Prediction of intracranial hemorrhage after internal carotid artery stenting in patients with symptomatic severe carotid stenosis by computed tomography perfusion
Introduction
Carotid artery stenting (CAS) has become an alternative to carotid endarterectomy for the treatment of carotid artery disease (1). Cerebral hyperperfusion syndrome (CHS) is an uncommon but critical complication after CAS, for which hyperperfusion-induced intracranial hemorrhage (ICH) is the most severe outcome if it is not managed properly and swiftly (2,3). The incidence of postoperative ICH following CAS is about 0.36–6.67%, and the mortality rate of ICH is about 75% (4,5). Although the incidence of postoperative ICH is low, given its detrimental consequences, it is an issue that needs to be urgently addressed in clinical practice. Thus, the accurate preoperative prediction of ICH before CAS is essential to develop interventional strategies to reduce morbidity.
Several imaging techniques have been used to investigate ICH and CHS, including transcranial Doppler, magnetic resonance imaging (MRI), positron emission tomography (PET), single-photon emission computed tomography (SPECT), and computed tomography perfusion (CTP) (6-10). Oxygen PET and SPECT are the gold-standard examinations for brain perfusion assessment; however, they cannot be performed frequently in clinical practice, as they require special equipment, expensive drugs, and the use of invasive examination methods (11). Conversely, CTP is a non-invasive and readily available method, which can be used to evaluate cerebral hemodynamics, as it provides relatively accurate and quantitative perfusion parameters, including the cerebral blood flow (CBF), cerebral blood volume (CBV), mean transit time (MTT), and time to peak (TTP). It has been widely used in clinical practice to measure brain perfusion (12,13).
Previous studies have mainly focused on predicting the risk factors for CHS; however, data on the predictors of risk for ICH are limited. Thus, this study sought to explore whether the occurrence of ICH is related to preoperative CTP parameters and the compensation of the Willis’ circle to guide interventional treatment and reduce the possible adverse consequences of ICH.
Methods
Patients
In this retrospective study, the data of 183 patients with symptomatic severe carotid artery stenosis diagnosed by digital subtraction angiography (DSA) who received CAS at the Department of Neurology, Nanfang Hospital of Southern Medical University from June 2019 to June 2022 were collected. The patient inclusion criteria were as follows: (I) had experienced a transient ischemic attack or stroke 180 days before the surgery; (II) a diagnosis of severe stenosis (70–99%) of the internal carotid artery (ICA) by DSA; (III) had received unilateral internal CAS; and (IV) had undergone a CTP examination before surgery. The patient exclusion criteria were as follows: (I) had undergone a CTP examination before surgery; (II) non-internal CAS, such as vertebrobasilar artery stenting, or middle cerebral artery stenting; and/or (III) a simultaneous bilateral stent insertion. In total, 87 of 183 patients were included in the study (Figure 1). The study was conducted in accordance with the Declaration of Helsinki (as revised in 2013) with the approval of the Ethics Committee of the Nanfang Hospital of Southern Medical University (No. NFEC-2022-368), and informed consent was provided by all the patients.
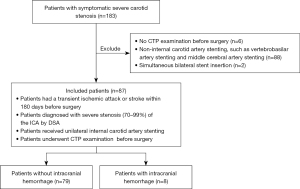
Operation methods
All the patients were pretreated with aspirin (100 mg/day), and clopidogrel (75 mg/day) for at least 3 days before the procedure. The treatment was performed under local anesthesia. The femoral artery was punctured using the Seldinger technique, and a bolus of heparin was administered for systemic anticoagulation during the procedure. After the guiding catheter had been inserted into the common carotid artery of the target side, a filter wire was navigated carefully through the ICA stenosis and placed at the end of the stenosis. Next, a balloon (Sterling 4*30 mm) was delivered along the filter wire to the stenosis position for pre-dilation. If a patient developed disordered consciousness, blood flow was restored as soon as possible, and after the balloon dilation, blood pressure was controlled at 70–80% of the preoperative blood pressure. Along the guiding micro-wire, the carotid stent (Wallstent 9*50 mm; Boston Scientific, Marlborough, MA, USA) was delivered to the site of stenosis, and the stent was released after it had been adjusted to cover the narrow segment completely. Afterwards, postprocedural angiography was performed to confirm the position of the stent. The residual stenosis condition was also examined to evaluate whether post-dilation was necessary. Finally, the filter wire was removed, and the arterial sheath was closed using Angio-Seal (Terumo, Tokyo, Japan). The patients experienced no discomfort during the operation and were returned to the neurosurgical intensive care unit where their vital signs and neurological functions were strictly monitored. After the procedure, each patient’s systolic blood pressure was controlled below 140 mmHg and their diastolic blood pressure was controlled below 90 mmHg, and all of the patients continued to take aspirin (100 mg/day) and clopidogrel (75 mg/day).
