The relationship between false-lumen area ratio and renal replacement therapy after acute aortic dissection repair on bilateral artery cannulation: a cross-sectional study
Introduction
Debakey I acute aortic dissection (AAD), a complication of organ malperfusion, is common. Malperfusion may involve any major arterial side branch and result in myocardial, cerebral, spinal cord, visceral, and/or limb ischemia with varying frequencies and severities. Comprehensive management of AAD has improved the survival rate of patients after surgical repair, but malperfusion resulting from the impaired branch artery poses a continuing challenge and management dilemma for cardiovascular surgical teams (1).
Renal replacement therapy (RRT) is a common complication and the main predictor of postoperative mortality in AAD repair (2). A previous study indicated that preoperative renal malperfusion in patients did not necessarily develop into acute kidney injury after AAD repair. Patients with renal malperfusion due to false lumen compression against true lumen in the descending aorta were at a great risk for requiring RRT (3). Another previous study showed that dilatation and shrinkage in the descending aorta could occur in patients after AAD repair (4). The transformation of the true- or false-lumen diameter and volume potentially affects the fluid dynamics in the descending aorta and the renal blood flow perfusion. The ratio of the area of the false lumen compared to the area of the total lumen (FLAR) can be easily measured using imaging analysis software and computed tomography angiography (CTA). A higher FLAR could result in blood perfusion deficiency because the aorta may be seriously compressed, and the ostium of branch arteries may possibly involve the false lumen.
Different cannulation perfusion modes exert a distinct effect in the hemodynamic characteristics of vital organs (5). Despite the continuous revolution in artery cannulation strategies for AAD with malperfusion, the optimal strategy for artery cannulation to establish a cardiopulmonary bypass is still debated (6). The more commonly used method in clinical practice remains peripheral artery cannulation combining the axillary and femoral arteries. Here, we investigated the segmental change of the FLAR in the descending aorta after AAD repair on intraoperative right axillary and femur artery perfusion mode and the relationship between FLAR and RRT. We present the following article in accordance with the STROBE reporting checklist (available at https://qims.amegroups.com/article/view/10.21037/qims-22-1103/rc).
Methods
The study was conducted in accordance with the Declaration of Helsinki (as revised in 2013). The Institutional Review Board of Sichuan Provincial People’s Hospital approved the protocol of this observational study (No. 2021215), and the informed consent was waived due to the retrospective nature of the study.
Population
The patients who underwent surgery for AAD repair between March 2013 and March 2022 in Sichuan Provincial People’s Hospital were cross-sectionally investigated. Patients receiving total aortic arch (TAA) surgery with right axillary and femur artery cannulation perfusion were screened. The exclusion criteria included patients who had a lack of preoperative and postoperative CTA imaging data, or those with preoperative kidney disease, Debakey II aortic dissection, or treatment with other artery cannulation perfusion modes. A total of 228 individuals were included in this study.
Surgical procedure
The right axillary artery (RAA) and femoral artery were cannulated as arterial inflow to establish cardiopulmonary bypass (CPB) with a Seldinger technique (7). The choice site of femoral artery cannulation depended on the involvement of dissection identified with the preoperative CTA. All the aortic repairs were performed via a median sternotomy under mild-to-moderate hypothermia circulatory arrest (HCA) with a target to achieve systemic cooling to a rectal temperature of 20–25 ℃. During the HCA period, selective antegrade cerebral perfusion was administered via the RAA at the flow rate of 5–10 mL/kg/min with the pressure maintained at around 60 mmHg. The left carotid artery and left subclavian artery were conventionally clamped externally near their origin from the aortic arch during HCA. One elephant trunk stent (28 mm × 120 mm; CRONUS, MicroPort Scientifc Corporation, Shanghai, China) was conventionally implanted into the distal anastomosis in an open manner. The soft edge of the frozen elephant trunk and the trimmed aortic wall were anastomosed to a 4-branched graft (Maquet, Rastatt, Germany). The stent end was usually extended downward to the fourth thoracic vertebrate level.
