Precision of quantitative parameters of 18F-FDG PET/CT in a rabbit VX2 tumor model
Introduction
Quantitative flourine-18 fluorodeoxyglucose positron emission tomography (18F-FDG PET) is believed to be a suitable assessment tool to evaluate tumor response to therapy (1). It can provide the main parameters including the standardized uptake value (SUV), SUV corrected for lean body mass (SUL), and total lesion glycolysis (TLG) values (2-4). However, quantitative analysis in 18F-FDG imaging is affected by various factors such as the definition of the region of interest, the pixel size, low resolution reconstruction, patient’s weight (body mass index) and the state of fasting blood glucose levels, and 18F-FDG injection leakage (5). Given the multitude of factors that affect quantitative analysis in 18F-FDG imaging, an understanding of the repeatability of the technique is required to make good use of the parameters for response assessment.
Precision is the degree of repeatability. A good precision could ensure that repeated measurements under unchanged physiological conditions give the same results. Adequate repeatability is essential for the clinical 18F-FDG PET/CT evaluation of patients with cancer within multi-center trials. In general, standard deviation (SD) and coefficient of variation (CV) can statistically reflect the degree of variation in data. Furthermore, the least significant change (LSC) should be also calculated when considering precision. The LSC is usually calculated by 15 individuals scanned three times each, 10 individuals scanned four times each, or 30 individuals scanned twice each (6). For example, in the drug treatment of osteoporosis, LSC of bone density is employed to evaluate whether a real biologic change occurred or if the change was caused by errors before and after the treatment. Until recently, only a limited number of studies elucidated 18F-FDG PET reproducibility in humans with cancer (7-11). The application of LSC in the response evaluation criteria in solid tumors using PET has not been formally proposed. In fact, due to the increased radiation exposure to 18F-FDG and CT scans and the medical ethics considerations, repeated PET/CT scanning in human participants for many days on end is not appropriate.
The VX2 rabbit tumor model consisted of immune-competent rabbits that were implanted with a Shope papillomavirus-associated carcinoma. The high survival rate made the rabbit VX2 tumor model a suitable candidate for in vivo inoculation. The rabbit model is more practical for a PET/CT scan compared to some other animal model such as rodent. Previous studies have employed this model to determine tumor response to therapy using PET/CT scans (12-16). However, no studies have been published with respect to precision for this model. The objective of the current study was to establish a method to assess quantitative precision in employing PET imaging of solid tumors before and after treatment.
Methods
Rabbit VX2 tumor model
Experiments were performed under a project license (No. 2015081001) granted by the Laboratory Animal Ethics Committee, Jinan University, in compliance with international Association for Assessment and Accreditation of Laboratory Animal Care guidelines for the care and use of animals. A total of 18 healthy male New Zealand rabbits, with a weight between 2.0 and 2.5 kg and age between 3 and 4 months old, were used in this study. All male New Zealand rabbits were specific pathogen-free animals in compliance with national laboratory animal standards. The animals were housed in cages of 2 rabbits, and the animal room was mainly illuminated by natural lighting, adjusted with incandescent lighting to achieve 12/12 h light/dark cycles. The animals were fed fodder, and drinking water ad libitum.
Fresh VX2 tumor tissue was surgically removed from the tumor-bearing rabbit (the weight and age were similar for the 18 healthy male New Zealand white rabbits), once executed. The fish-shaped solid part of the VX2 tumor tissue was placed in phosphate buffer saline (PBS), carefully cleaned, and minced into 1 mm3 pieces using an ophthalmic scissor.
Eighteen healthy male normal New Zealand white rabbits were anesthetized by intramuscular injection of 3% pentobarbital (0.8 mL/kg). Hair removal, skin preparation, disinfection, and 1 cm incision were successively performed on the rabbit’s right thigh for inoculation, and then 1–2 pieces of tumor tissue were slowly implanted into the intramuscular location. The vaccination region was carefully stitched and intramuscularly injected with 200,000 units of penicillin for three consecutive days to prevent infection. Tumor growth was observed every 24 h. After 2 weeks, the tumor nodules round in shape with a diameter of approximately 1 cm were palpable at the skin surface, and the VX2 tumor model could be used for experiments. All steps were strictly aseptic (17).
Of the 18 rabbits, three were used for a dynamic 18F-FDG PET/CT study to determine the best scanning time point after injection in VX2 tumor-bearing rabbits and 15 were used for a precision experiment which was performed by repeating 18F-FDG PET/CT scans for three consecutive days.
