Well-controlled versus poorly controlled diabetes in patients with obesity: differences in MRI-evaluated pancreatic fat content
Introduction
Obesity and diabetes are 2 of the most prevalent health problems associated with severe morbidity and increased mortality (1). Individuals with obesity have a significantly higher risk of type 2 diabetes (T2D) than those with a normal body mass index (BMI) (2,3). Most patients with T2D have abnormal adiposity characterized by visceral obesity and ectopic fat deposition (4).
Poor glycemic control among patients with T2D constitutes a major public health issue and is a leading risk factor for diabetic complications (5). Many factors, including high BMI, the duration of diabetes, age, gender, and lifestyle, contribute to poor glycemic control (6,7). Glycemic control remains the primary therapeutic goal in preventing target organ damage and other complications caused by diabetes (8). For obese patients with poorly controlled T2D, additional interventions, such as bariatric surgery, may be required to achieve optimal glycemic control (9).
Excessive visceral adipose tissue (VAT) and increased ectopic fat deposits contribute to systemic inflammation, metabolic dysfunction, and insulin resistance (10). Therefore, quantifying the distribution of adipose tissue in various body parts is crucial for studying diabetes and metabolic syndrome (11).
Magnetic resonance imaging (MRI) and computed tomography (CT) are the gold standard methods for dynamically assessing body composition (12). Moreover, MRI can accurately quantify the fat content in the pancreas and liver (13-15). Several MRI studies have reported that individuals with high VAT and ectopic fat deposits are at a significantly increased risk for T2D and metabolic syndrome (16,17).
In this context, we evaluated the distribution of adipose tissue in obese patients with and without impaired glucose metabolism. Furthermore, we aimed to determine the associations between VAT, hepatic proton-density fat fraction (PDFF), and pancreatic PDFF with poor glycemic control in obese patients with T2D and to evaluate the metabolic effect of bariatric surgery in obese patients with poorly controlled diabetes. We present the following article in accordance with the STROBE reporting checklist (available at https://qims.amegroups.com/article/view/10.21037/qims-22-1083/rc).
Methods
Study design and population
This is a retrospective cross-sectional study. The clinical records of 463 consecutive obese patients who underwent abdominal MRI scans during bariatric surgery between July 2019 to March 2021 at The First Affiliated Hospital of Jinan University were reviewed. After the exclusion of 278 patients with incomplete imaging data [no iterative decomposition of water and fat with echo asymmetry and least-squares estimation quantitation (IDEAL-IQ) sequence], 185 obese patients (all >18 years) with complete imaging data were included. Diagnoses of normal glucose tolerance (NGT), prediabetes, and T2D were made based on the criteria of the American Diabetes Association (18). The diabetics included in the present study included new-onset patients without prior treatment and those treated medically for 2–4 years. For medically treated patients, those with a glycated hemoglobin (HbA1c) level equal to or less than 7% were regarded as patients with well-controlled T2D, whereas others with an HbA1c level of more than 7% were considered patients with poorly controlled T2D (19,20). All participants were Chinese and of Han ethnicity. Participants aged over 50 years or with chronic or acute viral hepatitis (hepatitis A, B, or C), pancreatic disease, insufficient image quality or incomplete clinical datasets, and BMI over 50 kg/m2 were excluded from this study. According to the above criteria, 34 obese patients (18.4%) were excluded because of insufficient image quality and incomplete clinical datasets. Thus, the study included 28 individuals with new-onset T2D, 17 with well-controlled T2D, 32 with poorly controlled T2D, 20 with prediabetes, and 54 with NGT (Figure 1). Moreover, among patients with poorly controlled T2D, 18 patients were evaluated before and 12 months after bariatric surgery (laparoscopic sleeve gastrectomy), and a further 18 age- and sex-matched non-obese healthy individuals (18 kg/m2 < BMI < 25 kg/m2) served as controls. The study was conducted in accordance with the Declaration of Helsinki (as revised in 2013). The study was approved by the Ethics Committee of the First Affiliated Hospital of Jinan University, and individual consent for this retrospective analysis was waived.
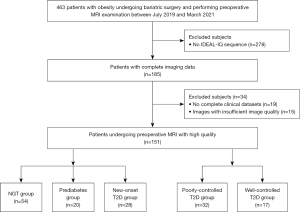
All participants underwent the following blood laboratory tests: HbA1c, fasting blood glucose (FBG), alanine aminotransferase (ALT), aspartate aminotransferase (AST), triglyceride (TG), low-density-lipoprotein cholesterol (LDL-c), high-density lipoprotein cholesterol (HDL-c), albumin (Alb), globulin (Gio), and C-reactive protein (CRP). All of these tests were performed in a standard manner. BMI was measured using the formula BMI = body weight (kg)/height2 (m2). All cases of obesity-related metabolic syndrome were confirmed by clinical or postoperative pathological examination.
MRI examination
All participants underwent MRI examinations using a 3.0T MRI scanner (Discovery MR750; GE Healthcare, Chicago, IL, USA). Both sequences were performed in a single breath hold (upon inspiration) to ensure optimal image quality. The MRI scan covered the diaphragm to the fourth lumbar vertebral body. Unenhanced fat-suppressed single-shot fast spin echo T2-weighted images (T2WI), gradient-recalled-echo T1-weighted images (T1WI) with in and opposed phase, and the IDEAL-IQ sequence were obtained. The IDEAL-IQ sequence had the following parameters: repetition time (TR) =15.6 ms, 6 echoes in each TR, first echo time (TE 1) =1.2–1.5 ms (increment: 1.23 ms, 6 echoes); flip angle, 8°; and slice thickness, 10 mm. The images were processed using the software provided by the manufacturer.