CT image acquisition and analysis
All CT images of the participants were completed with a multidetector-row CT (Siemens Healthineers, Erlangen, Germany) and analyzed by 1 researcher who was experienced and blinded to this study. The aorta was divided into 3 segments: the descending thoracic aorta stretching from the end of the frozen stent (S1), the abdominal aorta above the orifice of the renal artery (S2), and the abdominal aorta between the orifice of the renal artery and the iliac bifurcation (S3) (8). The false-lumen area was calculated by subtracting the true lumen area from the total aortic area (the outer surface of the aortic wall) (9). The FLAR was defined as the maximal value in each segment of the descending thoracic aorta and was calculated by dividing the false lumen area by the aortic area on the CT scan using the area measurement tool of the Neusoft PACS/RIS Version 5.5 Workstation (Neusoft Corp., Shenyang, China) (10). The postoperative to preoperative ratio of the FLAR was calculated by dividing the postoperative FLAR value by the preoperative FLAR value.
Study end points and definitions
The primary end point of the study was the segmental change of the FLAR. The secondary end points were the incidence of RRT and oprative death. The indication of RRT was determined by the extent of postoperative acute kidney injury (AKI) according to the Kidney Disease: Improving Global Outcomes (KDIGO) classification (11). Operative death was defined as death occurring during the hospitalization or after discharge from the hospital within 30 days of the procedure. Hepatic dysfunction was defined as newly onset serum hepatic transaminase or/and bilirubin elevated by >1.5 times the baseline within 7 days after the surgery. The laboratory variables for detecting serum inflammatory response, including high-sensitivity C-reactive protein (hs-CRP) and interleukin-6 (IL-6), were conventionally examined after patients returned to the intensive care unit (ICU) (12). The lactate level was periodically examined through arterial blood gas analysis postoperatively (13). The Acute Physiology and Chronic Health Evaluation (APACHE) II score was calculated 1 day after the operation using specialized ICU software.
Criteria for the initiation and termination of RRT
We evaluated postoperative change in the plasma creatinine (Cr) concentration during the first postoperative week and compared it with the preoperative baseline value, as the degree of AKI was determined using the plasma Cr increase within 1 week according to the KDIGO classification. RRT was considered in patients with an increase of Cr >26.5 umol/L within 48 h after surgery, a urine volume <0.5 mL/kg/h lasting for 6 h and serum K+ >6.0 mmol/L or HCO3− <10 mmol/L. Within 48 h after the last RRT, if Cr was >50 umol/L (with a sampling interval greater than 12 h) or urine volume was >0.5 mL/kg/h within 12 h, serum K+ was <5.5 mmol/L, and HCO3− was >18 mmol/L, then termination of RRT was considered, as indicated in previous studies (14).
Statistical analysis
Categorical variables are reported as numbers and frequencies and were compared using the χ2 test. Continuous variables are presented as the mean±standard deviation and were compared using the Student t test if they were normally distributed. If continuous variables were not normally distributed, they are presented as the median and interquartile ranges and were compared using the Mann-Whitney test. In cases of small sample sizes (n≤5), the Fisher exact tests was used. After all data were tested for normal distribution and homogeneity of variance, paired t tests and Wilcoxon tests were performed for parametric and nonparametric data, respectively. The FLAR to predict the incidence of RRT was analyzed using the area under the receiver operating characteristic (ROC) curve (AUC). All statistical analyses were performed using SPSS 25.0 (IBM Corp., Armonk, NY, USA), and a 2-sided P value of <0.05 was considered statistically significant.
Results
Baseline characteristics
A total of 409 patients with AAD were screened. Among them, patients were excluded if their preoperative or postoperative enhanced computed tomography images were not available in our institution due to transferal from other hospitals (n=58); if they had Debakey II aortic dissection (n=35), a single kidney (n=1), or dialysis or chronic kidney injury preoperatively (n=26); or if they underwent other cannulation perfusion type (n=51). Patients with intraoperative and postoperative death within 24 h were also excluded (n=10). Finally, 228 cases were recruited in this study (Figure 1).