Tumor-bearing rabbits were sacrificed after the last scan; the tumor tissue was removed and fixed with 4% paraformaldehyde for more than 24 h and embedded in paraffin. Pathologic specimens were obtained and stained using both hematoxylin-eosin (HE) and glucose transporter-1 (GLUT-1) immunohistochemistry. HE staining: paraffin sections were dewaxed to water, nucleus was stained with hematoxylin, and cytoplasm was stained with eosin and then was dehydrated and sealed. GLUT-1 immunohistochemistry: paraffin sections were dewaxed to water; antigen was repaired; endogenous peroxidase was blocked; bovine serum albumin or serum was blocked; mouse anti-GLUT-1 monoclonal antibody (1:200 dilution, incubated at 4 ℃ overnight) and corresponding secondary antibody (incubated at room temperature for 1 h) were added; 3,3-diaminobenzidine (DAB) was added; the nucleus was re-stained, dehydrated, and sealed; subsequently, microscopic examination, image collection, and analysis were performed. Hematoxylin staining rendered the nucleus blue, and a brown-colored precipitate indicated a positive expression of DAB.
18F-FDG PET/CT imaging
18F-FDG PET/CT examinations were performed with the same protocol using a GE Discovery PET/CT 690 system. 18F-FDG was provided by the accelerator center of Guangzhou Atom High Tech Isotope, and its radiochemical purity was higher than 95%.
Three rabbits prepared for the VX2 tumor model were fasted for at least 8 h prior to intravenous injection of 7.4 MBq/kg (0.2 mCi/kg) of 18F-FDG. The body weight and blood sugar were also measured. The three rabbits were under anesthesia (pentobarbital 0.8 mL/kg via ear vein bolus injection) and then put in prone position to perform 0–90 min dynamic PET/CT scan post radiotracer injection. Dynamic scan data were partitioned into 33 frames as follows: 6×10, 8×30, 5×60, 12×300, and 2×600 s. Post processed data was analyzed using Dynamic software in Xeleris workstation (GE Healthcare) to obtain the time-activity curves (TACs) of VX2 tumor tissue and normal liver tissue. Furthermore, a quick check of the true coincident counts in the rabbit was done 24 h after the PET/CT examination to determine whether the residue of the radiotracer had an impact on the next imaging session.
Fifteen rabbits were prepared as above. All the rabbits were under anesthesia (pentobarbital 0.8 mL/kg via ear vein injection) and then put in prone position to perform static PET/CT scan for 40–50 min (results come from a dynamic 18F-FDG PET/CT scan in three rabbits) post radiotracer injection. The CT data were acquired with an automatic dose modulation at 120 kV, 30 mA, a 512×512 image matrix, a field of view (FOV) of 70 cm, a noise index of 30, a reconstructed slice thickness of 3.75 mm. The PET protocol encompassed three bed positions with a scan duration of 180 s per bed position. The PET data was acquired in 3D time-of-flight mode using the adaptive statistical iterative reconstruction with a 30 cm transverse FOV and 15.7 cm axial FOV, reconstructed slice thickness of 3.27 mm, slice interval of 3.27 mm, and 256×256 image matrix. The attenuation correction was based on the CT. Each rabbit was scanned in this manner for 9–12 min per day (18). For each PET/CT scan (second and third visits), re-injection with 18F-FDG was required. All 15 tumor-bearing rabbits were scanned once per day for three consecutive days. The Advantage Workstation (GE Healthcare), version 4.6, was used for image post-processing. The PET images were reconstructed using an ordered-set expectation-maximization (OSEM) algorithm and CT scans were reconstructed with a section thickness of 3.75 mm and overlap between slices 0.48 mm to match the parameters of the PET scan. All the rabbits were returned to the animal breeding center of Jinan University after PET scan. We used the lean body mass (LBM) values measured by dual energy X-ray absorptiometry (DXA) to calculate the standardized uptake value (SUV) corrected for LBM (SUL). The tumor outlines were drawn layer by layer manually on CT images to obtain the tumor volume. The SUV values (SUVmax, SUVmean, and SUVpeak), SUL values (SULmax, SULmean, and SULpeak), and were measured in the PET volume computer-assisted reading (PET VCAR) software (GE Healthcare).
DXA scanning
DXA scan was performed using Lunar Prodigy (GE Healthcare, Madison, WI) after the third PET/CT scan to measure the body composition of tumor-bearing rabbits. The enCORE software (version 10.0, standard-array mode; GE Healthcare, Madison, WI, USA) was used to determine the bone mineral content (BMC), fat mass (FM), and lean mass (LM). DXA acquisitions were operated by an experienced technician, and the results were reviewed by two senior physicians. The sum of LM and total BMC measured by DXA is equal to LBM (19).
