Monitoring early responses to neoadjuvant chemotherapy and the factors affecting neoadjuvant chemotherapy responses in primary osteosarcoma
Introduction
Osteosarcoma is the most common primary malignant bone tumor in adolescents (1-3). Since surgery began to be combined with neoadjuvant chemotherapy (NACT) and postoperative chemotherapy, the 5-year survival rate of patients with osteosarcoma has improved and reached 60–80% (4). The histological necrosis rate of osteosarcoma is the gold standard for assessing the efficacy of NACT (5). Only patients with a good response to NACT are treated with local resection and limb salvage. However, the histological necrosis rate can only be assessed after surgery. The early noninvasive monitoring and assessment of the treatment response of tumors in the course of chemotherapy is crucial, as it may help to develop patient-specific therapeutic options and thus improve patients’ overall therapeutic outcomes.
In current clinical practice, a number of methods, including clinical symptom relief, alkaline phosphatase (ALP) level decline, and the Response Evaluation Criteria in Solid Tumors (RECIST 1.1), which are based on magnetic resonance imaging (MRI) or computer tomography (CT) measurements, are used to evaluate the efficacy of preoperative NACT. However, there is still no single clinical symptom or laboratory parameters that provides evidence of a good or poor response. Additionally, the therapeutic response may be underestimated using RECIST 1.1 (6).
In recent years, many studies have reported that conventional imaging, including CT, MRI, and functional imaging, such as dynamic contrast enhancement MR, 18F-fluorodeoxyglucose positron emission tomography, and diffusion-weighted imaging (DWI) (6-11), may be used to assess the therapeutic efficacies of NACT. DWI is currently the only imaging method that can noninvasively measure the local diffusion characteristics of water molecules in vivo. DWI reflects the information of spatial composition and the functional status of water exchange among various tissues in pathophysiological states at the molecular level. The diffusion of water molecules within tissues is impeded by cellular membranes, intracellular organelles, macromolecules, and extracellular space. Hypercellular tumor areas restrict the free mobility of water and exhibit low apparent diffusion coefficients (ADCs), while necrotic areas secondary to chemotherapy-induced cellular lysis exhibit higher ADCs through the mechanism of action of cytotoxic chemotherapeutic drugs. In theory, ADCs could be used to distinguish between tumor and necrotic tissues.
Recent studies have shown that the ADCs after the end of whole cycles can indicate the efficacy of NACT (9). The number of cycles of NACT needed varies from patient to patient, but most patients require 3 cycles. Each cycle of chemotherapy lasts for 1 week and is followed by a resting cycle of 2 weeks. Accurately assessing the efficacy of treatment early would be highly valuable in clinical practice. We thus studied the factors affecting the tumor necrosis rate (TNR) after NACT. To date, no research has yet been conducted on this issue.
This study sought to investigate whether the ADC or its change rate after the first cycle of chemotherapy could be used to monitor the effect of NACT on osteosarcoma and whether any factors significantly correlate with the TNR following NACT based on the Huvos grading system. We present the following article in accordance with the STARD reporting checklist (available at https://qims.amegroups.com/article/view/10.21037/qims-22-1095/rc).
Methods
Patient selection
This study was conducted in accordance with the Declaration of Helsinki (as revised in 2013) and was approved by and registered with the Ethics Committee of The Third Hospital of Hebei Medical University. All patients included in this study signed informed consent before undergoing MRI examination.
This study examined patients with osteosarcoma who underwent NACT. In total, 48 patients with conventional osteosarcoma (COS) were recruited at our hospital from June 2017 to November 2019, of whom 31 were male and 17 were female. To be eligible for inclusion in this study, the patients had to meet the following inclusion criteria: (I) have osteosarcoma diagnosed histologically by biopsy and have undergone no radiotherapy, chemotherapy, or surgical treatment before the MRI scan; and (II) have undergone NACT before the surgical procedure. Patients were excluded from the study if they met any of the following exclusion criteria: (I) did not complete NACT and surgery and/or (II) had poor DWI images or did not complete all the cycles of MRI. Among the patients, 7 were excluded from the study because they did not undergo surgery after chemotherapy or had severe MRI artifacts (Figure 1). All the patients underwent the same NACT regimen. The chemotherapy regimen was a combination of (I) cisplatin at a dose of 20 mg/m2 × 5 d and pirarubicin at a dose of 20–30 mg/m2 × 3 d, (II) ifosfamide at a dose of 2 g/m2 × 5 d, and (III) methotrexate at a dose of 8–12 g/m2 × 1 d. All the patients received 3 cycles of chemotherapy at intervals of 2–3 weeks.