Anthropometric measurements
All MRI images were acquired from our institution’s Picture Archiving and Communication System (PACS). For each participant, 2 consecutive axial images at the level of the L3 lumbar vertebra were processed and then averaged. A trained radiologist (YD) analyzed the MRI images, and another radiologist (YL) double-checked the results. Using Image J 1.51 (National Institutes of Health, Bethesda, MD, USA), the subcutaneous adipose tissue (SAT; cm2) and the VAT (cm2) were demarcated using predetermined thresholds for the signal intensity, as previously described (21).
Measurement of hepatic PDFF and pancreatic PDFF
The IDEAL-IQ images were reviewed by a radiologist with more than 5 years of experience in abdominal imaging blinded to the patient’s clinical and biochemical data. The PDFF levels of the liver and pancreas were performed on the fat fraction map using the workstation (AW 4.4; GE Healthcare). Hepatic PDFF levels were calculated by placing 2 regions of interest (ROIs) in each of the left and right lobes with an ROI of approximately 40–50 mm2. An ROI of approximately 10–15 mm2 was placed in each of the head, body, and tail of the pancreas, and the PDFF of each ROI was measured. Then, the average PDFF levels were calculated for the liver and pancreas. All ROIs avoided major vessels, ducts, and collecting systems (Figure 2).
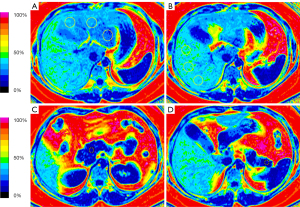
Statistical analysis
Descriptive data were reported as means with standard deviation (SD), medians with an interquartile range, or numbers with percentages. For categorical variables, a chi-square test was used. Continuous variables were tested for normality using the Shapiro-Wilk test and, when not normally distributed, nonparametric tests were performed.
In the initial univariate analysis, data between the NGT, prediabetes, and new-onset T2D groups were compared using one-way analysis of variance (ANOVA; parametric data) or the Kruskal-Wallis H test (nonparametric data) with post hoc Holm-Bonferroni correction for multiple comparisons. Comparisons between poorly controlled T2D and well-controlled T2D groups were evaluated by using the Student’s t-test (parametric data) and Mann-Whitney U test (nonparametric data), as appropriate.
Pancreatic tail PDFF, liver steatosis, Alb, and LDL-c distinguished poorly controlled T2D from well-controlled T2D based on the univariate analyses (all P<0.05). Therefore, in the next step, we used multivariate logistic regression analyses to evaluate which variables were independently associated with poorly controlled T2D.
Finally, comparisons before and after surgery were evaluated by using the paired t-test (parametric data) and the Wilcoxon signed-rank test (nonparametric data), as appropriate. An independent-samples t-test (parametric data) or Mann-Whitney U test (nonparametric data) was performed to compare continuous variables between the obese and control groups. The change after weight loss in patients with obesity for the continuous variable was calculated as the post-surgery value minus the pre-surgery value divided by the pre-surgery value, and the results were expressed as a percentage. The statistical analyses were performed using SPSS 26.0 (IBM Corp., Armonk, NY, USA). A P value (or a P value adjusted in the Bonferroni method) <0.05 was considered statistically significant.
Results
A total of 151 obese patients with or without impaired glucose metabolism were included (Figure 1). The mean age of the individuals was 30 years, and 71 were female. The mean BMI was 37.8 kg/m2.
Characteristics of the participants in the obese NGT, prediabetes, and new-onset T2D groups
Table 1 shows the characteristics of patients with obesity and NGT, prediabetes, and new-onset T2D. The BMI and CRP in the prediabetes group were significantly higher than those in the NGT group (Padjusted=0.046 and Padjusted<0.001, respectively). The proportions of patients with hyperlipidemia in the new-onset T2D group was significantly higher than that in the prediabetes (Padjusted=0.003) and NGT (Padjusted=0.01) groups.