PET precision calculation
The precision is equivalent to the root mean square (RMS) of SD or CV. The formulae are as follows (20):
where m is the number of samples.
The formula of LSC is as follows:
where Z’ is based on the statistical confidence level, Pr is the precision value (either RMS-SD or RMS-CV), n1 is the number of first measurements, and n2 is the number of follow-up measurements.
Statistical analysis
Statistical analysis was performed using the SPSS (version 17.0) software package. All data are expressed as the mean ± SD. The two-way analysis of variance (ANOVA) was used for inter-group comparison, and P values less than 0.05 were considered statistically significant.
Results
The results of dynamic 18F-FDG PET/CT scan in three rabbits revealed that the SUV uptake of tumor tissue increased with time and tended to be stable after 40 min post-injection, as shown in Figure 1. The SUV uptake of normal liver tissue peaked rapidly and then began to decrease until it tended to be steady at similar time point post-injection. Accordingly, the acquisition time of 18F-FDG PET/CT was determined to be 40–50 min after 18F-FDG injection. Three repeated 18F-FDG PET/CT scans (Figure 2) were performed for three consecutive days for all 15 rabbits. The VX2 tumors were successfully implanted in 18 rabbits’ right thigh and confirmed by pathology; the results of immunohistochemistry confirmed GLUT-1 positivity (Figure 3).
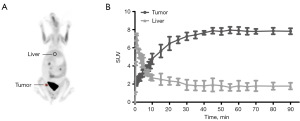
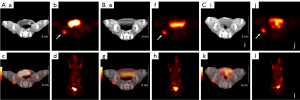
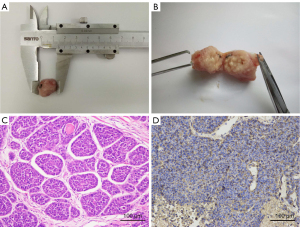
The mean body weight, blood glucose, injection dose, and uptake time of all 15 VX2 tumor models for three consecutive days are shown in Table 1. The mean tumor volume of 15 rabbits calculated from volume of interest (VOI) on CT images of three consecutive days had no statistical differences (Table 1). The mean LM, FM, and BMC of 15 rabbits measured by DXA were 1.911±0.288, 0.115±0.066, and 0.050±0.005 kg, respectively. The LM accounted for 97.2% of total body weight. The metabolic parameters including SUV (SUVmax, SUVmean, and SUVpeak), SUL (SULmax, SULmean and SULpeak), TLG (TLGSUV and TLGSUL) are also shown in Table 1. The TLGSUV and TLGSUL had statistically significant difference (P<0.05), and other metabolic parameters showed no significance (P>0.05).
Table 1
Parameter | First day | Second day | Third day |
---|---|---|---|
Weight (kg) | 2.11±0.35 | 2.18±0.31 | 2.15±0.30 |
Blood glucose (mmol/L) | 5.67±1.30 | 6.10±1.29 | 5.73±1.93 |
Dose of 18F-FDG (mCi) | 0.43±0.08 | 0.45±0.07 | 0.42±0.08 |
Uptake time (min) | 49.9±6.7 | 47.8±6.2 | 47.8±5.9 |
Tumor volume in CT (cm3) | 1.22±0.59 | 1.26±0.64 | 1.29±0.72 |
SUVmax | 5.72±1.48 | 6.02±1.76 | 5.90±1.45 |
SUVmean | 3.49±1.00 | 3.68±1.05 | 3.62±0.90 |
SUVpeak | 3.35±1.23 | 3.63±1.19 | 3.78±1.23 |
TLGSUV | 4.55±2.70a,b | 5.25±2.74c | 6.50±3.75 |
LiverSUV | 1.46±0.38 | 1.69±0.35 | 1.64±0.29 |
SULmax | 5.46±1.41 | 5.63±1.62 | 5.53±1.30 |
SULmean | 3.33±0.95 | 3.43±0.97 | 3.40±0.81 |
SULpeak | 3.19±1.16 | 3.39±1.12 | 3.54±1.12 |
TLGSUL | 4.33±2.55a,b | 4.93±2.59c | 6.05±3.46 |
LiverSUL | 1.39±0.38 | 1.61±0.36 | 1.57±0.29 |
Data were presented as mean ± standard deviation. a, P<0.05 (first day vs. second day); b, P<0.05 (first day vs. third day); c, P<0.05 (second day vs. third day). PET, positron emission tomography; 18F-FDG, flourine-18 fluorodeoxyglucose; CT, computed tomography; SUVmax, maximum SUV of target lesion; SUVmean, average SUV of target lesion; SUVpeak, SUVpeak of target lesion; TLGSUV, total lesion glycolysis = SUVmean × metabolism total volume; LiverSUV, average SUVmean in liver; SULmax, maximum SUL of target lesion; SULmean, average SUL of target lesion; SULpeak, SULpeak of target lesion; TLGSUV, total lesion glycolysis = SULmean × metabolism total volume; LiverSUL, average SULmean in liver; SUV, standardized uptake value; SUL, SUV corrected for lean body mass.