MRI protocols
All the patients were examined using a 3.0 T superconducting MRI scanner (MAGNETOM Verio, Siemens Healthineers, Erlangen, Germany). According to the different anatomy sites of the tumor, the patient was placed in the supine position with the head advanced or in the supine position with the foot advanced. Proper surface coils, such as a 8-channel knee coil or a 8-channel body coil, were selected according to the anatomy site. Conventional MRI sequences were used and DW images acquired. The imaging protocol included T1-weighted spin echo sequences (repetition time ms/echo time ms: 700/12); proton density (PD)-weighted fast spin echo sequences (4000/83) in the axial, coronal, and sagittal planes; and DW images acquired in the transverse plane using a spin echo echo-planar sequence with fat-suppression (5800/100; field of view 210 × 210 mm; image matrix 192×192; section/internal =3/0.75 mm; voxel size 1.1 mm × 1.1 mm ×3 mm with b values 0, 500, and 1,000, respectively). The monoexponential ADC map was generated using system software (the Siemens MRSC40794). The thickness and interlayer spacing was 5 mm and 1 mm, respectively, at the above cross-axis scanning sequence.
MRI examinations were performed 3 times as follows: the first MRI examination (MR1) before NACT was performed as a baseline, the second MRI examination (MR2) was performed after the first cycle of NACT (i.e., 5 days after the first phase of chemotherapy), and the third MR examination (MR3) was performed after the end of NACT (i.e., preoperatively).
MRI analysis
The quantitative measurement value on the ADC map was obtained using the Siemens console. Next, the ADC map was sketched manually by referring to the same layer of the proton density-weighted imaging with fat saturation (PDWI-FS) images. Two observers (HY and MJK with 10 and 3 years of experience in MRI, respectively) who were blinded to the clinical and histological information of the patients identified the tumors on the standard MRI and DW images by consensus. The prechemotherapy and postchemotherapy regions of interest (ROIs) that encircled the whole tumor section on the largest axial plane on the PDWI-FS images were manually drawn and then automatically copied on to the ADC map; any distortion artifacts and macroscopically visible necrotic, cystic, or hemorrhagic areas were avoided. The ADCs were measured on the ADC map in units of 10−3 mm2/s (Figure 2A-2C). Observer 1 repeated the measurements with the same rules after a 2-month interval. The ADCs before NACT and after the first, and third cycles of NACT were recorded as ADC1, ADC2, and ADC3, respectively. We defined the (ADC) change values as follows: ADC2-1 = ADC2 − ADC1; ADC3-1 = ADC3 − ADC1; and ADC3-2 = ADC3 − ADC2.
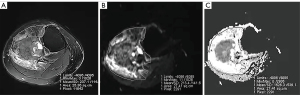
Histological TNR
Two experienced pathologists who were blinded to the clinical and MRI results evaluated all the specimens after the surgical resection of the tumor according to the Huvos grading system (12). In evaluating the TNR, the gross specimen of the tumor included the upper and lower medullary cavity, residual cortical bone, and surrounding soft tissue. The patients with a TNR ≥90% were defined as good responders, and those with a TNR <90% were defined as poor responders.
Statistical analysis
SPSS 21.0 software (IBM Corp., Armonk, NY, USA) was used to perform the statistical analyses. The interclass correlation coefficient (ICC) was used to test the repeatability of the ADC value measurements. The Shapiro-Wilk test was used to detect the normal distribution of the data. The continuous variables are represented as the mean ± standard deviation or the median and interquartile interval. The different ADCs between the 2 groups were compared using the 2-samples independent t test or the Mann-Whitney U test. The diagnostic performances of various MRI parameters were analyzed using receiver operating characteristics (ROC) curves, and the best threshold, sensitivity, and specificity were calculated. P values <0.05 were considered statistically significant. The clinical and laboratory features as well as the different ADC values were analyzed using a logistic regression to determine the features that were significant when calculated concurrently. The variables included in the multivariate analysis’ had P values <0.5 in the previous bivariate correlation test.