Table 1
Variables | NGT (n=54) | Prediabetes (n=20) | New-onset T2D (n=28) | P value | Adjusted P value | ||
---|---|---|---|---|---|---|---|
Prediabetes vs. NGT | T2D vs. NGT | T2D vs. prediabetes | |||||
Age, years† | 31.3 (8.9) | 30.0 (7.8) | 29.0 (8.3) | 0.50 | |||
BMI, kg/m2 | 36.4 (31.7–41.0) | 39.7 (35.0–43.6) | 39.1 (35.3–43.3) | 0.012 | 0.046 | 0.07 | 1.0 |
Weight, kg† | 102.8 (21.9) | 115.4 (19.8) | 113.2 (21.7) | 0.03 | 0.08 | 0.12 | 1.0 |
Height, cm† | 168.6 (7.1) | 170.4 (7.4) | 169.3 (9.0) | 0.69 | |||
Female‡ | 26 (48.1) | 10 (50.0) | 13 (46.4) | 0.90 | |||
Liver steatosis‡ | 46 (85.2) | 20 (100.0) | 27 (96.4) | 0.08 | |||
Hypertension‡ | 3 (5.6) | 4 (20.0) | 4 (14.3) | 0.17 | |||
Hyperlipidemia‡ | 18 (33.3) | 4 (20.0) | 18 (64.3) | 0.003 | 0.39 | 0.01 | 0.003 |
Glu, mmol/L | 4.9 (4.7–5.4) | 5.9 (5.5–6.5) | 9.2 (7.2–13.5) | <0.001 | <0.001 | <0.001 | 0.01 |
HbA1c, % | 5.5 (5.1–5.7) | 6.5 (6.2–6.9) | 8.7 (7.1–10.6) | <0.001 | <0.001 | <0.001 | 0.023 |
CRP, mg/L | 4.2 (1.6–5.9) | 8.0 (4.8–12.7) | 4.7 (2.5–9.7) | <0.001 | <0.001 | 0.09 | 0.28 |
ALT, U/L | 34.5 (24.8–53.0) | 79.5 (41.3–145.3) | 67.0 (38.5–111.3) | <0.001 | 0.002 | 0.008 | 1.0 |
AST, U/L | 22.5 (18.0–36.0) | 51.4 (30.0–88.5) | 36.0 (23.0–55.0) | <0.001 | <0.001 | 0.009 | 0.58 |
Alb, U/L† | 43.2 (3.3) | 43.1 (2.9) | 43.3 (3.2) | 0.96 | |||
Gio, U/L† | 29.1 (3.3) | 30.1 (4.8) | 31.1 (4.1) | 0.08 | |||
TG, mmol/L | 1.6 (1.3–2.3) | 1.6 (1.4–4.1) | 2.6 (1.4–4.6) | 0.011 | 1.0 | 0.009 | 0.23 |
HDL-c, mmol/L | 1.0 (0.9–1.2) | 1.0 (0.8–1.1) | 1.0 (0.9–1.1) | 0.14 | |||
LDL-c, mmol/L | 3.0 (2.7–3.7) | 3.0 (2.6–3.9) | 3.2 (2.8–3.9) | 0.40 | |||
Hepatic PDFF, % | 10.5 (5.5–19.8) | 19.9 (14.2–25.0) | 18.2 (14.3–22.7) | <0.001 | 0.004 | 0.002 | 1.0 |
Pancreatic PDFF, % | 4.1 (2.7–5.8) | 6.0 (4.3–9.1) | 6.7 (5.5–10.1) | <0.001 | 0.005 | <0.001 | 0.63 |
Pancreatic head PDFF, % | 4.1 (2.9–7.3) | 7.8 (4.3–11.0) | 9.0 (7.6–11.6) | <0.001 | 0.009 | <0.001 | 0.2 |
Pancreatic body PDFF, % | 4.0 (2.3–5.7) | 5.9 (3.6–9.4) | 6.0 (4.0–11.7) | 0.001 | 0.019 | 0.004 | 1.0 |
Pancreatic tail PDFF, % | 3.1 (2.2–5.1) | 4.9 (3.4–7.5) | 4.7 (3.1–7.7) | 0.004 | 0.016 | 0.026 | 1.0 |
VAT, cm2† | 145 (64.8) | 193.7 (59.2) | 186.9 (80.4) | 0.006 | 0.023 | 0.03 | 1.0 |
SAT, cm2† | 260.9 (104.5) | 388.5 (99.2) | 330.7 (138.7) | 0.007 | 0.03 | 0.03 | 1.0 |
Unless otherwise specified, data are medians, with interquartile ranges in parentheses. †, continuous variables are presented as the mean (SD); ‡, data are numbers of individuals, with percentages in parentheses. All comparisons were performed with the one-way analysis of variance (for parametric data) or Kruskal-Wallis tests (for nonparametric data), both with post hoc Holm-Bonferroni corrections. T2D, type 2 diabetes; BMI, body mass index; NGT, normal glucose tolerance; Glu, glucose; HbA1c, glycated hemoglobin; CRP, C-reactive protein; ALT, alanine aminotransferase; AST, aspartate aminotransferase; Alb, albumin; Gio, globulin; TG, triglyceride; HDL-c, high-density lipoprotein cholesterol; LDL-c, low-density-lipoprotein cholesterol; PDFF, proton-density fat fraction; VAT, visceral adipose tissue; SAT, subcutaneous adipose tissue.
The visceral fat, hepatic fat content, and pancreatic fat content were higher in the prediabetes and new-onset T2D groups than in the NGT group
The VAT and hepatic PDFF were significantly higher in the new-onset T2D and prediabetes groups than in the NGT group (all Padjusted<0.05; Figure 3). The whole pancreas, pancreatic head, pancreatic body, and pancreatic tail PDFF in the new-onset T2D and prediabetes groups were significantly higher compared to the NGT group (all Padjusted<0.05; Table 1). However, there was no significant difference in VAT, hepatic PDFF, and all pancreatic PDFF between the new-onset T2D and prediabetic groups.
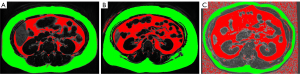
Clinical characteristics of the patients in the poorly controlled T2D and well-controlled T2D groups
The characteristics of patients in the well-controlled T2D group compared to those of the poorly controlled T2D group are shown in Table 2. The levels of Alb, TG, and LDL-c in the poorly controlled group were significantly higher than those in the well-controlled group (P=0.02, P=0.04, and P=0.002, respectively). In addition, the proportions of patients with liver steatosis were significantly higher in the poorly controlled group than in the well-controlled group (P=0.008).