The precision (RMS-CV and RMS-SD) of all metabolic parameters are shown in Table 2, as well as the LSC (RMS-CV and RMS-SD) with 80% and 95% confidence intervals (CIs). The precision RMS-CV% ranged from 18.0% to 18.8%. The LSC80 and LSC95RMS-CV% ranged from 32.6% to 33.9% and 50.0% to 52.1%, respectively.
Table 2
Parameter | SUVmax | SUVmean | SUVpeak | SULmax | SULmean | SULpeak |
---|---|---|---|---|---|---|
Pr | ||||||
RMS-CV% | 18.3% | 18.7% | 18.8% | 18.0% | 18.4% | 18.4% |
RMS-SD | 1.16 | 0.74 | 0.79 | 1.09 | 0.69 | 0.73 |
1×1LSC80 | ||||||
RMS-CV% | 33.1% | 33.9% | 34.1% | 32.6% | 33.3% | 33.3% |
RMS-SD | 2.10 | 1.34 | 1.43 | 1.96 | 1.25 | 1.33 |
1×1LSC95 | ||||||
RMS-CV% | 50.1% | 51.8% | 52.1% | 50.0% | 51.0% | 51.0% |
RMS-SD | 3.22 | 2.04 | 2.18 | 3.00 | 1.91 | 2.03 |
LSC, least significant change; Pr, precision; 1×1LSC80, the least significant changes for one scan at baseline and one at follow-up at 80% confidence; 1×1LSC95, the least significant changes for one scan at baseline and one at follow-up at 95% confidence; RMS-CV, the root mean square of coefficient of variation; RMS-SD, the root mean square of standard deviation; SUVmax, maximum SUV of target lesion; SUVmean, average SUV of target lesion; SUVpeak, SUVpeak of target lesion; SULmax, maximum SUL of target lesion; SULmean, average SUL of target lesion; SULpeak, SULpeak of target lesion; SUV, standardized uptake value; SUL, SUV corrected for lean body mass.
Discussion
VX2 is a rabbit squamous cell carcinoma which has been extensively used as a model for malignant tumor (18). Moreover, the rabbit VX2 tumor model has been employed in evaluating solid tumor response to therapy in animals in vivo (17). Actually, VX2 tumors rabbit models were widely used to inoculate in livers as preclinical investigations of hepatocellular carcinoma (21). The liver was recommended as the background in the PET Response Criteria in Solid Tumors (PERCIST), version 1.0, so we avoided inoculating VX2 tumor in the liver (4,22). The reason why we choose to inoculate in the thigh was that it had the advantages of determining the experimental time after observing the tumor volume by palpation in vitro and was relatively easy to operate for the precision test of 18F-FDG PET/CT imaging. Studies have shown that the expression of GLUT-1 may be one of the reasons for high 18F-FDG uptake in esophageal squamous cell carcinoma (ESCC) and non-small cell lung cancer (NSCLC) (23,24). Although the FDG uptake was based on multiple factors such as the Warburg effect, the high SUV value in VX2 tumor tissue could be attributed to the GLUT-1 expression.
Previous PET data of rabbit VX2 tumor model were collected during the period of 45–60 minutes after 18F-FDG injection (12,16). However, no explanation with respect to the acquisition time point was provided. In the present study, a preliminary experiment of dynamic PET/CT imaging was conducted to determine the best time point of acquisition. The TACs of the tumor tissue and normal liver were acquired from a 0–90 min dynamic PET study, and the results showed that the 18F-FDG uptake was stable during the 40–90-min period post injection (Figure 1). Therefore, the 40–50-min period after 18F-FDG injection was considered as the appropriate acquisition time period for emission in subsequent experiments. The SUV might be unstable if it was <40 min, and the rabbit might emerge from anesthesia if >50 min. Our results showed that the 40–50 min period was optimal according to this model, but whether it can be extended to others depends on the size of the tumor and factors such as blood supply. Consequently, we suggest that a dynamic PET acquisition should be conducted prior to PET scanning with different animal models as experimental objects to obtain the best collection time of corresponding animal models.