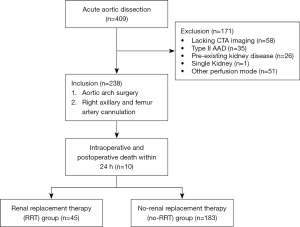
The FLAR results in 3 segments of the descending aorta
The postoperative CTA undertaken before patients were discharged from the hospital showed that the false lumen was patent in 162 patients (71.1%) in S1, 217 patients in S2 (95.2%), and 201 patients in S3 (88.2%; P<0.001). The FLAR on postoperative CTA was alleviated in 3 segments compared with the preoperative measurements (S1: 0.72±0.12 vs. 0.48±0.13, P<0.001; S2: 0.75±0.10 vs. 0.60±0.10, P<0.001; S3: 0.78±0.10 vs. 0.44±0.10, P<0.001; Figures 2,3). The postoperative/preoperative change of the FLAR was higher in S2 than in S1 and S3 (S1: 67%±14%; S2: 80%±8%; S3: 57%±12%; all P values <0.001; Figure 3).
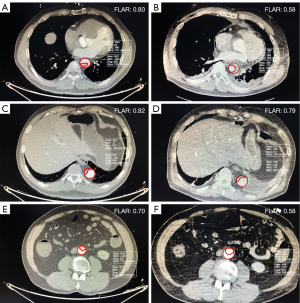
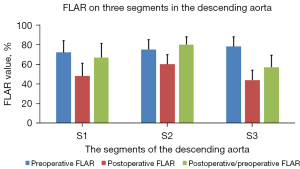
Analysis of RRT
Preoperative, perioperative, and postoperative outcomes were divided into 2 groups (the RRT group and the no-RRT group), and comparisons were made between these 2 groups. The 2 groups were similar with regard to demographic characteristics and comorbidities, except age {56 [50, 60] years for RRT group vs. 52 [46, 58] years for no-RRT group; P=0.04; Table 1}. The surgical type on the proximal end, operative time, and intraoperative blood product use were not remarkedly different between the 2 groups, as shown in Table 2. The postoperative to preoperative ratio of the FLAR on the S2 was higher in the RRT group than that in the no-RRT group (85%±7% vs. 79%±8%; P<0.001; Figure 4). The postoperative/preoperative ratio of the FLAR in S2, and not age, had the highest AUC (AUC 0.713) for predicting the incidence of RRT (CI: 0.626–0.801). The rates of 30-day mortality were 28.9% in the RRT group compared with 7.7% in the no-RRT group (P<0.001). Among these, the main causes were confirmed as stroke (n=2), sepsis (n=6), acute respiratory distress syndrome (ARDS; n=4), and deep sternal infection (n=1) in the RRT group, and stroke (n=9), sepsis (n=3), ARDS (n=1), and serious postoperative bleeding (n=1) in the no-RRT group. The duration of time in the ICU, the APACHE II score, and the duration of the ventilation time were higher in the RRT group than in the no-RRT group (4.5±1.4 vs. 3.9±1.5 days, P=0.021; 19±6 vs. 17±5, P=0.013; 58±20 vs. 48±19 h, P=0.001; Table 3).