Table 2
Variables | Poorly controlled T2D (n=32) | Well-controlled T2D (n=17) | P value |
---|---|---|---|
Age, years† | 39.6 (10.4) | 36.8 (9.6) | 0.22 |
BMI, kg/m2 | 34.9 (32.7–38.3) | 36.9 (32.7–43.1) | 0.33 |
Weight, kg | 99.0 (90.6–114.6) | 98.2 (84.5–122.5) | 0.99 |
Height, cm† | 169.4 (9.5) | 165.0 (10.2) | 0.14 |
Female‡ | 14 (43.8) | 8 (47.1) | 0.83 |
Liver steatosis‡ | 31 (96.9) | 12 (70.6) | 0.008 |
Hypertension‡ | 15 (46.9) | 7 (41.2) | 0.70 |
Hyperlipidemia‡ | 16 (50.0) | 8 (47.1) | 0.85 |
Medication history‡ | 0.77 | ||
OHA | 22 (68.8) | 11 (64.7) | |
OHA + insulin | 10 (31.3) | 6 (35.3) | |
Duration of diabetes, years | 3.0 (2.0–3.0) | 2.0 (2.0–3.0) | 0.38 |
Glu, mmol/L | 7.5 (6.6–10.2) | 5.8 (5.4–6.4) | <0.001 |
HbA1c, % | 7.3 (7.1–8.8) | 5.9 (5.7–6.4) | <0.001 |
CRP, mg/L | 4.5 (2.0–9.0) | 3.8 (2.3–6.8) | 0.65 |
ALT, U/L | 49.0 (26.5–82.0) | 40.1 (17.0–74.5) | 0.42 |
AST, U/L | 26.0 (20.0–46.0) | 30.0 (16.0–40.5) | 0.56 |
Alb, U/L† | 43.1 (3.4) | 40.6 (3.6) | 0.02 |
Gio, U/L | 30.4 (27.9–31.9) | 27.7 (27.1–30.6) | 0.09 |
TG, mmol/L | 2.8 (1.6–4.7) | 1.5 (1.2–2.4) | 0.04 |
HDL-c, mmol/L | 1.0 (0.9–1.2) | 0.9 (0.8–1.2) | 0.34 |
LDL-c, mmol/L† | 3.2 (0.8) | 2.4 (0.7) | 0.002 |
Hepatic PDFF, %† | 15.3 (7.5) | 13.6 (4.0) | 0.37 |
Pancreatic PDFF, % | 7.0 (5.8–9.0) | 6.2 (5.7–6.9) | 0.10 |
Pancreatic head PDFF, % | 8.4 (6.2–10.4) | 7.9 (7.6–8.7) | 0.64 |
Pancreatic body PDFF, % | 6.8 (5.4–8.6) | 6.8 (5.4–7.4) | 0.50 |
Pancreatic tail PDFF, % | 6.6 (5.1–9.8) | 4.7 (3.1–5.5) | 0.001 |
VAT, cm2† | 196.5 (73.5) | 198.7 (36.4) | 0.91 |
SAT, cm2 | 296.0 (208.8–472.7) | 327.8 (217.5–515.3) | 0.39 |
Unless otherwise specified, data are medians, with interquartile ranges in parentheses. †, continuous variables are presented as the mean (SD). ‡, data are numbers of individuals, with percentages in parentheses. All comparisons were performed with the independent-samples t-tests (for parametric data) or Mann-Whitney U test (for nonparametric data). T2D, type 2 diabetes; BMI, body mass index; OHA, oral hypoglycemic agent; Glu, glucose; HbA1c, glycated hemoglobin; CRP, C-reactive protein; ALT, alanine aminotransferase; AST, aspartate aminotransferase; Alb, albumin; Gio, globulin; TG, triglyceride; HDL-c, high-density lipoprotein cholesterol; LDL-c, low-density-lipoprotein cholesterol; PDFF, proton-density fat fraction; VAT, visceral adipose tissue; SAT, subcutaneous adipose tissue.
Pancreatic tail fat content was significantly higher in the poorly controlled T2D
The pancreatic tail PDFF in the poorly controlled group was significantly higher than that in the well-controlled group (P=0.001; Table 2). However, between groups, there was no statistically significant difference in the VAT, hepatic PDFF, whole pancreatic PDFF, pancreatic head PDFF, and pancreatic body PDFF.
Poorly controlled T2D was independently associated with pancreatic tail fat content
In the univariate logistic regression analysis, liver steatosis [odds ratio (OR) =12.92; 95% confidence interval (CI): 1.36–122.3; P=0.03], Alb (OR =1.25; 95% CI: 1.03–1.51; P=0.03), LDL-c (OR =3.93; 95% CI: 1.51–10.22; P=0.005), and pancreatic tail PDFF (OR =1.70; 95% CI: 1.13–2.54; P=0.009) were significantly associated with the presence of poorly controlled T2D.
In the multivariate analysis (Table 3), only pancreatic tail PDFF (OR =2.09; 95% CI: 1.11–3.94; P=0.022) was significantly associated with increased odds of poor glycemic control. In the receiver operating characteristic (ROC) curve analysis, the area under the curve (AUC) of pancreatic tail PDFF was 0.803 (95% CI: 0.682–0.925), and the optimal cutoff value was set at 5.9%, with a corresponding sensitivity of 62.5% and specificity of 100%.
Table 3
Predictors | Univariate logistic regression | Multivariate logistic regression | |||||
---|---|---|---|---|---|---|---|
OR | 95% CI | P value | OR | 95% CI | P value | ||
Liver steatosis | 12.92 | 1.36–122.3 | 0.03 | 3.20 | 0.28–37.17 | 0.35 | |
Alb | 1.25 | 1.03–1.51 | 0.03 | 1.27 | 0.94–1.71 | 0.11 | |
LDL-c | 3.93 | 1.51–10.22 | 0.005 | 2.39 | 0.82–6.94 | 0.11 | |
Pancreatic tail PDFF | 1.70 | 1.13–2.54 | 0.009 | 2.09 | 1.11–3.94 | 0.022 |
OR, odds ratio; CI, confidence interval; Alb, albumin; LDL-c, low-density-lipoprotein cholesterol; PDFF, proton-density fat fraction.