CTP study
Cerebral CTP and the imaging analysis were performed using a 256-sliced spiral CT (iCT 256; Philips, Best, The Netherlands). Iopromine (45 mL, 350 mg/mL) and normal saline (30 mL) were injected via the anterior vein of the right elbow through a high-pressure syringe at a rate of 4 mL/s. The dynamic perfusion scanning was performed with the following scanning parameters: tube voltage: 80 kV, tube current: 100 mA, layer thickness: 5 mm, layer spacing: 5 mm, and scanning: 30 stages, 16 layers per stage. After the CTP scan, a computed tomography angiography (CTA) scan was performed to observe the intracranial vessels with an additional 20 mL of contrast agent (bolus 2.5 mL/s). A total of 480 brain perfusion images were obtained. The raw images were independently analyzed by 2 senior neuroradiologists in a software package (Philips IntelliSpace Portal; https://www.philips.com.au/healthcare/product/HC881103/intellispace-portal-11). The ICA contralateral to the stenting was selected as the input artery, and the superior sagittal sinus as the output vein. The regions of interest (ROIs) were symmetrically placed at the bilateral regions of the middle cerebral artery territory, taking care to exclude hemorrhagic foci, infarction foci, calcification foci, blood vessels, and so on. The perfusion parameters were measured to obtain the CBF, CBV, MTT, and TTP values, and the ratio of the ipsilateral territory to the contralateral territory was calculated as the relative value (i.e., the rCBF, rCBV, rMTT, and rTTP).
Imaging evaluation
ICH was defined as the lesion side of the cerebral parenchymal hemorrhage, ventricular hemorrhage, or subarachnoid hemorrhage after operation, and it was evaluated by postoperative head CT. All the patients underwent a postoperative CT scan, and the patients with asymptomatic ICH underwent an additional cranial MRI scan. Preoperative and postoperative DSA was used to assess the degree of vascular stenosis, preoperative CTP imaging was performed to assess hypoperfusion, and preoperative CTA was performed to evaluate the Willis’ circle.
Willis’ circle evaluation
The evaluation of the Willis’ circle was based on the classification criteria reported by Hoksbergen et al. (14). The classification included the following 4 types of Willis’ circle: type I: good compensation of both anterior and posterior circulation; type II: good compensation of anterior circulation and poor compensation of posterior circulation; type III: poor compensation of anterior circulation and good compensation of posterior circulation; and type IV: poor compensation of both anterior and posterior circulation. In reference to the study of Zhang et al. (15), a vessel diameter <0.5 mm or <1/2 of the contralateral vessel was considered underdeveloped. In our study, well-developed anterior circulation was defined as both bilateral A1 and anterior communicating arteries (ACoAs), and was otherwise defined as underdeveloped anterior circulation. Absent anterior circulation was defined as either bilateral A1 or missing ACoAs. The definition of posterior circulation was the same as anterior circulation. A well-developed Willis’ circle had good compensation, whereas an absent and underdeveloped Willis’ circle had poor compensation.
Statistical analysis
The data were analyzed using the software SPSS 25.0 (IBM Corp., Armonk, NY, USA). The metrological data are presented as the median and quartiles [M (P25, P75)]. The Mann-Whitney U test was used for comparisons between 2 groups. The chi-squared test was used to compare the counting data. A receiver operating characteristic (ROC) curve analysis was conducted to examine the diagnostic value of the CTP parameters for ICH after CAS. A P value <0.05 was considered statistically significant.
