Effects of somatostatin analogs on uptake of radiolabeled somatostatin analogs on imaging: a systematic review and meta-analysis
Introduction
Neuroendocrine tumors (NETs) are a heterogeneous group of tumors that occur rarely in comparison with other malignant tumors (1). The incidence of NETs only contributes 0.5% of all malignancies (2). NETs originating from neuroendocrine cells disseminate throughout the body, and the most common site is the gastrointestinal tract, followed by the lung and pancreas (3). Somatostatin receptors (SSTRs), as members of the 7 transmembrane segment receptor superfamily, are overexpressed on most NETs and can bind somatostatin with high selectivity and affinity (4,5). Thus, SSTRs have become the therapeutic and diagnostic target in clinical conditions. Due to the low metabolic stability of natural somatostatin, many synthetic analogs such as octreotide, pasireotide, and lanreotide have been developed to improve the stability (6,7).
Iodine-123, Indium-111 (111In), and Technetinum-99m (99mTc)-labeled somatostatin analogs (SSAs) scintigraphy were the initial methods to visualize SSTRs-positive NETs with a detection rate ranging from 50% to 100% (8-11). However, these SSTRs imaging methods present some limitations in detecting liver lesions or small lesions because of the liver physiological uptake and the low resolution of gamma camera (12,13). Gallium-68 (68Ga)-labeled [1,4,7,10-tetraazacyclododecane-N,N',N'',N'''-tetraacetic acid]-conjugated SSAs (68Ga-DOTA-conjugated SSAs) such as 68Ga-[DOTA-D-Phe1-Tyr3-Thr8]-octreotide (68Ga-DOTATATE), 68Ga-[DOTA-D-Phe1-Tyr3]-octreotide (68Ga-DOTATOC), and 68Ga-[DOTA-D-Phe1-1-Nal3]-octreotide) (68Ga-DOTANOC) were subsequently developed and opened a new horizon in imaging NETs. Positron emission tomography/computed tomography (PET/CT) with 68Ga-DOTA-conjugated SSAs provides higher spatial resolution and more functional and anatomic data compared to single photon emission computed tomography (SPECT) and conventional imaging. Meanwhile, it also has better diagnostic sensitivity and specificity than fluorine-18 [18F]-fluorodeoxyglucose (18F-FDG) PET/CT for detecting NETs, resulting in significant management change (14-16). Up to now, 68Ga-DOTA-conjugated SSAs PET/CT has been widely used in primary tumor localization, metastatic disease detection, and response monitoring, as well as predicting the treatment response for NET patients (17,18).
As the first-line therapy for functionally active NETs, SSAs play a prominent role in controlling hormonal symptoms and reducing tumor growth (5,19). Since nonradioactive SSAs treatment and SSTRs imaging involve the same receptors, high-dose SSA treatment prior to imaging could theoretically interfere with the uptake of radiolabeled SSAs by receptor internalization and saturation (20). Both the European Association of Nuclear Medicine (EANM) procedure guideline for 68Ga-DOTA-conjugated peptides PET/CT and the Society of Nuclear Medicine and Molecular Imaging (SNMMI) concept in 68Ga-DOTATATE PET/CT recommend the time interval of 3–4 or 4–6 weeks between long-acting SSAs treatment and 68Ga-DOTA-conjugated peptides PET/CT to avoid possible SSTR blockade (15,21). However, no clear or strong evidence has been provided to confirm the necessity of SSAs withdrawal before PET imaging. In contrast, recent studies investigating the effect of nonradioactive SSAs on uptake of 68Ga-DOTA-conjugate peptides found reduced uptake in normal tissues, stable uptake in tumor sites, and improved tumor-to-background ratio (22-24). Therefore, in this systematic review and meta-analysis, we aimed to assess whether prior SSAs treatment affects the uptake of radiolabeled SSAs in normal organs and tumor lesions for patients with NETs. We present this article in accordance with the PRISMA-DTA reporting checklist (25) (available at https://qims.amegroups.com/article/view/10.21037/qims-23-477/rc).
Methods
Our study was registered on the international prospective register of systematic reviews (PROSPERO) (CRD42022321650).
Search strategy
A literature search of the databases of PubMed, Embase, and Web of Science (WoS) was performed until March 12, 2022. Keywords were based on the following: (“neuroendocrine tumor” OR “NETs”) AND (“somatostatin receptor imaging” OR “somatostatin receptor Scintigraphy” OR “PET” OR “photon emission tomography” OR “SPECT” OR “single photon emission computed tomography”) AND (“somatostatin analogs” OR “SSA” OR “Lanreotide” OR “octreotide” OR “Pasireotide” OR “DOTATATE” OR “DOTATOC” OR “DOTANOC”).
Study selection
Studies investigating SSTR imaging before and after the commencement of SSA therapy were evaluated. The inclusion criteria were as follows: (I) clinical original studies regarding the alteration of uptake in normal organs and tumors between two SSTR images, which were performed prior to and after SSA treatment. (II) Patients had been confirmed with histologically well-differentiated NETs. Studies irrelevant to the topic were excluded, and case reports, conference abstracts, letters, editorial materials, and reviews were also excluded. When data overlapped among studies, the study with the most details was chosen. The included studies were written in English and performed on humans.