Results
Participant characteristics
In total, 41 patients with COS treated with NACT were included in the final analysis (Table 1). Of the 41 patients with COS, 28 showed a poor response to chemotherapy while 13 showed a good response to chemotherapy according to the histological TNR (Figure 3A-3F).
Table 1
Variable | Values |
---|---|
Poor response | |
Cases | 28 |
Age (years) | |
Median (quartile) | 17.00 (13.00, 19.75) |
Range | 9–45 |
Males, n (%) | 19 (67.86) |
Position, n (%) | |
Lower femur | 13 (46.43) |
Upper tibia | 7 (25.00) |
Upper femur | 2 (7.14) |
Upper humerus | 6 (21.43) |
Good response | |
Cases | 13 |
Age (years) | |
Median (quartile) | 18.00 (13.00, 22.00) |
Range | 9–49 |
Males, n (%) | 10 (76.92) |
Position, n (%) | |
Lower femur | 5 (38.46) |
Upper tibia | 2 (15.38) |
Upper femur | – |
Upper humerus | 6 (46.15) |
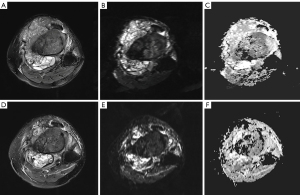
Reproducibility of ADC measurements
The intraobserver ICCs of reader 1 after the 2-month interval were 0.876 [95% confidence interval (CI): 0.780–0.932], 0.883 (95% CI: 0.792–0.936), and 0. 925 (95% CI: 0.864–0.959) for ADC1, ADC2, and ADC3, respectively. There was good reproducibility for the DWI parameter analysis.
The relationship between the ADCs and chemotherapy responses
First, we compared the clinical and laboratory features as well as the different ADCs between the groups (Table 2). The ADC2 and ADC3 of the good-responder group were higher than those of the poor-responder group (P<0.001, P=0.004). The ADC3-1 and ADC3-2 differed significantly between the 2 groups (P=0.008, P=0.047). The preoperative ALP level before chemotherapy of the good-responder group was higher than that of the poor-responder group (P=0.019). There were no significant differences between the 2 groups in terms of age, ADC1, ADC2-1, lactate dehydrogenase before chemotherapy (LDH BC) level, preoperative lactate dehydrogenase after chemotherapy (P LDH AC) level, and preoperative alkaline phosphatase after chemotherapy (P APL AC) level (Table 2).
Table 2
Variables | Good response (n=13) | Poor response (n=28) | t or z | P value |
---|---|---|---|---|
Age (years) | 18.00 (13.00, 22.00) | 17.00 (13.00, 19.75) | –0.312 | 0.757b |
ADC1 (×10−3 mm2/s) | 0.927±0.059 | 0.861±0.038 | –0.953 | 0.346a |
ADC2 (×10−3 mm2/s) | 1.366 (0.990, 1.571) | 0.906 (0.815, 1.145) | 2.269 | <0.001b |
ADC3 (×10−3 mm2/s) | 1.532±0.111 | 1.149±0.067 | –3.091 | 0.004a |
ADC2-1 (×10−3 mm2/s) | 0.414 (0.039, 0.662) | 0.104 (0.058, 0.202) | 1.681 | 0.096b |
ADC3-1 (×10−3 mm2/s) | 0.700 (0.330, 0.931) | 0.219 (0.113, 0.486) | 2.634 | 0.008b |
ADC3-2 (×10−3 mm2/s) | 0.253±0.055 | 0.131±0.032 | –2.047 | 0.047a |
NR (%) | 0.950 (0.900, 0.985) | 0.600 (0.300, 0.700) | –5.367 | 0.000b |
ALP BC (IU/L) | 273.00 (129.50, 860.00) | 148.50 (94.25, 197.00) | 2.312 | 0.019b |
P ALP AC (IU/L) | 102.00 (71.50, 189.00) | 118.50 (79.25, 143.50) | 0.126 | 0.901b |
LDH BC (IU/L) | 259.09 (157.00, 314.86) | 217.50 (170.79, 244.56) | 1.093 | 0.285b |
P LDH AC (IU/L) | 196.00 (165.46, 279.67) | 201.42 (163.34, 238.00) | –0.126 | 0.901b |
a, Two-sample independent t test; b, Nonparametric test (Mann-Whitney); Age is presented as the median (interquartile range); ADC1, ADC3, and ADC3-2 are presented as the mean ± standard deviation. ADC2, NR, ALP BC, P ALP AC, LDH BC, and P LDH AC are presented as the median (interquartile range). ADC1, apparent diffusion coefficient before neoadjuvant chemotherapy; ADC2, apparent diffusion coefficient after the first cycle of neoadjuvant chemotherapy; ADC3, apparent diffusion coefficient after the third cycle of neoadjuvant chemotherapy; ADC2-1, ADC2 − ADC1; ADC3-1, ADC3 − ADC1; ADC3-2, ADC3 − ADC2; ALP BC, alkaline phosphatase before chemotherapy; P ALP AC, preoperative alkaline phosphatase after chemotherapy; LDH BC, lactate dehydrogenase before chemotherapy; P LDH AC, preoperative lactate dehydrogenase after chemotherapy.