The metabolic effect of bariatric surgery in obese patients with poorly controlled diabetes
Table 4 summarizes the major aspects of the patients’ characteristics, including body weight, BMI, glycemic and lipid parameters, hepatic PDFF, and all pancreatic PDFF, in obese patients with poorly controlled T2D and in non-obese controls. Before surgery, the obese patients had significantly higher hepatic PDFF and all pancreatic PDFF compared to the non-obese controls (all P<0.01). Values of TG and LDL-c were significantly higher in the obese patients compared than in the non-obese controls (both P<0.01).
Table 4
Variables | Lean controls (n=18) | Obese surgical patient | P value | Change (%) from pre-surgery | ||||
---|---|---|---|---|---|---|---|---|
Pre-surgery (n=18) | Post-surgery (n=18) | Pre vs. Controls | Post vs. Controls | Pre vs. Post | ||||
Age, years† | 36.8 (6.5) | 36.9 (7.5) | – | 0.95 | – | – | – | |
Female‡ | 10 (55.6) | 9 (50.0) | – | 0.74 | – | – | – | |
Height, cm† | 168.4 (8.1) | 167.3 (5.5) | – | 0.95 | – | – | – | |
BMI, kg/m2 | 20.7 (19.4–22.7) | 39.3 (32.8–48.0) | 28.8 (26.1–31.2) | <0.001 | 0.01 | 0.002 | −28.5 (8.5) | |
Weight, kg | 57.9 (53.9–64.1) | 110.7 (89.1–143.5) | 81.4 (71.8–88.4) | <0.001 | 0.001 | 0.003 | −28.7 (8.6) | |
Glu, mmol/L | 5.5 (5.2–5.6) | 8.6 (7.2–12.4) | 5.5 (5.2–5.8) | <0.001 | 1.0 | <0.001 | −39.0 (17.2) | |
HbA1c, % | 5.5 (5.2–5.9) | 7.7 (7.4–8.2) | 5.6 (5.3–5.9) | <0.001 | 1.0 | <0.001 | −27.8 (−29.3, −27.3) | |
ALT, U/L | 32.0 (30.0–33.8) | 37.1 (26.8–65.3) | 33.5 (30.0–35.8) | 0.24 | 0.53 | 0.50 | −7.5 (−45.2, 5.2) | |
AST, U/L | 29.0 (25.3–31.8) | 32.0 (23.3–41.3) | 28.5 (23.5–34.5) | 0.35 | 0.74 | 0.16 | −11.5 (21.5) | |
TG, mmol/L | 0.7 (0.6–0.8) | 1.9 (1.5–2.1) | 1.5 (1.4–1.6) | 0.005 | 1.0 | 0.028 | −28.6 (−30.8, −10.1) | |
HDL-c, mmol/L† | 1.1 (0.1) | 1.0 (0.1) | 1.1 (0.1) | 0.79 | 0.82 | 0.39 | 7.5 (4.1) | |
LDL-c, mmol/L† | 2.2 (0.3) | 2.9 (0.5) | 2.2 (0.4) | <0.001 | 1.0 | 0.001 | −23.4 (2.5) | |
Hepatic PDFF, % | 7.8 (6.6–8.2) | 11.2 (9.8–18.3) | 7.8 (6.6–8.9) | <0.001 | 1.0 | 0.001 | −40.6 (11.9) | |
Pancreatic PDFF, % | 5.7 (5.0–5.9) | 7.8 (6.9–9.1) | 5.7 (5.3–5.9) | <0.001 | 1.0 | <0.001 | −30.1 (8.3) | |
Pancreatic head PDFF, % | 5.7 (5.2–6.4) | 9.0 (8.2–11.1) | 5.9 (5.2–6.6) | <0.001 | 1.0 | <0.001 | −39.2 (6.8) | |
Pancreatic body PDFF, % | 4.4 (3.9–5.3) | 5.6 (4.7–7.6) | 4.6 (4.0–5.5) | 0.005 | 0.75 | <0.001 | −19.9 (12.0) | |
Pancreatic tail PDFF, % | 4.7 (4.3–5.4) | 7.3 (5.5–7.9) | 4.8 (4.5–5.6) | 0.002 | 1.0 | 0.003 | −33.1 (−34.3, −23.3) |
Unless otherwise specified, data are medians, with interquartile ranges in parentheses. †, continuous variables are presented as the mean (SD). ‡, data are numbers of individuals, with percentages in parentheses. BMI, body mass index; Glu, glucose; HbA1c, glycated hemoglobin; ALT, alanine aminotransferase; AST, aspartate aminotransferase; TG, triglyceride; HDL-c, high-density lipoprotein cholesterol; LDL-c, low-density-lipoprotein cholesterol; PDFF, proton-density fat fraction.
Adiposity measures and adverse metabolic indices decreased 12 months after bariatric surgery. There was a 28.5% reduction in BMI (Figure 4) and a 27.8% reduction in HbA1c. The hepatic PDFF and all pancreatic PDFF significantly decreased after surgery (all P<0.01), and the values were statistically similar to those observed in the non-obese controls. Moreover, the change in BMI was associated with decreased hepatic PDFF (r=0.66; P=0.02) and pancreatic body PDFF (r=0.86; P<0.001).