Data extraction
Two reviewers independently extracted data from the eligible studies about study characteristics (i.e., first author, publication year, country, study design) and patient characteristics (i.e., patient population, age, clinical setting, SSAs treatment, uptake characteristics, time intervals). Technical details (i.e., imaging modality, ligand, and injection dose) and any data regarding the tracer uptake in normal tissues and tumor lesions before and after SSAs treatment were also collected.
Quality assessment
The quality of the included studies was independently assessed by two reviewers according to the revised Quality Assessment of Diagnostic Accuracy Studies-2 tool (QUADAS-2 revision) (26). The QUADAS-2 revision was used to evaluate the risk of bias for the following criteria: patient selection, first scan, second scan, and flow/timing, whereas applicability concerns were assessed for patient selection, first scan, and second scan (Table S1). Any discrepancies were resolved by discussion with a third reviewer.
Statistical analysis
The maximum standardized uptake value (SUVmax) of normal tissues including liver, spleen, adrenal glands, thyroid, and pituitary gland was extracted and individually pooled using a random effects model. Similarly, the uptake of tumor lesions categorized according to anatomical site (primary sites, liver, lymph nodes, and bone metastases) was also individually analyzed. Further, the measurements were compared between pretreatment and posttreatment scan. Stata version 15.0 (StataCorp., College Station, TX, USA) was used to conduct meta-regression analyses based on a linear mixed model for summarized mean SUVmax with 95% confidence intervals (CIs). The RevMan 5.3 software (Cochrane Collaboration, Copenhagen, Denmark) was used to evaluate the risk of bias. Heterogeneity between the studies was evaluated using the I2 statistic, and the I2 value greater than 50% was indicative of substantial heterogeneity (27).
Results
Literature search
The flow chart showed an overview of the search and selection process (Figure 1). A total of 6,928 records were identified, and 2,701 records were removed as duplication. After reviewing the title and abstract, 4,210 articles were excluded because they were cases, reviews, letters, conference abstracts, basic studies, or studies relevant to disease diagnosis or treatment. A total of 8 articles were excluded after full-text evaluation. Finally, 9 articles were eligible for this systematic review and meta-analysis (22-24,28-33).
Study description
Table 1 summarizes the main characteristics of the included 9 studies. Among them, 6 studies were performed in Europe, 2 studies in Australia, and 1 in China; 3 studies were performed prospectively, whereas 6 studies had a retrospective study design; 7 studies involved intraindividual research, 1 interindividual research, and 1 combined inter-and intra-individual study design. Table 2 shows the imaging modalities of SSTRs among the 9 studies. The uptake of 68Ga-DOTA-SSAs was assessed in 6 studies, especially 68Ga-DOTATATE in 5 studies, whereas the other 3 studies investigated the uptake of 111In or 99mTc labeled SSAs. The injection activity of radiolabeled SSAs was heterogeneous and contained both weighted-based and fixed activities.
Table 1
First author | Year | Design | Comparison | Country | Patients | Age (years) |
Clinical setting | SSA treatment | Uptake parameters | Time interval† | Time interval‡ |
---|---|---|---|---|---|---|---|---|---|---|---|
Jahn (33) | 2021 | P | Intraindividual | Sweden | 4 | 69±5.94 | Small-intestinal NETs who were all progressing on long-acting SSA | Sandostatin LAR: 30 mg (1 patients) every 3 weeks; Sandostatin Autogel: 120 mg (2 patients) every 2 weeks, 120 mg (1 patients) every 4 weeks | SUV, normalized SUV, net uptake rate (Ki) | 1–3.5 m | 10–15 min |
Gålne (24) | 2019 | P | Intraindividual | Sweden | 19 | 65.7±8.2 | Histologically verified NET with ongoing treatment with LA SSA, or evaluation of suspected NET with likely initiation of LA SSA treatment within a year | Lanreotide: 120 mg (9 patients) or 90 mg (2 patients) every 4 weeks | SUVmax (T/L) | 202 d | 14.7±8.8 d |
Octreotide: 30 mg (4 patients) or 20 mg (4 patients) every 4 weeks | |||||||||||
Li (32) | 2019 | R | Interindividual | China | 60 | 51.5 | Patients with G2 NETs graded by 2010 WHO classification | Sandostatin LAR: 30 mg every 4 weeks | Target/muscle ratio (T/M) | NA | 14.7±8.0 d |
Aalbersberg (23) | 2019 | P | Intraindividual | Netherland | 34 | 64.2 [45–78] | Histologically confirmed well-differentiated NET (grade I–II) | Lanreotide: 60, 90, or 120 mg every 3–4 weeks for the individual patients | SUVmax, SUVmean, SUVpeak (T/L) |
2 d | 1 d |
Cherk (31) | 2018 | R | Intraindividual | Australia | 21 | NA | Metastatic NETs | Sandostatin LAR: 30 mg (16 patients), 20 mg (2 patients) or 40 mg (1 patient) every 4 weeks | SUVmax (T/L) | 2–12 m | 3–4 w |
Lanreotide: 90 mg or 120 mg (2 patients) | |||||||||||
Ayati (22) | 2018 | R | Intraindividual | Australia | 30 | 64.6±13.4 | Histologically-proven metastatic intermediately differentiated to well-differentiated NETs | Sandostatin LAR: 30 mg (29 patients) or 60 mg (1 patients) every 4 weeks |
SUVmax, SUVmean | 9.6 m | 25.1±14.8 d |
Haug (30) | 2011 | R | Interindividual + intraindividual | Germany | 105 | 58±12 | Histologically proven well- to intermediately-differentiated NET | Sandostatin LAR: 30 mg (33 patients), 20 mg (1 patients) or 50 mg (1 patient) every 4 weeks | SUVmax, SUVmean | NA | 14.5±11.4 d |
Janson (29) | 1999 | R | Intraindividual | Sweden | 8 | 64 [49–74] | Five patients had malignant carcinoid tumors and three had malignant endocrine pancreatic tumors | Lanreotide: a daily dose of 6,000–12,000 μg four times daily | T/background | 10–13 m | 3 d |
Dörr (28) | 1993 | R | Intraindividual | Germany | 5 | NA | NETs midgut carcinoid with liver metastases and additional abdominal and/or mediastinal lymph node metastases | Octreotide: a daily dosage of 600 μg | T/background | <4 w | <1 d |
Data are presented as mean ± standard deviation or median [interquartile range]. †, time interval between two scans; ‡, time interval between most recent SSA treatment and PET or SPECT scan. P, prospective; R, retrospective; NETs, neuroendocrine tumors; SSA, somatostatin analog; LA, long-acting; WHO, World Health Organization; LAR, long-acting release; SUV, standardized uptake value; SUVmax, maximum standardized uptake value; SUVmean, mean standardized uptake value; SUVpeak, peak standardized uptake value; T/L, tumor/liver; T/M, tumor/muscle; T, tumor; NA, not available; m, month; w, week; d, day; PET, positron emission tomography; SPECT, single photon emission computed tomography.
