neurol 2014;72:299-305. [Crossref] [PubMed]
- Ignarro LJ, Buga GM, Wood KS, Byrns RE, Chaudhuri G. Endothelium-derived relaxing factor produced and released from artery and vein is nitric oxide. Proc Natl Acad Sci U S A 1987;84:9265-9. [Crossref] [PubMed]
- Suzuki Y, Fujita M, Mizutani N, Seki Y, Kimura M, Kajita Y, Takayasu M. Role of nitric oxide in the control of cerebral microcirculation under physiological and pathological conditions. Clin Hemorheol Microcirc 2000;23:307-12.
- Noda A, Suzuki Y, Takayasu M, Watanabe K, Takagi T, Hara M, Yoshida J. Elevation of nitric oxide metabolites in the cerebrospinal fluid of patients with moyamoya disease. Acta Neurochir (Wien) 2000;142:1275-9; discussion 1279-80. [Crossref] [PubMed]
- Mineharu Y, Miyamoto S. RNF213 and GUCY1A3 in Moyamoya Disease: Key Regulators of Metabolism, Inflammation, and Vascular Stability. Front Neurol 2021;12:687088. [Crossref] [PubMed]
- Jeon JS, Ahn JH, Moon YJ, Cho WS, Son YJ, Kim SK, Wang KC, Bang JS, Kang HS, Kim JE, Oh CW. Expression of cellular retinoic acid-binding protein-I (CRABP-I) in the cerebrospinal fluid of adult onset moyamoya disease and its association with clinical presentation and postoperative haemodynamic change. J Neurol Neurosurg Psychiatry 2014;85:726-31. [Crossref] [PubMed]