Second, we calculated the best threshold values for the abovementioned significantly different ADCs as determined by the ROC curves. The sensitivity and specificity of the respective parameters were also measured for each threshold value to predict the likelihood of a good histological response (Figure 4 and Table 3). An ADC2 ≥0.979×10−3 mm2/s was set as the cutoff value to indicate a good response to NACT, which resulted in a sensitivity of 84.6%, a specificity of 64.3%, and an area under the curve (AUC) of 0.723. An ADC3 value ≥1.499×10−3 mm2/s was set as the cutoff value to indicate a good response to NACT, which resulted in a specificity of 76.9%, a specificity of 75%, and an AUC of 0.747. An ADC3-1 value ≥0.306×10−3 mm2/s was set as the cutoff value to indicate a good response to NACT, which resulted in a sensitivity of 84.6%, a specificity of 71.4%, and an AUC 0.761.
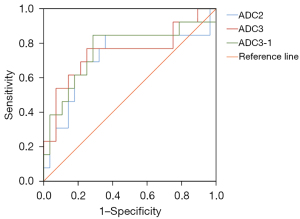
Table 3
Parameter | AUC (95% CI) | Sensitivity (%) | Specificity (%) | Cutoff (10−3 mm2/s) | Youden index | P value |
---|---|---|---|---|---|---|
ADC1 | 0.585 (0.396–0.775) | 0.385 | ||||
ADC2 | 0.723 (0.533–0.912) | 84.6 | 64.3 | 0.979 | 0.489 | 0.023 |
ADC3 | 0.747 (0.564–0.931) | 76.9 | 75 | 1.499 | 0.519 | 0.012 |
ADC2-1 | 0.665 (0.454–0.876) | 0.093 | ||||
ADC3-1 | 0.761 (0.584–0.938) | 84.6 | 71.4 | 0.306 | 0.560 | 0.008 |
ADC3-2 | 0.684 (0.501–0.867) | 0.061 |
AUC, area under the receiver operating characteristic curve; CI, confidence interval; ADC1, apparent diffusion coefficient before neoadjuvant chemotherapy; ADC2, apparent diffusion coefficient after the first cycle of neoadjuvant chemotherapy; ADC3, apparent diffusion coefficient after the third cycle of neoadjuvant chemotherapy. ADC2-1, ADC2 − ADC1; ADC3-1, ADC3 − ADC1; ADC3-2, ADC3 − ADC2.
Prognostic value of the clinical features, laboratory parameters, and the different ADC values in relation to histopathological responses following NACT
The ADC2 (P=0.022), ADC3 (P=0.009), ADC2-1 (P=0.041), and ADC3-1 (P=0.014) were found to be significantly associated with the histopathological responses following NACT based on the logistic regression analysis (Table 4).