Table 2
First author | Year | Modality | Ligand | Dose (MBq) |
---|---|---|---|---|
Jahn (33) | 2021 | Discovery MI, GE Healthcare | 68Ga-DOTATOC | 167±21 |
Gålne (24) | 2019 | Discovery 690 scanner (GE Healthcare) | 68Ga-DOTATATE | 2.5 MBq/kg |
Li (32) | 2019 | Discovery NM/CT 670, GE | 99mTc-HYNIC-TOC | 370 |
Aalbersberg (23) | 2019 | Gemini TOF PET/CT (Philips) | 68Ga-DOTATATE | 100 |
Cherk (31) | 2018 | Discovery 690 GE Healthcare or Siemens Biograph Healthcare | 68Ga-DOTATATE | 85–307 |
Ayati (22) | 2018 | Gemini TOF PET/CT scanner (Philips) | 68Ga-DOTATATE | 110–185 |
Haug (30) | 2011 | Gemini PET/CT scanner (Philips) | 68Ga-DOTATATE | 200 |
Janson (29) | 1999 | SPECT (Nuclear Diagnostics, Hagersten, Sweden and London, UK) | 111In-pentetreotide | 144 [114–238] |
Dörr (28) | 1993 | Siemens Orbiter 7500 Gamma Camera | 111In-pentetreotide | 137 [105–237] |
Data are presented as mean ± standard deviation, median [interquartile range] or n. PET/CT, positron emission tomography/computed tomography; SPECT, single photon emission computed tomography; MI, molecular imaging; NM, nuclear medicine; TOF, time of flight; 68Ga, Gallium-68; 99mTc, Technetinum-99m; 111In, Indium-111.
Quality assessment
Following the revised QUADAS-2 tool, we assessed the quality of included studies (Figure 2). For interindividual design studies, SSTRs imaging of the SSAs untreated group was regarded as the first scan while that of the SSAs treated group as the second scan. For intraindividual studies, pretreatment and posttreatment SSTRs imaging were defined as first and second scan, respectively. Almost all studies had a low risk of bias in patient selection. Only 1 study had an unclear risk of bias in patient selection and applicability concerns because 3 patients had undergone the first scan after a 24-hour period of octreotide withdrawal (28). Two studies showed unclear risk in the applicability concerns of patient selection because of the interindividual comparison design (30,32). Since the first and second scan results were measured independently, we graded the risk of bias and applicability concerns in both scans as low risk. Regarding the flow and timing, some studies had unclear risk because the time intervals between 2 scans were heterogeneous or they were interindividual studies (22,29,30,32,33).
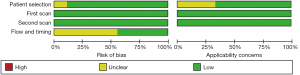
Main findings of included studies
Table 3 provides an overview of the effect of SSAs treatment on radiolabeled SSAs uptake. The radiolabeled SSAs uptake decreased in the liver in patients after SSAs treatment in all studies (22-24,28,30-33). The same trend was also observed in the spleen in almost all studies, whereas only 1 study showed that the spleen-to-muscle ratio of 99mTc-hydrazinonicotinyl-Tyr3-octreotide (99mTc-HYNIC-TOC) did not significantly change following SSAs therapy (22,23,28,30,31,33). No convincing data revealed that SSAs treatment significantly changed the uptake of radiolabeled SSAs in the adrenal gland, kidney, pituitary gland, bone, and parotid gland. A total of 3 studies suggested that radiolabeled SSAs uptake might be decreased in the thyroid after SSAs treatment (22,23,31). Meanwhile, 8 studies evaluated the effect of SSAs treatment on tumor uptake of radiolabeled tracer, and among them, 3 showed increased tumor uptake after SSAs treatment whereas the remaining did not show any significant effect (22-24,28,30-33). In 5 studies, the tumor-to-liver/background ratio increased significantly after SSAs treatment (23,24,28,29,31).