Our results found that the mean tumor volume drawn in CT images of three consecutive days were not statistically different, which was consistent with previous studies conducted by Xu et al. (14) and Song et al. (18). In 18F-FDG PET/CT imaging, the lesion with high 18F-FDG uptake was seen on the vaccination area of the VX2 tumor tissue for every tumor-bearing rabbit. The mean SUVmax in 3 days in the lesion ranged from 5.72 to 6.02, which was four times higher than that in the normal liver tissue, which ranged from 1.46 to 1.69. Oya et al. (25) reported similar results and showed that the tumor had 3.5±0.9 times higher 18F-FDG uptake than the normal liver.
SUV, defined as the ratio of activity in tissue per milliliter to the activity in the injected dose per kilogram patient body weight, is the most widely used method for quantitative assessment of clinical PET. However, SUV generated by normalization of 18F-FDG uptake to patient body weight overestimates 18F-FDG uptake in heavy patients, as their fraction of body fat (with low 18F-FDG uptake) is often increased (26). SUV normalized by LBM is becoming a popular technique and has also been advocated as the preferred method for quantifying metabolic activity for treatment response assessment in clinical trials (4). LBM can be accurately measured by the DXA method. DXA has gained wider acceptability as a research tool for the evaluation of body composition because it provides a precise body composition analysis (27). DXA measures the soft tissue and bone mass independently and then separates the soft tissue into LM and FM. The LBM as measured by DXA can make the SUL calculation results accurate and reliable. The precision of SUV parameters, including SUVmax, SUVmean, and SUVpeak, ranged from 18.3% to 18.8%, similar to all the SUL parameters (18.0–18.4%), including SULmax, SULmean, and SULpeak. The DXA analysis showed that FM accounted for only one percent of total body weight in the experimental rabbits, which could be the reason all the SUV and SUL parameters exhibited minor difference.
Precision is essential for the assessment of solid tumor response to therapy using 18F-FDG PET/CT imaging. In theory, precision is divided into short-term and long-term precision according to the experimental time. A long-term precision study in which patients are followed over the course of at least 1 year would be preferable to a short-term precision study but is logistically more difficult. On the other hand, short-term precision studies need to be completed within two weeks to one month. As an indicator of repeatability, the short-term precision is usually used to measure the LSC of quantitative parameters. For statistical validity, it is recommended that the number of individuals and scans per individual is determined on statistical grounds to ensure a minimum of 30 degrees of freedom (df), which is chosen to ensure that the upper limit for the 95% CI of the precision value calculated is no more than 34% greater than the calculated precision value (20). One such combination is 15 individuals scanned three-times each. Other combinations include 10 individuals scanned four times each and 30 individuals scanned twice each. In our study, the PET/CT scans were repeated thrice for 15 rabbits, and the measurements of mean, SD, and CV were obtained. Then, the mean, SD, and CV for each set of measurements for an individual and the root-mean square SD (RMS-SD) and root-mean square CV (RMS-CV) for the entire group were calculated. The RMS-SD/RMS-CV was the short-term precision value for the VX2 tumor. To our knowledge, the impact of precision on animal PET/CT imaging in the VX2 tumor model has not been reported previously. Our study was the first study to propose and establish the precision experimental method and calculate LSC in the rabbit VX2 tumor PET imaging experiment.
In previous studies, LSC was used to evaluate the efficacy of osteoporosis drug therapy, and the change in bone mineral density (BMD) before and after treatment which exceeded the calculated LSC (28,29). The purpose of this study was to explore the possibility of LSC in PET quantitative evaluation of the therapeutic effect of solid tumor. SUVmax and SULpeak are the most commonly used parameters in 18F-FDG PET/CT imaging. The study calculated the credibility of the 80% and 95% SUVmax/SULpeak LSC (RMS-CV% and RMS-SD). We wanted to use LSC in employing PET imaging of a rabbit VX2 tumor model before and after treatment at a later stage which was our future research direction.