Table 4
Characteristics | Univariate analysis | Multivariate analysis | |||
---|---|---|---|---|---|
OR (95% CI) | P | OR (95% CI) | P | ||
Age (years) | 1.012 (0.942, 1.087) | 0.750 | |||
Gender (male/female) | 1.679 (0.347, 7.180) | 0.554 | |||
Pulmonary metastasis | 0.513 (0.127, 2.064) | 0.347 | |||
ADC1 | 4.945 (0.187, 130.757) | 0.339 | |||
ADC2 | 13.916 (1.474, 131.373) | 0.022* | 0.350 (0.003, 42.516) | 0.668 | |
ADC3 | 15.132 (1.988, 115.166) | 0.009* | 33.706 (0.489, 2,323.919) | 0.103 | |
ADC2-1 | 15.546 (1.123, 215.239) | 0.041* | |||
ADC3-1 | 14.074 (1.692, 117.086) | 0.014* | |||
ADC3-2 | 48.556 (0.888, 2,655.352) | 0.057 | |||
ALP BC | 1.002 (1.000, 1.005) | 0.056 | |||
P ALP AC | 1.002 (0.997, 1.006) | 0.475 | |||
LDH BC | 1.006 (0.997, 1.016) | 0.184 | |||
P LDH AC | 1.001 (0.995, 1.007) | 0.723 |
*, risk factors included in the univariate analysis. CI, confidence interval; OR, odds ratio; ADC1, apparent diffusion coefficient before neoadjuvant chemotherapy; ADC2, apparent diffusion coefficient after the first cycle of neoadjuvant chemotherapy; ADC3, apparent diffusion coefficient after the third cycle of neoadjuvant chemotherapy; ADC2-1, ADC2 − ADC1; ADC3-1, ADC3 − ADC1; ADC3-2, ADC3 − ADC2; ALP BC, alkaline phosphatase before chemotherapy; P ALP AC, preoperative alkaline phosphatase after chemotherapy; LDH BC, lactate dehydrogenase before chemotherapy; P LDH AC, preoperative lactate dehydrogenase after chemotherapy.
Discussion
This study examined 41 patients with COS to determine if the ADC after the first cycle of chemotherapy could be used to monitor the effect of NACT and whether the NACT response was affected by clinical features, laboratory parameters, and different ADC values. The results showed that in evaluating the efficacy of NACT for patients with COS, ADC2 had a higher sensitivity (84.6%) than ADC3 (76.9%) while ADC2 had a lower specificity (64.3%) than did ADC3 (75.0%). The sensitivity (84.6% vs. 76.9%) and specificity (64.3% vs. 75%) of ADC2 and ADC3 as factors in monitoring the NACT responses of patients with COS were very close. After the first cycle of chemotherapy, ADC2 can be used to monitor the effect of NACT on osteosarcoma.
Previous studies have largely investigated changes in the ADC before and after all cycles of NACT as factors for monitoring the chemotherapy response of patients with osteosarcoma (12,13), and most of these studies have reported that the ADC of the group with a good response was higher than that of the group with a poor response, which suggests that changes in the ADC may reflect the efficacy of chemotherapy. However, the reliability of these results needs to be further verified, as the sample sizes of these studies were very small, ranging from 8 to 19 patients, and there was no analysis of diagnostic efficacy. Subsequently, the mean diffusivity (MD) diffusion coefficient, mean kurtosis (MK) diffusion coefficient, and ADC of 29 patients with osteosarcoma were examined using diffusion kurtosis imaging. A comparison of the MD diffusion coefficient of post-chemotherapy, the ADC of post-chemotherapy, and the MK diffusion coefficient of post-chemotherapy showed that the MD diffusion coefficient of post-chemotherapy had a higher diagnostic performance (AUC =0.91) than did the ADC of post-chemotherapy (AUC =0.80) and MK diffusion coefficient of post-chemotherapy (AUC =0.72) (13). However, the reliability of these results requires confirmation. Bajpai et al. (12) reported that the ADCs or change rates were not significantly correlated with the TNRs in 31 patients with osteosarcoma; however, their study, the ROI of the ADC measurements included the entire tumor volume, and calcifications, bleeding, and liquefaction could not be avoided. Additionally, the DWI images were taken using a 1.5 T MR scanner. To date, only 1 study has examined the efficacy of the ADC in the middle stage of chemotherapy as an index for monitoring chemotherapy response (14). The middle stage was defined as the most central period from the beginning to the end of chemotherapy. The results showed that the ADC difference between prechemotherapy and midterm chemotherapy was more accurate than the ADC and change rate. However, due to the small sample size (only 15 patients), no diagnostic efficacy analysis was conducted. In addition, due to the side effects of chemotherapy, among other reasons, not all the patients received all the chemotherapy cycles, and thus it was difficult to set the accurate mid-stage time.
In the current study, the ADC2, ADC3, and ADC3-1 results were similar. ADC2 occurred earlier than did the other 2 phases, so ADC2 was an early a preoperative indicator of NACT response. The advantage of ADC2 is that it can determine the efficacy much earlier than ADC3 and thus may even have the potential to help change chemotherapy protocols early if patients do not show a good response; however, further research is needed to confirm this.