Table 3
Author [year] | Liver | Spleen | Kidney | Adrenal gland | Thyroid | Pituitary gland | Parotid gland | Bone | Tumor | Tumor-to-liver/background ratio |
---|---|---|---|---|---|---|---|---|---|---|
Jahn [2021]† (33) | ↓ | ↓ | NS | – | – | – | – | NS | NS | – |
Gålne [2019] (24) | ↓ | – | – | – | – | – | – | – | NS | The tumor-to-liver ratio was higher after treatment initiation with LA SSA and the tumor lesions in the liver were better visualized |
Li [2019]‡ (32) | ↓ | NS | NS | NS | – | – | – | ↓ | NS | – |
Aalbersberg [2019] (23) | ↓ | ↓ | NS | NS | ↓ | NS | NS | NS | ↑ | The tumor-to-liver ratio for SUVmax increased after lanreotide injection in all lesions |
Cherk [2018] (31) | ↓ | ↓ | – | NS | ↓ | ↑ | NS | – | ↑§ | Metastatic lesion uptake and lesion-to-liver SUVmax ratio increased in 82% of lesions following SSA therapy |
Ayati [2018] (22) | ↓ | ↓ | – | NS | ↓ | NS | – | – | NS | – |
Haug [2011] (30) | ↓ | ↓ | NS | NS | – | NS | – | – | NS | – |
Janson [1999] (29) | – | – | – | – | – | – | – | – | – | The tumor-to-background ratio had an average increase in the ratio of 50%, while the spleen-to-background ratio decreased significantly (the average reduction ratio was 55%) |
Dörr [1993] (28) | ↓ | ↓ | ↓ | – | – | – | – | – | ↑ | The tumor-to-liver ratio improved markedly |
†, the time interval was 7 hours; ‡, the individual measurement of tracer uptake was target-to-muscle ratio; §, 61% of metastatic lesions had an increase in SUVmax following SSA therapy. ↓, decrease; ↑, increase; –, not available; SSAs, somatostatin analogs; NS, no significance; SUVmax, maximum standardized uptake value.
Meta-analysis of SUVmax in normal organs and tumors
A total of 6 studies investigated the effect of SSAs on the uptake of the 68Ga-labeled SSAs. Among these, 5 studies presented detailed data about the uptake of 68Ga-DOTATATE in healthy organs and tumors. We further extracted and pooled these data and performed meta-analysis. Figure 3 displays the pooled SUVmax prior to and after SSAs treatment in the liver (Figure 3A) and spleen (Figure 3B). The SUVmax of the liver prior to SSAs treatment was 9.56 (95% CI: 9.17–9.95, I2=85.9%) and decreased to 7.62 (95% CI: 7.24–8.00, I2=93.3%) following SSAs therapy. The corresponding parameters were 25.74 (95% CI: 24.61–26.88, I2=77.9%) and 20.39 (95% CI: 19.29–21.49, I2=80.3%) for spleen. As shown in Figure S1 and Figure S2, the summarized SUVmax of the adrenal gland, thyroid, and pituitary gland before SSAs treatment were 18.63 (95% CI: 17.68–19.58), 4.52 (95% CI: 4.10–4.95), and 3.99 (95% CI: 3.66–4.31), respectively. After SSAs treatment, the summarized parameters of these normal tissues were 17.81 (95% CI: 16.62–19.01), 3.03 (95% CI: 2.65–3.40), and 5.58 (95% CI: 4.83–6.33), respectively. SUVmax significantly decreased in the liver (P=0.001) and spleen (P=0.006) following SSAs treatment whereas no significant change was noted in the adrenal gland (P=0.83), thyroid (P=0.07), and pituitary gland (P=0.33) (Figure 4A). With regard to tumor uptake, pooled parameters before and after SSAs treatment are demonstrated in Figure S3 and Figure S4. The uptake of the hottest lesion did not differ significantly between pre-and post-treatment scans (28.14±14.3 vs. 29.28±14.51, P=0.37). In addition, neither primary tumor sites nor metastases showed significant differences after SSAs treatment (Figure 4B).
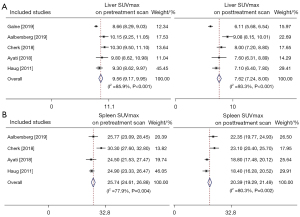
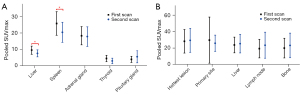
Discussion
This meta-analysis summarizes the effect of SSAs therapy prior to imaging on the uptake of radiolabeled SSAs for NETs patients. We detected significantly decreased tracer uptake in the liver and spleen after SSAs treatment, especially uptake of 68Ga-labeled SSAs. Conversely, no significant change was observed in primary tumor sites or metastatic lesions.
SSAs, as synthetic SSAs targeting SSTRs, have been widely applied in NETs imaging and therapy (34). The rationale is the tumor cell receptor-mediated internalization of the radio- or non-radiolabeled SSAs and their retention in the cytoplasm (21). Theoretically, treatment with nonradioactive SSAs could result in possible SSTRs occupancy and blockade, and then interfere with the interpretation of radiolabeled SSAs imaging. Velikyan et al. found that different doses of octreotide (0, 50, 250, and 500 µg) administered immediately before 68Ga-DOTATOC PET/CT imaging affected the 68Ga-DOTATOC uptake, revealing a blocking or saturation effect at higher amounts of SSA (35). Thus, current SSTRs imaging guidelines recommended the discontinuation of SSAs therapy prior to imaging to avoid possible decreased radioactive SSAs uptake (15). However, most of our included studies reported inconsistent results, of which 3 studies showed a significant increase in tumor lesions after SSAs treatment, whereas in 5 studies, the difference of tumor uptake was not significant. Taken together, our pooled results indicate that SUVmax of primary tumors or metastases did not change significantly after SSAs treatment. The plausible explanation may be the fast SSTRs recovery in NETs within a short time frame, compared to that in normal organs (36,37). Although our findings may represent the worst-case scenario, they may indicate that nonradioactive SSAs treatment has little effect on the tumor uptake of radiolabeled SSAs on SSTRs imaging.