Results
Incidence of ICH
Of the 87 patients included in the study, 71 were male and 16 were female. The patients had an average age of 64.40±9.99 years (range, 35–85 years). In total, 80 (92%) patients had carotid artery stenosis caused by atherosclerosis. All patients received unilateral stenting treatment, and stent placement was successful in all 87 cases (success rate: 100%). Of the 87 patients, 8 (9.2%) had postoperative ICH. The baseline characteristics of the patients with and without ICH are shown in Table 1.
Table 1
Variable | Non-ICH (n=79) | ICH (n=8) | P value |
---|---|---|---|
Sex | 1.000 | ||
Male | 64 | 7 | |
Female | 15 | 1 | |
Age | 0.415 | ||
≥60 years | 56 | 4 | |
<60 years | 23 | 4 | |
Hypertension | 0.793 | ||
Yes | 53 | 5 | |
No | 26 | 3 | |
Diabetes | 0.989 | ||
Yes | 24 | 3 | |
No | 55 | 5 | |
Smoking | 0.684 | ||
Yes | 19 | 3 | |
No | 60 | 5 | |
Stenosis degree | 0.021 | ||
70–80% | 25 | 0 | |
80–90% | 18 | 1 | |
≥90% | 36 | 7 | |
Stenting side | 0.452 | ||
Left | 46 | 3 | |
Right | 33 | 5 | |
Preoperative SBP [M (P25, P75)] | 138 (129.5, 150.5) | 139.5 (123.5, 144.5) | 0.735 |
Preoperative DBP [M (P25, P75)] | 79 (75, 86) | 81 (75.5, 85) | 0.953 |
Postoperative SBP [M (P25, P75)] | 126 (116, 138) | 125 (120.25, 134) | 0.936 |
Postoperative DBP [M (P25, P75)] | 73 (65, 80) | 77 (67.5, 81.5) | 0.347 |
ICH, intracranial hemorrhage; SBP, systolic blood pressure, DBP, diastolic blood pressure.
Of the 79 patients without ICH, 64 were male and 15 were female. Among these cases, 56 were aged ≥60 years and 23 were aged <60 years. The degree of stenosis was 70–80% (31.6%) in 25 patients, 80–90% (22.8%) in 18 patients, and ≥90% (45.6%) in 36 patients. In total, 73 (92.4%) patients had carotid artery stenosis caused by atherosclerosis.
Of the 8 patients with ICH, 7 were male and 1 was female. Among these 8 patients, 4 were aged ≥60 years and 4 were aged <60 years. The degree of stenosis was 80–90% (12.5%) in 1 patient, and ≥90% (87.5%) in 7 patients. In total, 7 (87.5%) patients had carotid artery stenosis caused by atherosclerosis, and 2 (25%) patients presented with asymptomatic ICH. Additionally, 4 patients had cerebral parenchymal hemorrhage, 1 patient had subarachnoid hemorrhage, and 3 patients had cerebral parenchymal and subarachnoid hemorrhages. The hemorrhagic foci was 1–6 cm in diameter in the ICH group. These patients developed ICH within 1–3 days of the procedure.
CTP examination results
All of the patients underwent CTP before operation. The absolute and relative CTP values, including the CBF, CBV, MTT, TTP, rCBF, rCBV, rMTT, and rTTP, were collected for the statistical analysis, and the results showed that the CBF, MTT, rCBF, rMTT, and rTTP differed significantly between the ICH group and non-ICH group (Table 2). The ROC curve analysis showed that in the prediction of ICH, the CBF, MTT, rCBF, rMTT, and rTTP had AUCs of 0.741 [95% confidence interval (CI): 0.529–0.954], 0.735 (95% CI: 0.498–0.972), 0.794 (95% CI: 0.615–0.972), 0.808 (95% CI: 0.602–1.000), and 0.797 (95% CI: 0.590–1.000), respectively. The CTP parameter with the maximal AUC for ICH was the rMTT, of which the optimal cut-off point for ICH was 1.88, the sensitivity was 62.5%, and the specificity was 96.2% (Figure 2). This indicated that patients with an rMTT >1.88 were more likely to develop ICH. Imaging from a patient is shown in Figure 3.