In general, the reliability of 95% statistical confidence level is the ideal requirement. In the evaluation of the efficacy of osteoporosis drug therapy, 80% statistical confidence can usually meet the need of clinical judgment (20). PET Response Criteria in Solid Tumors (PERCIST), version 1.0, defined 30% as a cut-off value on the basis of previous studies on repeatability; when the SULpeak before and after treatment changes in more than 30%, it is associated with a metabolic response or disease (4). In 2008, Song et al. (18) inferred that an SUV decrease of 30% or more was a suitable criterion to judge whether individuals were sensitive to particular chemotherapeutic drugs in a rabbit VX2 tumor model. According to our results, the 80% SUVmax/SULpeak LSC was 33.1%/33.3%, which was close to 30%. For this reason, we proposed that 80% confidence level in the efficacy of solid tumors might be more reasonable. In addition, for animal studies of tumor therapeutic drugs, whether LSC is calculated with 80% or 95% confidence may still require more rigorous animal experiments based on PET solid tumor efficacy evaluation and their pathological results as support. The ultimate purpose of the precision experiment was to provide a basis for the efficacy evaluation of tumor treatment drugs or treatment methods at a later stage and to increase the reliability of the final results. However, the current research is to establish a precision method based on 18F-FDG PET solid tumor evaluation, and the experimental research of VX2 tumor treatment effect evaluation based on the precision evaluation method will be carried out. Therefore, this was the inadequacy of this study and our future research direction.
TLG was considered a predictor of clinical efficacy value in some literatures (30-32). To the best of our knowledge, no previous TLG study has been published in animal PET/CT imaging for assessment of tumor response to therapy. In this paper, the TLGSUV and TLGSUL had statistically significant difference between three groups. It might be related to the definition of TLG. TLG was the product of metabolic tumor volume (MTV) multiplied by SUVmean. The product of the two variables leaded to an increase in error. Thus, we did not make further efforts to calculate the TLG precision results. The results here demonstrated that TLG might not be an effective additional parameter in terms of explaining the assessment of tumor response to therapy in the rabbit VX2 tumor model.
Conclusions
GLUT-1 was expressed in the VX2 tumor cell membrane. Our study established the method to assess quantitative precision of a solid tumor in a rabbit VX2 tumor model using PET imaging. Moreover, the LSC of SUV was calculated which was approximately 33% at 80% confidence level, which will serve as a foundation for the evaluation of therapeutic effect in the future. TLG changed greatly and was not suitable for precision evaluation.
Acknowledgments
We thank the staff members of the Department of Nuclear Medicine, First Affiliated Hospital of Jinan University for excellent technical support.
Funding: This work was supported by the National Natural Science Foundation of China (No. 81871383), the Medical Scientific Research Foundation of Guangdong Province (No. A2018135), the Fundamental Research Funds for the Central Universities (No. 21617311), and the Science and Technology Program of Guangzhou (No. 201804010440).
Footnote
Conflicts of Interest: All authors have completed the ICMJE uniform disclosure form (available at https://qims.amegroups.com/article/view/10.21037/qims-22-1079/coif). All authors report that this work was supported by the National Natural Science Foundation of China (No. 81871383), Medical Scientific Research Foundation of Guangdong Province (No. A2018135), Fundamental Research Funds for the Central Universities (No. 21617311) and Science and Technology Program of Guangzhou (No. 201804010440). The authors have no other conflicts of interest to declare.
Ethical Statement: The authors are accountable for all aspects of the work in ensuring that questions related to the accuracy or integrity of any part of the work are appropriately investigated and resolved. Experiments were performed under a project license (No. 2015081001) granted by the Laboratory Animal Ethics Committee, Jinan University, in compliance with international Association for Assessment and Accreditation of Laboratory Animal Care guidelines for the care and use of animals.
Open Access Statement: This is an Open Access article distributed in accordance with the Creative Commons Attribution-NonCommercial-NoDerivs 4.0 International License (CC BY-NC-ND 4.0), which permits the non-commercial replication and distribution of the article with the strict proviso that no changes or edits are made and the original work is properly cited (including links to both the formal publication through the relevant DOI and the license). See: https://creativecommons.org/licenses/by-nc-nd/4.0/.