In contrast to the change of tumor uptake after SSAs, our results showed that the SUVmax of 68Ga-labeled tracer in the liver and spleen were significantly decreased on the posttreatment scan with an approximately 20% reduction, whereas no significant difference was observed in other normal tissues including the adrenal, thyroid, and pituitary glands. These findings are almost in agreement with previously reported relevant studies. In a prospective study with intraindividual design, Aalbersberg et al. reported significantly decreased SUVmax in the liver (10.15 vs. 9.08, P<0.001) and spleen (25.77 vs. 22.35, P<0.001) after SSAs, with a reduction of about 10% (23). A recent study showed a higher reduction of 25% and 20% in physiologic spleen and liver accumulation, respectively, similar to our findings (23). It should be noted that following SSAs therapy, the thyroid uptake also decreased although the difference did not reach statistical significance due to the small sample size. Some studies also investigated the uptake difference in the kidney, parotid gland, and bone between 2 PET/CT scans, but these data were too scarce to be pooled in this meta-analysis. The different tendency between tumor and liver or spleen uptake may be due to different SSTRs recycling kinetics mainly consisting of receptor internalization and expression after SSAs therapy (37). Interestingly, a recent study that investigated the time-dependent extended effect of SSAs on the tumor versus normal tissue uptake of 68Ga-DOTATOC found that tumor SUV decreased significantly from baseline to 1 hour post-injection but subsequently increased to baseline level at 4 hours whereas the uptake in the liver and spleen remained significantly below baseline level at 7 hours, suggesting faster SSTRs recycling in tumors than in normal tissues (33). In addition, the difference in inherent receptor density could also lead to the different thresholds for receptor saturation in normal tissues and tumors (38,39).
Given that there was no evidence of decreased tumor uptake but significant reduction in the liver or spleen uptake, several included studies consistently demonstrated the improved tumor-to-liver or background ratio after SSAs treatment. In spite of the prominent heterogeneity of NETs, this finding may be generalized to all types of this tumor. Aalbersberg et al. reported increased tumor-to-liver ratio for SUVmax in all lesions after SSA, including abdominal, liver, lymph node, and bone lesions (23). The increased ratio not only facilitates tumor detection but also provides obvious implications for peptide receptor radionuclide treatment (PRRT). Firstly, the improved tumor-to-background or liver ratio perhaps increases the likelihood of being suitable for PRRT. Then, nonradioactive SSAs pretreatment may decrease the uptake of 177Lu- or 90Y-SSAs in normal tissues, especially in the spleen, thus reducing potential radiation exposure and adverse side effects on normal tissues (40). However, the amount of peptide administered during PRRT is much higher than that for 68Ga-SSAs PET/CT, thus further research is needed to confirm our findings in PRRT (39). Apart from these, the change in tumor-to-liver or -spleen ratio was also used as a valid marker for evaluating the disease status in some studies (41-43). Clinicians should be aware of the effect of SSAs on the tumor-to-background ratio on SSTRs imaging, in case of misdiagnosis as disease progression during response assessment.
Consistent with previous studies, our systematic review and meta-analysis that supports no withdrawal of SSAs treatment prior to SSTRs imaging might affect the procedure guideline for 68Ga-DOTA-SSAs PET/CT. If continuation of SSAs treatment prior to SSTRs imaging is undertaken, it is of great benefit to patients by controlling the symptoms and reducing the risk of tumor growth. There is also no need for patients to use short-acting SSAs instead that has to be administrated 3 times daily for preparation of SSTRs imaging. Meanwhile, the nuclear medicine department can have flexible schedules for SSTRs imaging without having to adhere to the SSAs administration time.
Our study has some limitations. Firstly, the number of included articles was relatively small, especially for pooled analysis, which might be a possible source of bias. Secondly, prominent heterogeneity of the study design was found among included studies, such as SSAs treatment, time intervals between 2 scans, and from the last injection to posttreatment scan, which significantly affected the reliability of pooled results. In addition, the study quality including patient selection, various radiolabeled SSAs, and diverse imaging outcomes also made contributions to the high heterogeneity. Thirdly, the included studies used different radiolabeled SSAs tracers, scanners, and scanning methods, thus the imaging interpretation and parameter measurement may be inconsistent among studies.
Conclusions
SSAs therapy prior to imaging resulted in a significant reduction in the liver and spleen uptake, but did not decrease the uptake of radiolabeled SSAs in tumor primary or metastatic sites as well as other normal tissues. These findings have significant implications for procedure guidelines of SSTRs imaging and support the unnecessary discontinuation of SSAs prior to radiolabeled SSAs imaging (Table 4). Further prospective, multicenter, and long-term prospective longitudinal studies with a large sample are also needed to better determine the effect of SSAs therapy on the uptake of radiolabeled SSAs in normal organs and tumor lesions.