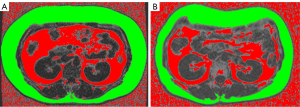
Discussion
Poor glycemic control among patients with T2D constitutes a major public health issue and is a leading risk factor for diabetic complications (22,23). High BMI is closely related to poor glycemic control (24). For obese patients with poorly controlled T2D, additional interventions may be required to achieve optimal glycemic control (25). Several studies have reported significant differences in fat distribution between normal-weight and obese participants with and without T2D (16,26). However, no previous studies have investigated differences in visceral fat and ectopic fat accumulation between poorly controlled and well-controlled diabetes in obese patients. Thus, the main objective of this study was to provide more insight into the effect of abnormal adiposity on glycemic control in obese patients.
First, our findings indicated that adipose pathology might be the primary driver of obesity-related comorbidities, consistent with the findings of previous studies (27). Not all individuals classified as obese have excessive adiposity. Moreover, even among people classified as super or super-super obese, not all will have metabolic complications, such as T2D (28). In the present study, although BMI was higher in the prediabetes group than in the NGT group, there was no significant difference in BMI between the new-onset T2D and NGT groups. Conversely, some people with normal-weight or mild obesity develop metabolic complications due to ectopic adipose deposits (27). For example, compared to White populations, Asian populations tend to develop T2D at a younger age, with a lower BMI, and with relatively little weight gain, likely owing to increased insulin resistance associated with increased visceral fat and inadequate beta cell response (29). The present study showed significant differences in VAT, hepatic PDFF, and pancreatic PDFF between the new-onset T2D, prediabetic, and NGT groups in obese patients, with more ectopic fat deposition in the T2D and prediabetic groups compared to the NGT group.
Furthermore, our study demonstrated that pancreatic tail PDFF was strongly associated with poorly controlled T2D. Poor glycemic control is associated with a poor prognosis in obese patients with T2D (30,31). Poorly controlled hyperglycemia also increases the severity and mortality of patients with coronavirus disease of 2019 (COVID-19) (32). In this study, TG and LDL-c were significantly higher in patients with poor glycemic control, which was similar to the studies reported by Benoit et al. (33). Moreover, we found that pancreatic tail PDFF was a novel imaging marker associated with glycemic control. One possible explanation for this finding is that the density of beta islet cells in the pancreatic tail is higher (up to twice as high) than that in the pancreatic head or body (34). It has been reported that patients undergoing distal pancreatectomy are more prone to develop long-term diabetes than those receiving pancreatic head resections (35,36). Therefore, potential inflammation and islet cell dysfunction in the pancreatic tail caused by steatosis may be associated with poorly controlled T2D (37).
Finally, our study indicated that bariatric surgery is an effective therapy for poorly controlled T2D and obesity. Weight loss is the most significant predictor of the remission of T2D, and loss of 15% or more of body weight in the long term can have a disease-modifying effect in people with T2D (38). In the present study, obese patients with poorly controlled T2D who lost 29% of their body weight had improved glycemic control, and showed decreased pancreatic fat content 12 months after bariatric surgery. The change in BMI was associated with decreased pancreatic PDFF. However, this study did not find an association between changes in pancreatic fat content and HbA1c.
This study has several limitations. It was a retrospective study. In addition, due to the strict inclusion criteria, the sample size of this study was relatively small, which may be accompanied by type II errors. Further large, prospective studies are required to obtain more accurate findings. Finally, this was a single-center study; multicenter studies are needed to explore the consistency of results across ethnic populations.
In conclusion, the results of the present study indicate that pancreatic tail fat content is strongly associated with poor glycemic control in obese patients with T2D. Bariatric surgery is an effective therapy for obesity and poorly controlled diabetes and significantly reduces the ectopic fat content.
Acknowledgments
Funding: This study has received funding from the Natural Science Foundation of Guangdong Province (No. 2017A030313901), the Guangzhou Science, Technology, and Innovation Commission (No. 201804010239), the Fundamental Research Funds for the Central Universities (No. 21621061), the Administration of Traditional Chinese Medicine of Guangdong Province (No. 2022113), the Medical Scientific Research Foundation of Guangdong Province (No. A2022337), and the Administration of Traditional Chinese Medicine of Guangdong Province (No. 2022110).
Footnote
Reporting Checklist: The authors have completed the STROBE reporting checklist. Available at https://qims.amegroups.com/article/view/10.21037/qims-22-1083/rc
Conflicts of Interest: All authors have completed the ICMJE uniform disclosure form (available at https://qims.amegroups.com/article/view/10.21037/qims-22-1083/coif). The authors have no conflicts of interest to declare.
Ethical Statement: The authors are accountable for all aspects of the work in ensuring that questions related to the accuracy or integrity of any part of the work are appropriately investigated and resolved. The study was conducted in accordance with the Declaration of Helsinki (as revised in 2013). The study was approved by the Ethics Committee of the First Affiliated Hospital of Jinan University, and individual consent for this retrospective analysis was waived.
Open Access Statement: This is an Open Access article distributed in accordance with the Creative Commons Attribution-NonCommercial-NoDerivs 4.0 International License (CC BY-NC-ND 4.0), which permits the non-commercial replication and distribution of the article with the strict proviso that no changes or edits are made and the original work is properly cited (including links to both the formal publication through the relevant DOI and the license). See: https://creativecommons.org/licenses/by-nc-nd/4.0/.