Our results shown that ADC2 ≥0.979×10−3 mm2/s was set as the cutoff value to indicate a good response to chemotherapy NACT, which resulted in a sensitivity of 84.6%, a specificity of 64.3%. This value was lower than that of Liu et al. (13), who examined 29 patients with osteosarcoma and found that when the ADC was ≥1.16×10−3 mm2/s after all the chemotherapy cycles, the sensitivity and specificity of the good-response group were 91.7% and 70.6%, respectively. The ADC value before chemotherapy (i.e., the ADC1) did not differ significantly between the 2 groups. These indexes are not suitable for observing chemotherapy responses. It is speculated that the heterogeneity of osteosarcoma in different patients is very large. After chemotherapy, the antitumor vascular effects of the chemotherapeutic drugs, such as tumor cell necrosis, cell number reduction, cell membrane structure damage, the increase of the intercellular space, and the reduced degree of water molecular diffusion movement limitation, will increase the ADC value (14) over a certain period of time.
To our knowledge, studies on the prognostic factors affecting the histopathological response following NACT are limited (15). This was the first study to assess and evaluate the direct correlations of the clinical and laboratory features and different ADC values with the histopathology responses of patients following NACT. Our results showed that ADC2, ADC3, and ADC3-1 were factors associated with the efficacy of NACT in patients with COS. However, we found that the clinical and laboratory characteristics were not factors associated with the efficacy evaluation of TNR in NACT in patients with COS. Based on the multivariate analysis, no factors were significantly associated with the histopathological responses of patients. Thus, the different ADCs were not found to be independent factors associated with the efficacy of NACT in osteosarcoma.
Our study had two potential limitations. First, the number of patients in the poor-responder group was significantly higher than that in the good-responder group (28 vs. 13 cases), which might have affected the reliability of the statistical results. Second, the long-term disease-free survival outcomes were not evaluated. Thus, in the future, studies with large cohorts and multicentric data with standardized and optimized MRI protocols and long-term survival follow-ups need to be conducted.
Conclusions
The ADC2 after the first cycle of NACT is a promising indicator for monitoring the tumor responses of patients with osteosarcoma to NACT. This potential indicator may enable early changes to be made to chemotherapy regimens if patients show no response. Moreover, histopathological changes reflecting the responses to NACT of patients with osteosarcoma can be determined by assessing the different ADCs.
Acknowledgments
Funding: This study was supported by the Science and Technology Program of Hebei Province (No. 22377751D).
Footnote
Reporting Checklist: The authors have completed the STARD reporting checklist. Available at https://qims.amegroups.com/article/view/10.21037/qims-22-1095/rc
Conflicts of Interest: All authors have completed the ICMJE uniform disclosure form (available at https://qims.amegroups.com/article/view/10.21037/qims-22-1095/coif). The authors have no conflicts of interest to declare.
Ethical Statement: The authors are accountable for all aspects of the work in ensuring that questions related to the accuracy or integrity of any part of the work are appropriately investigated and resolved. This study was conducted in accordance with the Declaration of Helsinki (as revised in 2013) and was approved by and registered with the Ethics Committee of The Third Hospital of Hebei Medical University, and the individual consent of each patient was obtained.
Open Access Statement: This is an Open Access article distributed in accordance with the Creative Commons Attribution-NonCommercial-NoDerivs 4.0 International License (CC BY-NC-ND 4.0), which permits the non-commercial replication and distribution of the article with the strict proviso that no changes or edits are made and the original work is properly cited (including links to both the formal publication through the relevant DOI and the license). See: https://creativecommons.org/licenses/by-nc-nd/4.0/.