Table 1
Characteristics | RRT (n=45) | No-RRT (n=183) | P value |
---|---|---|---|
Age (years) | 56 [50, 60] | 52 [46, 58] | 0.04 |
Male | 30 (68.9) | 129 (69.9) | 0.617 |
BMI (kg/m2) | 22.9±2.0 | 23.3±2.2 | 0.234 |
Previous medical history | |||
Hypertension | 36 (80.0) | 137 (74.9) | 0.471 |
Dyslipidemia | 11 (24.4) | 33 (18.0) | 0.329 |
Involved branch arteries | |||
Coronary artery | 5 (11.1) | 18 (9.8) | 0.799 |
Brachiocephalic artery | 10 (22.2) | 37 (20.2) | 0.766 |
Superior mesenteric artery | 17 (37.8) | 60 (32.8) | 0.526 |
Coeliac trunk artery | 20 (44.4) | 58 (31.7) | 0.106 |
Renal artery | 35 (77.8) | 125 (68.3) | 0.213 |
Renal artery ostium obstruction | 6 | 19 | 0.596 |
False-lumen perfusion origin | 21 | 67 | 0.234 |
Cardiac function | |||
LAD (mm) | 39.4±5.6 | 38.8±6.9 | 0.3 |
LVEDD (mm) | 51.9±6.3 | 51.3±6.0 | 0.562 |
LVEF (%) | 55.8±7.5 | 55.8±7.0 | 0.976 |
Laboratory findings | |||
hs-CRP (mg/L) | 26.4±12.1 | 27.2±12.3 | 0.685 |
hs-troponin I (μg/L) | 0.11 [0.05, 0.29] | 0.08 [0.03, 0.22] | 0.394 |
Lactate (mmol/L) | 0.35±0.16 | 0.34±0.13 | 0.528 |
Hb (g/L) | 135.3±20.9 | 132.2±18.2 | 0.326 |
WBC (109/L) | 10.6±2.0 | 10.9±2.2 | 0.535 |
Neutrophil (109/L) | 8.9±1.7 | 9.1±1.9 | 0.432 |
The data are presented as mean ± standard deviation, median [interquartile range], or number (frequency). RRT, renal replacement therapy; BMI, body mass index; LAD, left atrium diameter; LVEDD, left ventricular end-diastolic diameter; LVEF, left ventricular ejection fraction; hs-CRP, high-sensitivity C-reactive protein; Hb, hemoglobin; WBC, white blood count.
Table 2
Characteristics | RRT (n=45) | No-RRT (n=183) | P value |
---|---|---|---|
Surgical type on the sinus of the aorta | 0.944 | ||
Sinus repair (commissure suspension) | 23 (51.1) | 91 (49.7) | |
Bentall | 8 (17.8) | 28 (15.3) | |
Wheat | 4 (8.9) | 16 (8.7) | |
Carbrol (modified) | 10 (22.2) | 48 (26.2) | |
Intraoperative conditions (min) | |||
Procedure time | 403±23 | 401±23 | 0.556 |
CPB | 187±21 | 188±20 | 0.854 |
ACC | 91±18 | 88±17 | 0.363 |
HCA | 20±3 | 20±2 | 0.956 |
Intraoperative hypothermia (℃) | |||
Minimum rectal temperature | 22.3±2.9 | 22.3±2.9 | 0.97 |
Minimum nasopharyngeal temperature | 20.7±2.9 | 20.7±3.0 | 0.984 |
Intraoperative allogenic transfusion | |||
RBC (U) | 4.8±2.1 | 4.4±2.0 | 0.258 |
PLT (U) | 0.4±0.6 | 0.6±0.7 | 0.063 |
FFP (mL) | 633±257 | 623±207 | 0.786 |
Cryoprecipitation (U) | 1.5±1.8 | 1.4±1.9 | 0.856 |
The data are presented as mean ± standard deviation or number (frequency). RRT, renal replacement therapy; CPB, cardiopulmonary bypass; ACC; aortic cross clamp; HCA, hypothermia circulatory arrest; RBC, red blood cell; PLT, platelet; FFP, fresh frozen plasma.