Table 2
Parameter | Non-ICH (n=79) | ICH (n=8) | Z | P value |
---|---|---|---|---|
CBF [M (P25, P75)] | 35.43 (30.25, 42.27) | 27.07 (23.88, 34.43) | −2.240 | 0.025 |
CBV [M (P25, P75)] | 4.22 (3.84, 4.76) | 4.59 (4.00, 4.64) | −0.455 | 0.649 |
MTT [M (P25, P75)] | 7.57 (6.23, 9.25) | 11.36 (7.98, 13.23) | −2.181 | 0.029 |
TTP [M (P25, P75)] | 21.38 (18.21, 23.61) | 22.50 (20.10, 25.20) | −0.823 | 0.411 |
rCBF [M (P25, P75)] | 0.88 (0.74, 0.98) | 0.62 (0.53, 0.81) | −2.725 | 0.006 |
rCBV [M (P25, P75)] | 1.04 (0.98, 1.14) | 1.17 (1.04, 1.29) | −1.535 | 0.125 |
rMTT [M (P25, P75)] | 1.22 (1.03, 1.51) | 1.93 (1.46, 2.32) | −2.857 | 0.004 |
rTTP [M (P25, P75)] | 1.06 (1.01, 1.13) | 1.23 (1.14, 1.28) | −2.763 | 0.006 |
CTP, computed tomography perfusion; ICH, intracranial hemorrhage; CBF, cerebral blood flow; CBV, cerebral blood volume; MTT, mean transit time; TTP, time to peak; rCBF relative CBF; rCBV, relative CBV; rMTT, relative MTT; rTTP, relative TTP.
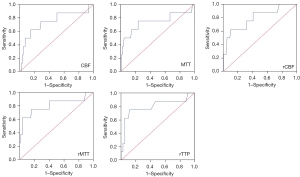
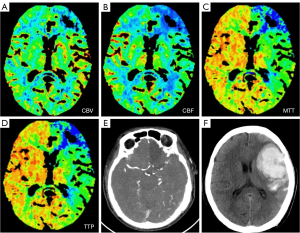
Willis’ circle evaluation
Based on the preoperative CTA results, the patients were classified based on the 4 types of Willis’ circle. Table 3 shows the relationship between ICH and the type of Willis’ circle. Of the 79 patients without ICH, 10 had good compensation of both anterior and posterior circulation, 39 had poor compensation of either anterior or posterior circulation, and 30 had poor compensation of both anterior and posterior circulation. Of the 8 patients with ICH, 5 had poor compensation of either anterior or posterior circulation, and 3 had poor compensation of both anterior and posterior circulation. There was no statistically significant difference between the 2 groups (P=0.713), which indicated that the occurrence of ICH after surgery was not related to the type of Willis’ circle.
Table 3
Variable | Non-ICH (n=79) | ICH (n=8) | P value |
---|---|---|---|
Willis’ circle type | 0.713 | ||
Type I | 10 | 0 | |
Type II | 29 | 3 | |
Type III | 10 | 2 | |
Type IV | 30 | 3 |
ICH, intracranial hemorrhage.
Discussion
Due to the high disability and mortality rate of ICH after CAS, it is essential that the risk factors for ICH after CAS in patients with symptomatic severe carotid stenosis be explored. In our study, the incidence of ICH was 9.2%. We found that the CBF, MTT, rCBF, rMTT, and rTTP were risk factors in predicting ICH following CAS, and the rMTT was the most effective diagnostic parameter. Further, when the rMTT was >1.88, the patients with symptomatic severe carotid stenosis had a higher risk of developing ICH after CAS.
A meta-analysis of 28,956 participants who had undergone CAS reported that the incidence of ICH after carotid intervention ranged from 0% to 4.44% in studies with <100 participants, 0% to 2.21% in studies with 101–1,000 participants, and 0.09% to 0.6% in studies with >1,001 participants (16). Notably, the incidence of ICH was higher in studies with small sample sizes than in studies with large sample sizes. In our study, the incidence of ICH was 9.2%, which was significantly higher than that reported in previous studies. We attribute this to the strict selection criteria of our study, under which only patients with symptomatic severe carotid stenosis were included. In addition, stenting may be safer in patients with mild carotid stenosis, and the occurrence of ICH after surgery may be reduced.