References
- Testa S, Hu BD, Saadeh NL, Pribnow A, Spunt SL, Charville GW, Bui NQ, Ganjoo KN. A Retrospective Comparative Analysis of Outcomes and Prognostic Factors in Adult and Pediatric Patients with Osteosarcoma. Curr Oncol 2021;28:5304-17. [Crossref] [PubMed]
- Luo Z, Li J, Qin G, Zeng H, He Z, Pan D, Li Y, Chen W, Shen X. Clinical and imaging features of 112 patients with irregular and flat bone osteosarcoma. Quant Imaging Med Surg 2022;12:1988-2001. [Crossref] [PubMed]
- Xu L, Yang P, Hu K, Wu Y, Xu-Welliver M, Wan Y, Luo C, Wang J, Wang J, Qin J, Rong Y, Niu T. Prediction of neoadjuvant chemotherapy response in high-grade osteosarcoma: added value of non-tumorous bone radiomics using CT images. Quant Imaging Med Surg 2021;11:1184-95. [Crossref] [PubMed]
- Kubo T, Furuta T, Johan MP, Ochi M, Adachi N. Value of diffusion-weighted imaging for evaluating chemotherapy response in osteosarcoma: A meta-analysis. Mol Clin Oncol 2017;7:88-92. [Crossref] [PubMed]
- Huvos AG, Rosen G, Marcove RC. Primary osteogenic sarcoma: pathologic aspects in 20 patients after treatment with chemotherapy en bloc resection, and prosthetic bone replacement. Arch Pathol Lab Med 1977;101:14-8. [PubMed]
- Degnan AJ, Chung CY, Shah AJ. Quantitative diffusion-weighted magnetic resonance imaging assessment of chemotherapy treatment response of pediatric osteosarcoma and Ewing sarcoma malignant bone tumors. Clin Imaging 2018;47:9-13. [Crossref] [PubMed]
- Hayashida Y, Yakushiji T, Awai K, Katahira K, Nakayama Y, Shimomura O, Kitajima M, Hirai T, Yamashita Y, Mizuta H. Monitoring therapeutic responses of primary bone tumors by diffusion-weighted image: Initial results. Eur Radiol 2006;16:2637-43. [Crossref] [PubMed]
- Uhl M, Saueressig U, Koehler G, Kontny U, Niemeyer C, Reichardt W, Ilyasof K, Bley T, Langer M. Evaluation of tumour necrosis during chemotherapy with diffusion-weighted MR imaging: preliminary results in osteosarcomas. Pediatr Radiol 2006;36:1306-11. [Crossref] [PubMed]
- Zhao S, Su Y, Duan J, Qiu Q, Ge X, Wang A, Yin Y. Radiomics signature extracted from diffusion-weighted magnetic resonance imaging predicts outcomes in osteosarcoma. J Bone Oncol 2019;19:100263. [Crossref] [PubMed]
- Kubo T, Furuta T, Johan MP, Adachi N, Ochi M. Percent slope analysis of dynamic magnetic resonance imaging for assessment of chemotherapy response of osteosarcoma or Ewing sarcoma: systematic review and meta-analysis. Skeletal Radiol 2016;45:1235-42. [Crossref] [PubMed]
- Hongtao L, Hui Z, Bingshun W, Xiaojin W, Zhiyu W, Shuier Z, Aina H, Yuanjue S, Daliu M, Zan S, Yang Y. 18F-FDG positron emission tomography for the assessment of histological response to neoadjuvant chemotherapy in osteosarcomas: a meta-analysis. Surg Oncol 2012;21:e165-70. [Crossref] [PubMed]
- Bajpai J, Gamnagatti S, Kumar R, Sreenivas V, Sharma MC, Khan SA, Rastogi S, Malhotra A, Safaya R, Bakhshi S. Role of MRI in osteosarcoma for evaluation and prediction of chemotherapy response: correlation with histological necrosis. Pediatr Radiol 2011;41:441-50. [Crossref] [PubMed]
- Liu C, Xi Y, Li M, Jiao Q, Zhang H, Yang Q, Yao W. Monitoring Response to Neoadjuvant Chemotherapy of Primary Osteosarcoma Using Diffusion Kurtosis Magnetic Resonance Imaging: Initial Findings. Korean J Radiol 2019;20:801-11. [Crossref] [PubMed]
- Baunin C, Schmidt G, Baumstarck K, Bouvier C, Gentet JC, Aschero A, Ruocco A, Bourlière B, Gorincour G, Desvignes C, Colavolpe N, Bollini G, Auqier P, Petit P. Value of diffusion-weighted images in differentiating mid-course responders to chemotherapy for osteosarcoma compared to the histological response: preliminary results. Skeletal Radiol 2012;41:1141-9. [Crossref] [PubMed]
- Prabowo Y, Setiawan I, Kamal AF, Kodrat E, Labib Zufar ML. Correlation between Prognostic Factors and the Histopathological Response to Neoadjuvant Chemotherapy in Osteosarcoma: A Retrospective Study. Int J Surg Oncol 2021;2021:8843325. [Crossref] [PubMed]