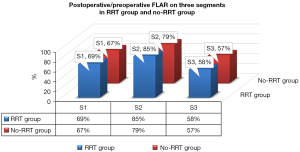
Table 3
Characteristics | RRT (n=45) | No-RRT (n=183) | P value |
---|---|---|---|
Inflammation response indexes | |||
hs-CRP (12 h, mg/L) | 73±16 | 69±16 | 0.167 |
hs-CRP (24 h, mg/L) | 121±17 | 116±16 | 0.077 |
hs-CRP (48 h, mg/L) | 197±16 | 189±16 | 0.007 |
IL-6 (24 h, pg/mL) | 149.1±60.6 | 144.4±59.5 | 0.636 |
IL-10 (24 h, pg/mL) | 8.9 [5.3, 14.7] | 9.1 [4.1, 18.8] | 0.620 |
Cardiac injury indexes | |||
TnI (12 h, ng/mL) | 10.6 [8.7, 14.0] | 10.2 [8.9, 12.2] | 0.176 |
TnI (24 h, ng/mL) | 6.3±1.9 | 5.8±1.6 | 0.066 |
TnI (48 h, ng/mL) | 3.2±1.5 | 3.0±1.1 | 0.193 |
Anaerobic metabolism | |||
Lactate (8 h, mmol/L) | 9.1±1.6 | 9.0±1.7 | 0.648 |
Lactate (16 h, mmol/L) | 5.3±1.2 | 5.3±1.0 | 0.988 |
Lactate (24 h, mmol/L) | 3.5±0.8 | 3.5±0.8 | 0.956 |
Lactate (48 h, mmol/L) | 2.2±0.8 | 2.1±0.6 | 0.485 |
ICU recovery | |||
APACHE Ⅱ score (24 h) | 19±6 | 17±5 | 0.013 |
Ventilation time (h) | 58±20 | 48±19 | 0.001 |
Duration in ICU stay (d) | 4.5±1.4 | 3.9±1.5 | 0.021 |
Chest tube drainage (mL) | 924±132 | 909±170 | 0.569 |
30-day recovery | |||
Death | 13 (28.9) | 14 (7.7) | <0.001 |
Stroke | 11 (24.4) | 28 (15.3) | 0.144 |
Duration in hospital stay (d) | 16 [14, 20] | 16 [14, 19] | 0.705 |
The data are presented as mean ± standard deviation, median [interquartile range], or number (frequency). RRT, renal replacement therapy; hs-CRP, highly sensitive C-reactive protein; IL, interleukin; TnI, troponin I; APACHE Ⅱ score, Acute Physiology, Age and Chronic Health Evaluation (APACHE) II score; ICU, intensive care unit.
Discussion
The principal findings of our study are as follows: (I) there was significant anatomically segmental changes of FLAR on the descending aorta; (II) the FLAR change on the abdominal aorta above the orifice of renal artery was less than that of the descending thoracic aorta and the abdominal aorta between the ostia of renal artery and the iliac bifurcation; (III) the lower change of FLAR on the abdominal aorta above the orifice of renal artery was related to clinical adverse events, including RRT in participants undergoing ascending aorta repair and aortic arch replacement.
Malperfusion is present in up to 40% of patients with AAD and results in increased morbidity and mortality (15). A previous study reported high incidences of aortic remodeling after total arch replacement was conducted using the frozen elephant trunk procedure for true-lumen expansion of the descending aorta (16). Aortic repair, including proximal aorta reconstruction, total arch replacement, and the frozen elephant trunk implantation procedure, is commonly administered in patients with type A AAD. These procedures can restore the bloodstream passage stemming from the proximal end of the descending aorta to the distal true lumen. However, the remodeling effect of the extra dissected descending aorta on the successive descending thoracic and abdominal aorta after the aortic repair procedure is well documented.
Combining cannulation with RAA and the femoral artery to repair AAD is widespread in clinical practice. Some known advantages of double artery cannulation include stabilization of CPB flow, avoidance of the overuse of large cannulas on the axillary artery, prevention of the retrograde perfusion that occurs with single femoral artery cannulation, and a shorter cooling period (17). The application of bilateral artery perfusion has been shown not to increase the surgical risks compared to RAA cannulation but could help reduce the incidence of postoperative AKI (18). In this situation, femoral cannulation can increase visceral perfusion and compensate for the partial blood to the celiac and superior mesenteric arteries, as the intimal flap motion block in the AAD model is perfused only by axillary cannulation (19). Therefore, we investigated the change in the morphologic characteristics before and after aortic repair under the bilateral artery cannulation mode via CTA imaging.