Table 4
Items | Guideline | Our findings |
---|---|---|
SSAs preparation before the scan | Withdrawal for 3–4 weeks or 4–6 weeks† | No withdrawal† |
1–2 days‡ | Withdrawal for several hours (>4 hours)‡ | |
Implications for PET interpretation | No decreased uptake in tumor sites whereas decreased uptake in healthy organs (e.g., liver, spleen) | |
Cautious about using tumor-to-liver/spleen ratio for response assessment |
†, long-acting SSAs; ‡, short-acting SSAs. PET, positron emission tomography; SSAs, somatostatin analogs.
Acknowledgments
Funding: This work was supported by
Footnote
Reporting Checklist: The authors have completed the PRISMA-DTA reporting checklist. Available at https://qims.amegroups.com/article/view/10.21037/qims-23-477/rc
Conflicts of Interest: All authors have completed the ICMJE uniform disclosure form (available at https://qims.amegroups.com/article/view/10.21037/qims-23-477/coif). The authors report that this work was supported by the National Natural Science Foundation of China (No. 81901776) and the Post-Doctor Research Project, West China Hospital, Sichuan University (No. 2023HXBH075).
Ethical Statement: The authors are accountable for all aspects of the work in ensuring that questions related to the accuracy or integrity of any part of the work are appropriately investigated and resolved.
Open Access Statement: This is an Open Access article distributed in accordance with the Creative Commons Attribution-NonCommercial-NoDerivs 4.0 International License (CC BY-NC-ND 4.0), which permits the non-commercial replication and distribution of the article with the strict proviso that no changes or edits are made and the original work is properly cited (including links to both the formal publication through the relevant DOI and the license). See: https://creativecommons.org/licenses/by-nc-nd/4.0/.
References
- Rindi G, Klimstra DS, Abedi-Ardekani B, Asa SL, Bosman FT, Brambilla E, et al. A common classification framework for neuroendocrine neoplasms: an International Agency for Research on Cancer (IARC) and World Health Organization (WHO) expert consensus proposal. Mod Pathol 2018;31:1770-86.
- Rust E, Hubele F, Marzano E, Goichot B, Pessaux P, Kurtz JE, Imperiale A. Nuclear medicine imaging of gastro-entero-pancreatic neuroendocrine tumors. The key role of cellular differentiation and tumor grade: from theory to clinical practice. Cancer Imaging 2012;12:173-84. [Crossref] [PubMed]
- Dasari A, Shen C, Halperin D, Zhao B, Zhou S, Xu Y, Shih T, Yao JC. Trends in the Incidence, Prevalence, and Survival Outcomes in Patients With Neuroendocrine Tumors in the United States. JAMA Oncol 2017;3:1335-42. [Crossref] [PubMed]
- Reisine T, Bell GI. Molecular biology of somatostatin receptors. Endocr Rev 1995;16:427-42. [Crossref] [PubMed]
- De Martino MC, Hofland LJ, Lamberts SW. Somatostatin and somatostatin receptors: from basic concepts to clinical applications. Prog Brain Res 2010;182:255-80. [Crossref] [PubMed]
- Bombardieri E, Maccauro M, De Deckere E, Savelli G, Chiti A. Nuclear medicine imaging of neuroendocrine tumours. Ann Oncol 2001;12:S51-61. [Crossref] [PubMed]
- Reubi JC, Waser B. Concomitant expression of several peptide receptors in neuroendocrine tumours: molecular basis for in vivo multireceptor tumour targeting. Eur J Nucl Med Mol Imaging 2003;30:781-93. [Crossref] [PubMed]
- Chiti A, Fanti S, Savelli G, Romeo A, Bellanova B, Rodari M, van Graafeiland BJ, Monetti N, Bombardieri E. Comparison of somatostatin receptor imaging, computed tomography and ultrasound in the clinical management of neuroendocrine gastro-entero-pancreatic tumours. Eur J Nucl Med 1998;25:1396-403. [Crossref] [PubMed]
- Jamar F, Fiasse R, Leners N, Pauwels S. Somatostatin receptor imaging with indium-111-pentetreotide in gastroenteropancreatic neuroendocrine tumors: safety, efficacy and impact on patient management. J Nucl Med 1995;36:542-9.