Previous studies have reported several risk factors for ICH, including severe carotid artery stenosis (≥90%), poor compensation of collateral circulation, and failure to control postoperative blood pressure (17,18). Russell et al. (19) found that severe stenosis of the ICA may be a risk factor in the development of ICH. In our investigation, the surgical sites of 7 ICH patients were nearly totally occluded (≥90%), demonstrating that those with severe carotid artery stenosis were more likely to develop ICH. For the patients in this study, 92% of the carotid artery stenosis was due to atherosclerosis, and there was no significant difference between the ICH and non-ICH groups, which suggested that the occurrence of ICH was not related to the different etiologies of carotid stenosis. The results of our study also revealed that there was no significant difference in the hypertension incidence rates between the ICH and non-ICH groups. Additionally, there were no significant differences in the preoperative or postoperative blood pressure between the 2 groups. Thus, hypertension was not linked to the development of ICH, which may be explained by the strict control of blood pressure during the perioperative period. We also found that diabetes was not associated with ICH, which is consistent the findings of some previous studies (15,17); however, it should be noted that some other studies have found that diabetes is a risk factor for ICH after CAS (20,21). Thus, during the CAS treatment period, patients with a history of diabetes should be closely monitored.
A few studies have predicted postoperative ICH using preoperative CTP parameter features, but inconsistent conclusions have been reached. For example, Zhang et al. (15) reported that patients with a preoperative TTP index >1.22 had a higher risk of developing ICH after CAS, whereas Austein et al. (10) found that none of the CTP parameters were a predictor of ICH, and that the Alberta Stroke Program Early CT score and initial CTP core volume were more reliable parameters for assessing the risk of ICH. In this study, we found that the CBF, MTT, rCBF, rMTT, and rTTP differed significantly between the ICH group and non-ICH group. Compared to the group without ICH, the CBF and rCBF values declined more pronouncedly, and the MTT, rMTT, and rTTP values were significantly prolonged in the ICH group. There were no significant differences in the CBV and rCBV between the 2 groups; however, an increasing trend was observed in the ICH group. According to Waaijer et al. (22), a decrease in the CBF, combined with a slight increase in the CBV and an obvious prolongation in the MTT indicate vasodilation and an impairment of the autoregulation mechanism. Even if normal blood flow is restored after successful stenting, dilated vessels cannot constrict properly due to the impairment of the autoregulation mechanism, which may be a potential risk factor for hyperperfusion and increase the risk of ICH.
We also found that the rMTT was the most accurate parameter for predicting ICH after CAS, and the risk of ICH increased when the preoperative rMTT was >1.88. Tseng et al. (23) reported that patients with a prolonged absolute difference in mean transit time (dMTT) of more than 3 seconds had a higher risk of developing CHS after CAS. A prolongation of the dMTT may further signify a severe decrease in perfusion pressure, and Tseng et al. suggested that the MTT may be more sensitive than the CBF and CBV in detecting cerebral hemodynamic changes because the MTT is calculated using the CBF and CBV (i.e., MTT = CBV/CBF). As the CBF decreases and the CBV increases, the MTT increases significantly. Kikuchi et al. (24) showed that MTT images could be used to assess the extent of impaired cerebral perfusion reserve in patients with occlusive cerebrovascular disease by using dynamic susceptibility contrast-enhanced MRI. Thus, it is critical to detect preoperative CTP parameters, and patients with an rMTT >1.88 should be closely monitored for evidence of ICH after CAS.
Many studies have reported that patients with poor compensation in the Willis’ circle are prone to develop postoperative ICH; however, our results did not support this finding. In our study, there was no statistically significant difference between the ICH group and non-ICH group. The definitions adopted for good or poor collateral compensation have varied in different studies (15,25-27). For example, Katano et al. (27) classified excellent collateral circulation solely as the patency of the ACoAs with well-developed bilateral A1 segments, regardless of the posterior communicating arteries. However, our definition was stricter: good anterior and posterior circulation were both defined as complete collateral circulation. Even if the collateral circulation is partially compensated in patients with symptomatic severe ICA stenosis, ICH may occur due to an impairment in the autonomic mechanisms. In this study, 3 cases of ICH occurred in patients with good compensating anterior circulation and 2 cases occurred in patients with good compensating posterior circulation, which may be related to this mechanism. We also found that of the 87 patients, none of the 10 patients with good compensation of both the anterior and posterior circulation developed ICH after surgery. Thus, patients with complete compensation of the Willis’ circle appeared to be less likely to develop ICH after surgery.