References
- Chobot A, Górowska-Kowolik K, Sokołowska M, Jarosz-Chobot P. Obesity and diabetes-Not only a simple link between two epidemics. Diabetes Metab Res Rev 2018;34:e3042. [Crossref] [PubMed]
- Kramer H, Cao G, Dugas L, Luke A, Cooper R, Durazo-Arvizu R. Increasing BMI and waist circumference and prevalence of obesity among adults with Type 2 diabetes: the National Health and Nutrition Examination Surveys. J Diabetes Complications 2010;24:368-74. [Crossref] [PubMed]
- Silveira EA, de Souza Rosa LP, de Carvalho Santos ASEA, de Souza Cardoso CK, Noll M. Type 2 Diabetes Mellitus in Class II and III Obesity: Prevalence, Associated Factors, and Correlation between Glycemic Parameters and Body Mass Index. Int J Environ Res Public Health 2020;17:3930. [Crossref] [PubMed]
- Kahn SE, Hull RL, Utzschneider KM. Mechanisms linking obesity to insulin resistance and type 2 diabetes. Nature 2006;444:840-6. [Crossref] [PubMed]
- Mata-Cases M, Rodríguez-Sánchez B, Mauricio D, Real J, Vlacho B, Franch-Nadal J, Oliva J. The Association Between Poor Glycemic Control and Health Care Costs in People With Diabetes: A Population-Based Study. Diabetes Care 2020;43:751-8. [Crossref] [PubMed]
- Alzaheb RA, Altemani AH. The prevalence and determinants of poor glycemic control among adults with type 2 diabetes mellitus in Saudi Arabia. Diabetes Metab Syndr Obes 2018;11:15-21. [Crossref] [PubMed]
- Juarez DT, Sentell T, Tokumaru S, Goo R, Davis JW, Mau MM. Factors associated with poor glycemic control or wide glycemic variability among diabetes patients in Hawaii, 2006-2009. Prev Chronic Dis 2012;9:120065. [Crossref] [PubMed]
- Zheng B, Su B, Price G, Tzoulaki I, Ahmadi-Abhari S, Middleton L. Glycemic Control, Diabetic Complications, and Risk of Dementia in Patients With Diabetes: Results From a Large U.K. Cohort Study. Diabetes Care 2021;44:1556-63. [Crossref] [PubMed]
- Hanipah ZN, Schauer PR. Bariatric Surgery as a Long-Term Treatment for Type 2 Diabetes/Metabolic Syndrome. Annu Rev Med 2020;71:1-15. [Crossref] [PubMed]
- Wu H, Ballantyne CM. Metabolic Inflammation and Insulin Resistance in Obesity. Circ Res 2020;126:1549-64. [Crossref] [PubMed]
- Chait A, den Hartigh LJ. Adipose Tissue Distribution, Inflammation and Its Metabolic Consequences, Including Diabetes and Cardiovascular Disease. Front Cardiovasc Med 2020;7:22. [Crossref] [PubMed]
- Ceniccola GD, Castro MG, Piovacari SMF, Horie LM, Corrêa FG, Barrere APN, Toledo DO. Current technologies in body composition assessment: advantages and disadvantages. Nutrition 2019;62:25-31. [Crossref] [PubMed]
- Wang M, Luo Y, Cai H, Xu L, Huang M, Li C, Dong Z, Li ZP, Feng ST. Prediction of type 2 diabetes mellitus using noninvasive MRI quantitation of visceral abdominal adiposity tissue volume. Quant Imaging Med Surg 2019;9:1076-86. [Crossref] [PubMed]
- Sun J, Lv H, Li M, Zhao L, Liu Y, Zeng N, Wei X, Chen Q, Ren P, Liu Y, Zhang P, Yang Z, Zhang Z, Wang Z. How much abdominal fat do obese patients lose short term after laparoscopic sleeve gastrectomy? A quantitative study evaluated with MRI. Quant Imaging Med Surg 2021;11:4569-82. [Crossref] [PubMed]
- Zhang QH, Zhao Y, Tian SF, Xie LH, Chen LH, Chen AL, Wang N, Song QW, Zhang HN, Xie LZ, Shen ZW, Liu AL. Hepatic fat quantification of magnetic resonance imaging whole-liver segmentation for assessing the severity of nonalcoholic fatty liver disease: comparison with a region of interest sampling method. Quant Imaging Med Surg 2021;11:2933-42. [Crossref] [PubMed]
- Sarma MK, Saucedo A, Darwin CH, Felker ER, Umachandran K, Kohanghadosh D, Xu E, Raman S, Thomas MA. Noninvasive assessment of abdominal adipose tissues and quantification of hepatic and pancreatic fat fractions in type 2 diabetes mellitus. Magn Reson Imaging 2020;72:95-102. [Crossref] [PubMed]
- Kiefer LS, Fabian J, Rospleszcz S, Lorbeer R, Machann J, Storz C, Kraus MS, Schlett CL, Roemer F, Wintermeyer E, Rathmann W, Nikolaou K, Peters A, Bamberg F. Assessment of the degree of abdominal myosteatosis by magnetic resonance imaging in subjects with diabetes, prediabetes and healthy controls from the general population. Eur J Radiol 2018;105:261-8. [Crossref] [PubMed]
- Addendum. 2. Classification and Diagnosis of Diabetes: Standards of Medical Care in Diabetes-2021. Diabetes Care 2021;44(Suppl. 1):S15-S33. Diabetes Care 2021;44:2182. [Crossref] [PubMed]
- Gavin TP, Ernst JM, Kwak HB, Caudill SE, Reed MA, Garner RT, Nie Y, Weiss JA, Pories WJ, Dar M, Lin CT, Hubal MJ, Neufer PD, Kuang S, Dohm GL. High Incomplete Skeletal Muscle Fatty Acid Oxidation Explains Low Muscle Insulin Sensitivity in Poorly Controlled T2D. J Clin Endocrinol Metab 2018;103:882-9. [Crossref] [PubMed]