- Krenning EP, Kwekkeboom DJ, Bakker WH, Breeman WA, Kooij PP, Oei HY, van Hagen M, Postema PT, de Jong M, Reubi JC, et al. Somatostatin receptor scintigraphy with [111In-DTPA-D-Phe1]- and [123I-Tyr3]-octreotide: the Rotterdam experience with more than 1000 patients. Eur J Nucl Med 1993;20:716-31. [Crossref] [PubMed]
- Olsen JO, Pozderac RV, Hinkle G, Hill T, O'Dorisio TM, Schirmer WJ, Ellison EC, O'Dorisio MS. Somatostatin receptor imaging of neuroendocrine tumors with indium-111 pentetreotide (Octreoscan). Semin Nucl Med 1995;25:251-61. [Crossref] [PubMed]
- Buchmann I, Henze M, Engelbrecht S, Eisenhut M, Runz A, Schäfer M, Schilling T, Haufe S, Herrmann T, Haberkorn U. Comparison of 68Ga-DOTATOC PET and 111In-DTPAOC (Octreoscan) SPECT in patients with neuroendocrine tumours. Eur J Nucl Med Mol Imaging 2007;34:1617-26. [Crossref] [PubMed]
- Hofmann M, Maecke H, Börner R, Weckesser E, Schöffski P, Oei L, Schumacher J, Henze M, Heppeler A, Meyer J, Knapp H. Biokinetics and imaging with the somatostatin receptor PET radioligand (68)Ga-DOTATOC: preliminary data. Eur J Nucl Med 2001;28:1751-7. [Crossref] [PubMed]
- Barrio M, Czernin J, Fanti S, Ambrosini V, Binse I, Du L, Eiber M, Herrmann K, Fendler WP. The Impact of Somatostatin Receptor-Directed PET/CT on the Management of Patients with Neuroendocrine Tumor: A Systematic Review and Meta-Analysis. J Nucl Med 2017;58:756-61. [Crossref] [PubMed]
- Bozkurt MF, Virgolini I, Balogova S, Beheshti M, Rubello D, Decristoforo C, Ambrosini V, Kjaer A, Delgado-Bolton R, Kunikowska J, Oyen WJG, Chiti A, Giammarile F, Sundin A, Fanti S. Guideline for PET/CT imaging of neuroendocrine neoplasms with (68)Ga-DOTA-conjugated somatostatin receptor targeting peptides and (18)F-DOPA. Eur J Nucl Med Mol Imaging 2017;44:1588-601.
- Refardt J, Hofland J, Wild D, Christ E. Molecular Imaging of Neuroendocrine Neoplasms. J Clin Endocrinol Metab 2022;107:e2662-70. [Crossref] [PubMed]
- Pruthi A, Pankaj P, Verma R, Jain A, Belho ES, Mahajan H. Ga-68 DOTANOC PET/CT imaging in detection of primary site in patients with metastatic neuroendocrine tumours of unknown origin and its impact on clinical decision making: experience from a tertiary care centre in India. J Gastrointest Oncol 2016;7:449-61. [Crossref] [PubMed]
- Velikyan I, Sundin A, Sörensen J, Lubberink M, Sandström M, Garske-Román U, Lundqvist H, Granberg D, Eriksson B. Quantitative and qualitative intrapatient comparison of 68Ga-DOTATOC and 68Ga-DOTATATE: net uptake rate for accurate quantification. J Nucl Med 2014;55:204-10. [Crossref] [PubMed]
- Scodeller P, Simón-Gracia L, Kopanchuk S, Tobi A, Kilk K, Säälik P, Kurm K, Squadrito ML, Kotamraju VR, Rinken A, De Palma M, Ruoslahti E, Teesalu T. Precision Targeting of Tumor Macrophages with a CD206 Binding Peptide. Sci Rep 2017;7:14655. [Crossref] [PubMed]
- Virgolini I, Ambrosini V, Bomanji JB, Baum RP, Fanti S, Gabriel M, Papathanasiou ND, Pepe G, Oyen W, De Cristoforo C, Chiti A. Procedure guidelines for PET/CT tumour imaging with 68Ga-DOTA-conjugated peptides: 68Ga-DOTA-TOC, 68Ga-DOTA-NOC, 68Ga-DOTA-TATE. Eur J Nucl Med Mol Imaging 2010;37:2004-10. [Crossref] [PubMed]
- Bodei L, Ambrosini V, Herrmann K, Modlin I. Current Concepts in (68)Ga-DOTATATE Imaging of Neuroendocrine Neoplasms: Interpretation, Biodistribution, Dosimetry, and Molecular Strategies. J Nucl Med 2017;58:1718-26. [Crossref] [PubMed]
- Ayati N, Lee ST, Zakavi R, Pathmaraj K, Al-Qatawna L, Poon A, Scott AM. Long-Acting Somatostatin Analog Therapy Differentially Alters (68)Ga-DOTATATE Uptake in Normal Tissues Compared with Primary Tumors and Metastatic Lesions. J Nucl Med 2018;59:223-7. [Crossref] [PubMed]
- Aalbersberg EA, de Wit-van der Veen BJ, Versleijen MWJ, Saveur LJ, Valk GD, Tesselaar MET, Stokkel MPM. Influence of lanreotide on uptake of (68)Ga-DOTATATE in patients with neuroendocrine tumours: a prospective intra-patient evaluation. Eur J Nucl Med Mol Imaging 2019;46:696-703. [Crossref] [PubMed]
- Gålne A, Almquist H, Almquist M, Hindorf C, Ohlsson T, Nordenström E, Sundlöv A, Trägårdh E. A Prospective Observational Study to Evaluate the Effects of Long-Acting Somatostatin Analogs on (68)Ga-DOTATATE Uptake in Patients with Neuroendocrine Tumors. J Nucl Med 2019;60:1717-23. [Crossref] [PubMed]
- Liberati A, Altman DG, Tetzlaff J, Mulrow C, Gøtzsche PC, Ioannidis JP, Clarke M, Devereaux PJ, Kleijnen J, Moher D. The PRISMA statement for reporting systematic reviews and meta-analyses of studies that evaluate health care interventions: explanation and elaboration. J Clin Epidemiol 2009;62:e1-34. [Crossref] [PubMed]