Our study had several limitations. First, we used the ICA contralateral to the stenting as the input artery. If the reference artery was stenotic, it will affect the arterial input function, overestimating the absolute MTT and underestimating the absolute CBF (28,29). Second, the absolute values of the CTP parameters are subject to physiologic variations and post-processing steps (22). To address this limitation, we used relative values, eliminating the physiologic variations and the interpatient differences. Third, the sample size was too small, which may have caused a statistical bias. Finally, given the retrospective nature of the study, selection bias was likely. Large sample size studies at multiple centers need to be conducted to evaluate the risk factors for ICH in the future.
To date, many risk factors for CHS have been reported, but due to the low incidence of ICH, few studies have reported the risk factors for ICH after CAS, especially when the CTP technique is used. In our study, we explored the risk of ICH by CTP by analyzing the characteristics of the preoperative CTP parameters, the Willis’ circle type, and the patients’ clinical baseline data. Due to the small sample size, we obtained some negative results. In the future, we intend to expand the sample size and conduct a prospective study to further confirm the risk factors for ICH after CAS in patients with symptomatic severe carotid stenosis.
Conclusions
CTP can be used to predict ICH after CAS in patients with symptomatic severe carotid stenosis, and patients with a preoperative rMTT >1.88 should be closely monitored for evidence of ICH after CAS.
Acknowledgments
The authors would like to thank the Department of Medical Imaging Center, Nanfang Hospital for providing the CT and MRI images for this study.
Funding: This work was supported by the Scientific and Technological Innovation 2030—“New Generation of Artificial Intelligence” Major Project (No. 2020AAA0104102), the Research and Development of Common Diseases Diagnosis and Treatment System for Middle-aged and Elderly People Based on Artificial Intelligence (No. 202103000037), and the Program for Changjiang Scholars and Innovative Research Team in University (No. IRT_16R37).
Footnote
Conflicts of Interest: All the authors have completed the ICMJE uniform disclosure form (available at https://qims.amegroups.com/article/view/10.21037/qims-22-1148/coif). KD is an employee of the Philips Healthcare Company. The Hospital and the Company are in a collaborative relationship. The other authors have no conflicts of interest to declare.
Ethical Statement: The authors are accountable for all aspects of the work in ensuring that questions related to the accuracy or integrity of any part of the work are appropriately investigated and resolved. The study was conducted in accordance with the Declaration of Helsinki (as revised in 2013). This study was approved by the Ethics Committee of Nanfang Hospital of Southern Medical University (No. NFEC-2022-368). Informed consent was provided by all the patients.
Open Access Statement: This is an Open Access article distributed in accordance with the Creative Commons Attribution-NonCommercial-NoDerivs 4.0 International License (CC BY-NC-ND 4.0), which permits the non-commercial replication and distribution of the article with the strict proviso that no changes or edits are made and the original work is properly cited (including links to both the formal publication through the relevant DOI and the license). See: https://creativecommons.org/licenses/by-nc-nd/4.0/.