References
- Deroose CM, Stroobants S, Liu Y, Shankar LK, Bourguet P. Using PET for therapy monitoring in oncological clinical trials: challenges ahead. Eur J Nucl Med Mol Imaging 2017;44:32-40. [Crossref] [PubMed]
- Shang J, Ling X, Zhang L, Tang Y, Xiao Z, Cheng Y, Guo B, Gong J, Huang L, Xu H. Comparison of RECIST, EORTC criteria and PERCIST for evaluation of early response to chemotherapy in patients with non-small-cell lung cancer. Eur J Nucl Med Mol Imaging 2016;43:1945-53. [Crossref] [PubMed]
- Sugawara Y, Zasadny KR, Neuhoff AW, Wahl RL. Reevaluation of the standardized uptake value for FDG: variations with body weight and methods for correction. Radiology 1999;213:521-5. [Crossref] [PubMed]
- Wahl RL, Jacene H, Kasamon Y, Lodge MA. From RECIST to PERCIST: Evolving Considerations for PET response criteria in solid tumors. J Nucl Med 2009;50:122S-50S. [Crossref] [PubMed]
- Boellaard R. Need for standardization of 18F-FDG PET/CT for treatment response assessments. J Nucl Med 2011;52:93S-100S. [Crossref] [PubMed]
- Bonnick SL. Monitoring changes in bone density. Womens Health (Lond) 2008;4:89-97. [Crossref] [PubMed]
- de Langen AJ, Vincent A, Velasquez LM, van Tinteren H, Boellaard R, Shankar LK, Boers M, Smit EF, Stroobants S, Weber WA, Hoekstra OS. Repeatability of 18F-FDG uptake measurements in tumors: a metaanalysis. J Nucl Med 2012;53:701-8. [Crossref] [PubMed]
- Rasmussen JH, Fischer BM, Aznar MC, Hansen AE, Vogelius IR, Löfgren J, Andersen FL, Loft A, Kjaer A, Højgaard L, Specht L. Reproducibility of (18)F-FDG PET uptake measurements in head and neck squamous cell carcinoma on both PET/CT and PET/MR. Br J Radiol 2015;88:20140655. [Crossref] [PubMed]
- Weber WA, Gatsonis CA, Mozley PD, Hanna LG, Shields AF, Aberle DR, Govindan R, Torigian DA, Karp JS, Yu JQ, Subramaniam RM, Halvorsen RA, Siegel BA. ACRIN 6678 Research team; MK-0646-008 Research team. Repeatability of 18F-FDG PET/CT in Advanced Non-Small Cell Lung Cancer: Prospective Assessment in 2 Multicenter Trials. J Nucl Med 2015;56:1137-43. [Crossref] [PubMed]
- Minn H, Zasadny KR, Quint LE, Wahl RL. Lung cancer: reproducibility of quantitative measurements for evaluating 2-F-18-fluoro-2-deoxy-D-glucose uptake at PET. Radiology 1995;196:167-73. [Crossref] [PubMed]
- Kumar V, Nath K, Berman CG, Kim J, Tanvetyanon T, Chiappori AA, Gatenby RA, Gillies RJ, Eikman EA. Variance of SUVs for FDG-PET/CT is greater in clinical practice than under ideal study settings. Clin Nucl Med 2013;38:175-82. [Crossref] [PubMed]
- Kim JI, Lee HJ, Kim YJ, Kim KG, Lee KW, Lee JH, Lee HJ, Lee WW. Multiparametric monitoring of early response to antiangiogenic therapy: a sequential perfusion CT and PET/CT study in a rabbit VX2 tumor model. ScientificWorldJournal 2014;2014:701954. [Crossref] [PubMed]
- Xu H, Sun G, Wang H, Yue Q, Tang H, Wu Q. Dynamic observation of the radiosensitive effect of irisquinone on rabbit VX2 lung transplant tumors by using fluorine-18-deoxyglucose positron emission tomography/computed tomography. Nucl Med Commun 2013;34:220-8. [Crossref] [PubMed]
- Xu Yp, Yang M. Pan Dh, Wang Lz, Liu L, Huang P, Shao G. Bioevaluation study of 32P-CP-PLLA particle brachytherapy in a rabbit VX2 lung tumor model. Appl Radiat Isot 2012;70:583-8. [Crossref] [PubMed]
- Liapi E, Geschwind JF, Vali M, Khwaja AA, Prieto-Ventura V, Buijs M, Vossen JA, Ganapathy-Kanniappan S, Wahl RL. Assessment of tumoricidal efficacy and response to treatment with 18F-FDG PET/CT after intraarterial infusion with the antiglycolytic agent 3-bromopyruvate in the VX2 model of liver tumor. J Nucl Med 2011;52:225-30. [Crossref] [PubMed]
- Song SL, Deng C, Wen LF, Liu JJ, Wang H, Feng D, Wong CY, Huang G. 18F-FDG PET/CT-related metabolic parameters and their value in early prediction of chemotherapy response in a VX2 tumor model. Nucl Med Biol 2010;37:327-33. [Crossref] [PubMed]