- Whiting PF, Rutjes AW, Westwood ME, Mallett S, Deeks JJ, Reitsma JB, Leeflang MM, Sterne JA, Bossuyt PM. QUADAS-2 Group. QUADAS-2: a revised tool for the quality assessment of diagnostic accuracy studies. Ann Intern Med 2011;155:529-36. [Crossref] [PubMed]
- Higgins JP, Thompson SG. Quantifying heterogeneity in a meta-analysis. Stat Med 2002;21:1539-58. [Crossref] [PubMed]
- Dörr U, Räth U, Sautter-Bihl ML, Guzman G, Bach D, Adrian HJ, Bihl H. Improved visualization of carcinoid liver metastases by indium-111 pentetreotide scintigraphy following treatment with cold somatostatin analogue. Eur J Nucl Med 1993;20:431-3. [Crossref] [PubMed]
- Janson ET, Kälkner KM, Eriksson B, Westlin JE, Oberg K. Somatostatin receptor scintigraphy during treatment with lanreotide in patients with neuroendocrine tumors. Nucl Med Biol 1999;26:877-82. [Crossref] [PubMed]
- Haug AR, Rominger A, Mustafa M, Auernhammer C, Göke B, Schmidt GP, Wängler B, Cumming P, Bartenstein P, Hacker M. Treatment with octreotide does not reduce tumor uptake of (68)Ga-DOTATATE as measured by PET/CT in patients with neuroendocrine tumors. J Nucl Med 2011;52:1679-83. [Crossref] [PubMed]
- Cherk MH, Kong G, Hicks RJ, Hofman MS. Changes in biodistribution on (68)Ga-DOTA-Octreotate PET/CT after long acting somatostatin analogue therapy in neuroendocrine tumour patients may result in pseudoprogression. Cancer Imaging 2018;18:3. [Crossref] [PubMed]
- Li Y, Xu J, Xu X, Zhang J, Zhang Y. Long-acting octreotide treatment has no impact on tumor uptake of 99mTc-HYNIC-TOC in patients with neuroendocrine tumors. Nucl Med Commun 2019;40:1005-10. [Crossref] [PubMed]
- Jahn U, Ilan E, Velikyan I, Fröss-Baron K, Lubberink M, Sundin A. Receptor depletion and recovery in small-intestinal neuroendocrine tumors and normal tissues after administration of a single intravenous dose of octreotide measured by (68)Ga-DOTATOC PET/CT. EJNMMI Res 2021;11:118. [Crossref] [PubMed]
- Eychenne R, Bouvry C, Bourgeois M, Loyer P, Benoist E, Lepareur N. Overview of Radiolabeled Somatostatin Analogs for Cancer Imaging and Therapy. Molecules 2020;25:4012. [Crossref] [PubMed]
- Velikyan I, Sundin A, Eriksson B, Lundqvist H, Sörensen J, Bergström M, Långström B. In vivo binding of [68Ga]-DOTATOC to somatostatin receptors in neuroendocrine tumours--impact of peptide mass. Nucl Med Biol 2010;37:265-75. [Crossref] [PubMed]
- Waser B, Tamma ML, Cescato R, Maecke HR, Reubi JC. Highly efficient in vivo agonist-induced internalization of sst2 receptors in somatostatin target tissues. J Nucl Med 2009;50:936-41. [Crossref] [PubMed]
- Reubi JC, Waser B, Cescato R, Gloor B, Stettler C, Christ E. Internalized somatostatin receptor subtype 2 in neuroendocrine tumors of octreotide-treated patients. J Clin Endocrinol Metab 2010;95:2343-50. [Crossref] [PubMed]
- Kletting P, Kull T, Maaß C, Malik N, Luster M, Beer AJ, Glatting G. Optimized Peptide Amount and Activity for 90Y-Labeled DOTATATE Therapy. J Nucl Med 2016;57:503-8. [Crossref] [PubMed]
- Sabet A, Nagarajah J, Dogan AS, Biersack HJ, Sabet A, Guhlke S, Ezziddin S. Does PRRT with standard activities of 177Lu-octreotate really achieve relevant somatostatin receptor saturation in target tumor lesions?: insights from intra-therapeutic receptor imaging in patients with metastatic gastroenteropancreatic neuroendocrine tumors. EJNMMI Res 2013;3:82. [Crossref] [PubMed]
- Xu C, Zhang H. Somatostatin receptor based imaging and radionuclide therapy. Biomed Res Int 2015;2015:917968. [Crossref] [PubMed]
- Sharma R, Wang WM, Yusuf S, Evans J, Ramaswami R, Wernig F, Frilling A, Mauri F, Al-Nahhas A, Aboagye EO, Barwick TD. (68)Ga-DOTATATE PET/CT parameters predict response to peptide receptor radionuclide therapy in neuroendocrine tumours. Radiother Oncol 2019;141:108-15. [Crossref] [PubMed]
- Menon BK, Kalshetty A, Bhattacharjee A, Basu S. Standardized uptake values and ratios on 68Ga-DOTATATE PET-computed tomography for normal organs and malignant lesions and their correlation with Krenning score in patients with metastatic neuroendocrine tumors. Nucl Med Commun 2020;41:1095-9. [Crossref] [PubMed]
- Ortega C, Wong RKS, Schaefferkoetter J, Veit-Haibach P, Myrehaug S, Juergens R, Laidley D, Anconina R, Liu A, Metser U. Quantitative (68)Ga-DOTATATE PET/CT Parameters for the Prediction of Therapy Response in Patients with Progressive Metastatic Neuroendocrine Tumors Treated with (177)Lu-DOTATATE. J Nucl Med 2021;62:1406-14. [Crossref] [PubMed]