References
- Abou-Chebl A, Yadav JS, Reginelli JP, Bajzer C, Bhatt D, Krieger DW. Intracranial hemorrhage and hyperperfusion syndrome following carotid artery stenting: risk factors, prevention, and treatment. J Am Coll Cardiol 2004;43:1596-601. [Crossref] [PubMed]
- Nakagawa I, Park H, Kotsugi M, Myouchin K, Takeshima Y, Matsuda R, Yamada S, Park YS, Nakase H. Hypocapnia Induced by Hyperventilation with Indocyanine Green Kinetics Detects the Effect of Staged Carotid Angioplasty to Avoid Hyperperfusion in Patients with Impaired Cerebral Hemodynamic Reserve. Transl Stroke Res 2022;13:77-87. [Crossref] [PubMed]
- Hussain MA, Alali AS, Mamdani M, Tu JV, Saposnik G, Salata K, Nathens AB, de Mestral C, Bhatt DL, Verma S, Al-Omran M. Risk of intracranial hemorrhage after carotid artery stenting versus endarterectomy: a population-based study. J Neurosurg 2018;129:1522-9. [Crossref] [PubMed]
- Kang HS, Han MH, Kwon OK, Kwon BJ, Kim SH, Oh CW. Intracranial hemorrhage after carotid angioplasty: a pooled analysis. J Endovasc Ther 2007;14:77-85. [Crossref] [PubMed]
- Zhou Y, Zheng C, Chen L, Lin Y. Fatal intracranial hemorrhage after carotid artery stenting: Three case reports and a literature review. J Int Med Res 2020;48:300060520937576. [Crossref] [PubMed]
- Orion D, Yavne Y, Peretz S, Givaty G. Diagnosing Hyperperfusion Syndrome: CT Perfusion or Transcranial Doppler? Isr Med Assoc J 2015;17:656-8. [PubMed]
- Wan CC, Chen DY, Tseng YC, Yan FX, Lee KY, Chiang CH, Chen CJ. Fluid-attenuated inversion recovery vascular hyperintensities in predicting cerebral hyperperfusion after intracranial arterial stenting. Neuroradiology 2017;59:791-6. [Crossref] [PubMed]
- Lin T, Lai Z, Zuo Z, Lyu Y, Feng F, You H, Hou B, Qu J, Wu B, Liu C. ASL perfusion features and type of circle of Willis as imaging markers for cerebral hyperperfusion after carotid revascularization: a preliminary study. Eur Radiol 2019;29:2651-8. [Crossref] [PubMed]
- Nishimoto T, Oka F, Okazaki K, Ishihara H. Relationship between cerebral hyperperfusion syndrome and the immediate change of cerebral blood flow after carotid artery stenting evaluated by single-photon emission computed tomography. Neuroradiology 2022;64:1157-64. [Crossref] [PubMed]
- Austein F, Fischer AC, Fiehler J, Jansen O, Lindner T, Gellißen S. Value of Perfusion CT in the Prediction of Intracerebral Hemorrhage after Endovascular Treatment. Stroke Res Treat 2021;2021:9933015. [Crossref] [PubMed]
- Zhang J, Xia C, Liu Y, Qian W, Peng W, Liu K, Li L, Zhao F, Li Z. Comparative study of MR mTI-ASL and DSC-PWI in evaluating cerebral hemodynamics of patients with Moyamoya disease. Medicine (Baltimore) 2018;97:e12768. [Crossref] [PubMed]
- Wang R, Yu S, Alger JR, Zuo Z, Chen J, Wang R, An J, Wang B, Zhao J, Xue R, Wang DJ. Multi-delay arterial spin labeling perfusion MRI in moyamoya disease--comparison with CT perfusion imaging. Eur Radiol 2014;24:1135-44. [Crossref] [PubMed]
- Yoshie T, Ueda T, Takada T, Nogoshi S, Fukano T, Hasegawa Y. Prediction of cerebral hyperperfusion syndrome after carotid artery stenting by CT perfusion imaging with acetazolamide challenge. Neuroradiology 2016;58:253-9. [Crossref] [PubMed]
- Hoksbergen AW, Fülesdi B, Legemate DA, Csiba L. Collateral configuration of the circle of Willis: transcranial color-coded duplex ultrasonography and comparison with postmortem anatomy. Stroke 2000;31:1346-51. [Crossref] [PubMed]
- Zhang L, Dai D, Li Z, Duan G, Zhang YW, Yang P, Huang Q, Xu Y, Hong B, Liu J. Risk factors for hyperperfusion-induced intracranial hemorrhage after carotid artery stenting in patients with symptomatic severe carotid stenosis evaluation. J Neurointerv Surg 2019;11:474-8. [Crossref] [PubMed]