- Ishii K, Hosono MN, Wada Y, Maeda M, Kondo S, Takada Y, Tada T, Okamura T, Watanabe Y, Inoue Y. Usefulness of FDG-microPET for early evaluation of therapeutic effects on VX2 rabbit carcinoma. Ann Nucl Med 2006;20:123-30. [Crossref] [PubMed]
- Song SL, Liu JJ, Huang G, Wang ZH, Song YY, Sun XG, Chen T. Changes in 18F-FDG uptake within minutes after chemotherapy in a rabbit VX2 tumor model. J Nucl Med 2008;49:303-9. [Crossref] [PubMed]
- Decazes P, Métivier D, Rouquette A, Talbot JN, Kerrou K. A Method to Improve the Semiquantification of 18F-FDG Uptake: Reliability of the Estimated Lean Body Mass Using the Conventional, Low-Dose CT from PET/CT. J Nucl Med 2016;57:753-8. [Crossref] [PubMed]
- Bonnick SL, Johnston CC Jr, Kleerekoper M, Lindsay R, Miller P, Sherwood L, Siris E. Importance of precision in bone density measurements. J Clin Densitom 2001;4:105-10. [Crossref] [PubMed]
- Parvinian A, Casadaban LC, Gaba RC. Development, growth, propagation, and angiographic utilization of the rabbit VX2 model of liver cancer: a pictorial primer and "how to" guide. Diagn Interv Radiol 2014;20:335-40. [Crossref] [PubMed]
- Cao Y, Zhou K, Diao W, Long X, Tian F, Su M, Jia Z. Age-related changes of standardized uptake values in the blood pool and liver: a decade-long retrospective study of the outcomes of 2,526 subjects. Quant Imaging Med Surg 2021;11:95-106. [Crossref] [PubMed]
- Kaida H, Kawahara A, Hayakawa M, Hattori S, Kurata S, Fujimoto K, Azuma K, Hirose Y, Takamori S, Hiromatsu Y, Nakashima T, Fujita H, Kage M, Hayabuchi N, Ishibashi M. The difference in relationship between 18F-FDG uptake and clinicopathological factors on thyroid, esophageal, and lung cancers. Nucl Med Commun 2014;35:36-43. [Crossref] [PubMed]
- Botha H, Farah CS, Koo K, Cirillo N, McCullough M, Paolini R, Celentano A. The Role of Glucose Transporters in Oral Squamous Cell Carcinoma. Biomolecules 2021;11:1070. [Crossref] [PubMed]
- Oya N, Nagata Y, Tamaki N, Takagi T, Murata R, Magata Y, Abe M, Konishi J. FDG-PET evaluation of therapeutic effects on VX2 liver tumor. J Nucl Med 1996;37:296-302. [PubMed]
- Zasadny KR, Wahl RL. Standardized uptake values of normal tissues at PET with 2-[fluorine-18]-fluoro-2-deoxy-D-glucose: variations with body weight and a method for correction. Radiology 1993;189:847-50. [Crossref] [PubMed]
- Andreoli A, Scalzo G, Masala S, Tarantino U, Guglielmi G. Body composition assessment by dual-energy X-ray absorptiometry (DXA). Radiol Med 2009;114:286-300. [Crossref] [PubMed]
- Saag KG, Petersen J, Brandi ML, Karaplis AC, Lorentzon M, Thomas T, Maddox J, Fan M, Meisner PD, Grauer A. Romosozumab or Alendronate for Fracture Prevention in Women with Osteoporosis. N Engl J Med 2017;377:1417-27. [Crossref] [PubMed]
- Hochberg MC, Ross PD, Black D, Cummings SR, Genant HK, Nevitt MC, Barrett-Connor E, Musliner T, Thompson D. Larger increases in bone mineral density during alendronate therapy are associated with a lower risk of new vertebral fractures in women with postmenopausal osteoporosis. Fracture Intervention Trial Research Group. Arthritis Rheum 1999;42:1246-54. [Crossref] [PubMed]
- Liao S, Lan X, Cao G, Yuan H, Zhang Y. Prognostic predictive value of total lesion glycolysis from 18F-FDG PET/CT in post-surgical patients with epithelial ovarian cancer. Clin Nucl Med 2013;38:715-20. [Crossref] [PubMed]
- Kim TM, Paeng JC, Chun IK, Keam B, Jeon YK, Lee SH, Kim DW, Lee DS, Kim CW, Chung JK, Kim IH, Heo DS. Total lesion glycolysis in positron emission tomography is a better predictor of outcome than the International Prognostic Index for patients with diffuse large B cell lymphoma. Cancer 2013;119:1195-202. [Crossref] [PubMed]
- Jiang C, Teng Y, Zheng Z, Zhou Z, Xu J. Value of total lesion glycolysis and cell-of-origin subtypes for prognostic stratification of diffuse large B-cell lymphoma patients. Quant Imaging Med Surg 2021;11:2509-20. [Crossref] [PubMed]