- Raoufi M, Khalili S, Mansouri M, Mahdavi A, Khalili N. Well-controlled vs poorly-controlled diabetes in patients with COVID-19: Are there any differences in outcomes and imaging findings? Diabetes Res Clin Pract 2020;166:108286. [Crossref] [PubMed]
- Jang M, Park HW, Huh J, Lee JH, Jeong YK, Nah YW, Park J, Kim KW. Predictive value of sarcopenia and visceral obesity for postoperative pancreatic fistula after pancreaticoduodenectomy analyzed on clinically acquired CT and MRI. Eur Radiol 2019;29:2417-25. [Crossref] [PubMed]
- Tsuda A, Ishimura E, Ohno Y, Ichii M, Nakatani S, Machida Y, Mori K, Uchida J, Fukumoto S, Emoto M, Nakatani T, Inaba M. Poor glycemic control is a major factor in the overestimation of glomerular filtration rate in diabetic patients. Diabetes Care 2014;37:596-603. [Crossref] [PubMed]
- Huang ES, Liu JY, Moffet HH, John PM, Karter AJ. Glycemic control, complications, and death in older diabetic patients: the diabetes and aging study. Diabetes Care 2011;34:1329-36. [Crossref] [PubMed]
- Targher G, Zoppini G, Lippi G, Guidi GC, Muggeo M. Effect of serum gamma-glutamyltransferase and obesity on the risk of dyslipidemia and poor glycemic control in type 2 diabetic patients: cross-sectional findings from the Verona Diabetes Study. Clin Chem 2007;53:1867-9; author reply 1869-70. [Crossref] [PubMed]
- Arterburn DE, Telem DA, Kushner RF, Courcoulas AP. Benefits and Risks of Bariatric Surgery in Adults: A Review. JAMA 2020;324:879-87. [Crossref] [PubMed]
- Kiefer LS, Fabian J, Rospleszcz S, Lorbeer R, Machann J, Kraus MS, Roemer F, Rathmann W, Meisinger C, Heier M, Nikolaou K, Peters A, Storz C, Diallo TD, Schlett CL, Bamberg F. Distribution patterns of intramyocellular and extramyocellular fat by magnetic resonance imaging in subjects with diabetes, prediabetes and normoglycaemic controls. Diabetes Obes Metab 2021;23:1868-78. [Crossref] [PubMed]
- Bays HE. Adiposopathy is "sick fat" a cardiovascular disease? J Am Coll Cardiol 2011;57:2461-73. [Crossref] [PubMed]
- Stefan N, Häring HU, Hu FB, Schulze MB. Metabolically healthy obesity: epidemiology, mechanisms, and clinical implications. Lancet Diabetes Endocrinol 2013;1:152-62. [Crossref] [PubMed]
- Teufel F, Seiglie JA, Geldsetzer P, Theilmann M, Marcus ME, Ebert C, et al. Body-mass index and diabetes risk in 57 low-income and middle-income countries: a cross-sectional study of nationally representative, individual-level data in 685 616 adults. Lancet 2021;398:238-48. [Crossref] [PubMed]
- Long CA, Fang ZB, Hu FY, Arya S, Brewster LP, Duggan E, Duwayri Y. Poor glycemic control is a strong predictor of postoperative morbidity and mortality in patients undergoing vascular surgery. J Vasc Surg 2019;69:1219-26. [Crossref] [PubMed]
- Borgharkar SS, Das SS. Real-world evidence of glycemic control among patients with type 2 diabetes mellitus in India: the TIGHT study. BMJ Open Diabetes Res Care 2019;7:e000654. [Crossref] [PubMed]
- Zhu L, She ZG, Cheng X, Qin JJ, Zhang XJ, Cai J, et al. Association of Blood Glucose Control and Outcomes in Patients with COVID-19 and Pre-existing Type 2 Diabetes. Cell Metab 2020;31:1068-77.e3. [Crossref] [PubMed]
- Benoit SR, Fleming R, Philis-Tsimikas A, Ji M. Predictors of glycemic control among patients with Type 2 diabetes: a longitudinal study. BMC Public Health 2005;5:36. [Crossref] [PubMed]
- Wang X, Misawa R, Zielinski MC, Cowen P, Jo J, Periwal V, Ricordi C, Khan A, Szust J, Shen J, Millis JM, Witkowski P, Hara M. Regional differences in islet distribution in the human pancreas--preferential beta-cell loss in the head region in patients with type 2 diabetes. PLoS One 2013;8:e67454. [Crossref] [PubMed]
- Kang MJ, Jung HS, Jang JY, Jung W, Chang J, Shin YC, Kim SW. Metabolic effect of pancreatoduodenectomy: Resolution of diabetes mellitus after surgery. Pancreatology 2016;16:272-7. [Crossref] [PubMed]
- Kwon JH, Kim SC, Shim IK, Song KB, Lee JH, Hwang DW, Park KM, Lee YJ. Factors Affecting the Development of Diabetes Mellitus After Pancreatic Resection. Pancreas 2015;44:1296-303. [Crossref] [PubMed]
- Nadarajah C, Fananapazir G, Cui E, Gichoya J, Thayalan N, Asare-Sawiri M, Menias CO, Sandrasegaran K. Association of pancreatic fat content with type II diabetes mellitus. Clin Radiol 2020;75:51-6. [Crossref] [PubMed]
- Lingvay I, Sumithran P, Cohen RV, le Roux CW. Obesity management as a primary treatment goal for type 2 diabetes: time to reframe the conversation. Lancet 2022;399:394-405. [Crossref] [PubMed]