- Abreu P, Nogueira J, Rodrigues FB, Nascimento A, Carvalho M, Marreiros A, Nzwalo H. Intracerebral hemorrhage as a manifestation of cerebral hyperperfusion syndrome after carotid revascularization: systematic review and meta-analysis. Acta Neurochir (Wien) 2017;159:2089-97. [Crossref] [PubMed]
- Xu S, Wu P, Zhang T, Ji Z, Wang C, Shi H. Prevalence and Clinical Predictors of Intracranial Hemorrhage Following Carotid Artery Stenting for Symptomatic Severe Carotid Stenosis. World Neurosurg 2021;155:e353-61. [Crossref] [PubMed]
- Ogasawara K, Sakai N, Kuroiwa T, Hosoda K, Iihara K, Toyoda K, Sakai C, Nagata I, Ogawa AJapanese Society for Treatment at Neck in Cerebrovascular Disease Study Group. Intracranial hemorrhage associated with cerebral hyperperfusion syndrome following carotid endarterectomy and carotid artery stenting: retrospective review of 4494 patients. J Neurosurg 2007;107:1130-6. [Crossref] [PubMed]
- Russell DA, Gough MJ. Intracerebral haemorrhage following carotid endarterectomy. Eur J Vasc Endovasc Surg 2004;28:115-23. [Crossref] [PubMed]
- Donnelly R, Emslie-Smith AM, Gardner ID, Morris AD. ABC of arterial and venous disease: vascular complications of diabetes. BMJ 2000;320:1062-6. [Crossref] [PubMed]
- Grunwald IQ, Politi M, Reith W, Krick C, Karp K, Zimmer A, Struffert T, Roth C, Kühn AL, Haass A, Papanagiotou P. Hyperperfusion syndrome after carotid stent angioplasty. Neuroradiology 2009;51:169-74. [Crossref] [PubMed]
- Waaijer A, van Leeuwen MS, van Osch MJ, van der Worp BH, Moll FL, Lo RT, Mali WP, Prokop M. Changes in cerebral perfusion after revascularization of symptomatic carotid artery stenosis: CT measurement. Radiology 2007;245:541-8. [Crossref] [PubMed]
- Tseng YC, Hsu HL, Lee TH, Hsieh IC, Chen CJ. Prediction of cerebral hyperperfusion syndrome after carotid stenting: a cerebral perfusion computed tomography study. J Comput Assist Tomogr 2009;33:540-5. [Crossref] [PubMed]
- Kikuchi K, Murase K, Miki H, Yasuhara Y, Sugawara Y, Mochizuki T, Ikezoe J, Ohue S. Quantitative evaluation of mean transit times obtained with dynamic susceptibility contrast-enhanced MR imaging and with (133)Xe SPECT in occlusive cerebrovascular disease. AJR Am J Roentgenol 2002;179:229-35. [Crossref] [PubMed]
- Mo D, Luo G, Wang B, Ma N, Gao F, Sun X, Xu X, Miao Z. Staged carotid artery angioplasty and stenting for patients with high-grade carotid stenosis with high risk of developing hyperperfusion injury: a retrospective analysis of 44 cases. Stroke Vasc Neurol 2016;1:147-53. [Crossref] [PubMed]
- Lau AY, Wong EH, Wong A, Mok VC, Leung TW, Wong KS. Significance of good collateral compensation in symptomatic intracranial atherosclerosis. Cerebrovasc Dis 2012;33:517-24. [Crossref] [PubMed]
- Katano H, Mase M, Sakurai K, Miyachi S, Yamada K. Revaluation of collateral pathways as escape routes from hyperemia/hyperperfusion following surgical treatment for carotid stenosis. Acta Neurochir (Wien) 2012;154:2139-48; discussion 2148-9. [Crossref] [PubMed]
- Yamada K, Wu O, Gonzalez RG, Bakker D, Østergaard L, Copen WA, Weisskoff RM, Rosen BR, Yagi K, Nishimura T, Sorensen AG. Magnetic resonance perfusion-weighted imaging of acute cerebral infarction: effect of the calculation methods and underlying vasculopathy. Stroke 2002;33:87-94. [Crossref] [PubMed]
- Calamante F, Gadian DG, Connelly A. Quantification of perfusion using bolus tracking magnetic resonance imaging in stroke: assumptions, limitations, and potential implications for clinical use. Stroke 2002;33:1146-51. [Crossref] [PubMed]