The imaging analysis using a CTA software system in this study showed the true-lumen area and total lumen area before and after AAD repair. FLAR was defined as the maximal value in the respective segment with the extra false-lumen area divided by the total lumen area. In a previous study, failed false-lumen volume reduction after thoracic endovascular aortic repair (TEVAR) was associated with a significantly increased mortality rate or reintervention during follow-up (20). In our study, the ratio of postoperative/preoperative FLAR in the middle segment tended to be higher compared to that in the proximal and distal segments of the descending aorta. This finding was similar to that of a previous study, which showed that the patent ratio of the abdominal false lumen was higher than that of the thoracic false lumen (4). Moreover, the lower segment of the abdominal aorta may be expanded more significantly than the other segments, which possibly occurs because the arterial inflow is situated on the femur artery and provides enough radial force from downward to upward even through the nonpulse bloodstream. The situation was similar with aortic remodeling after frozen elephant trunk repair (21). The olive-shaped false lumen change in the descending aorta exerts greater pressure on the outer wall of the true lumen in the upper abdominal aorta, where organs, like the kidneys, receive blood supplies from the main branching artery of the aorta.
Failed false-lumen shrinkage indicated worse aortic remodeling after AAD repair in our study. A positive association was observed between the lower FLAR change in the S2 segment and the events of RRT. This finding did not differ between the RRT and the no-RRT groups in the other 2 anatomic segments. The smaller morphological and volume change in the descending aorta indicated that there was more impedance from the outer wall of the true lumen, which transmitted the pressure to the orifice of the branch artery and jeopardized the organ perfusion. Although the preoperative false lumen could not predict the incidence rate of RRT, a smaller change in the postoperative/preoperative FLAR could predict it. This was reflected in the association between RRT and a higher inflammation response at 48 h after the procedure (hs-CRP), which was less likely to be affected by the surgical procedure strategy. This delayed inflammation reaction was more likely to have been affected by the postoperative recovery condition of the patient rather than by intraoperative management (22). It has also been reported that RRT also predicts higher medical costs due to more critical care conditions and longer durations of ICU stays (23).
The limitations of this study included its relatively small sample, the lack of long-term follow-up, and the use of a single imaging indicator, which might have been underpowered for identifying the morphological features associated with clinical events. However, we analyzed a large number of CT data sets on a population with homogeneous cannulation. A previous study used the maximal aortic diameter as a predictor of postoperative false-lumen volume dilation after aortic replacement for acute Debakey type I aortic dissection (9). In another study, apart from increases in aortic diameter, new or increased partial false-lumen thrombosis areas and increases in the circumferential extent of the false lumen were also strongly associated with late adverse events in medically treated type B aortic dissection (24). FLAR is more visible and more easily quantifiable; therefore, we only analyzed the index of the maximal FLAR in different segments instead of the maximal aortic diameter as the primary focus. The serial analysis of each participant in 3 segments resulted would require tedious manual labor for the imaging researcher, and thus addition of indicators would be far too onerous.
Conclusions
This study investigated the segmental changes of the FLAR in the descending aorta during the early phase of postoperative aortic dissection after AAD repair with intraoperative right axillary and femur artery perfusion mode and the relationship between FLAR and RRT. Our results demonstrated that postoperative/preoperative FLAR exhibited a differential change in the 3 segments in the descending aorta. When compared with patients in the no-RRT group, those in the RTT group were associated with less change in the postoperative/preoperative FLAR and more 30-day mortality.
Acknowledgments
Funding: This work was supported by the Science Fund for Distinguished Young Scholars of Sichuan Province (No. 2021JDJQ0041), the Huanhua Talent for Supporting Discipline Backbone of Sichuan Provincial People’s Hospital (No. SY2022017), and the National Natural Science and Technology Foundation of China (No. 81800274).
Footnote
Reporting Checklist: The authors have completed the STROBE reporting checklist. Available at https://qims.amegroups.com/article/view/10.21037/qims-22-1103/rc
Conflicts of Interest: All authors have completed the ICMJE uniform disclosure form (available at https://qims.amegroups.com/article/view/10.21037/qims-22-1103/coif). The authors have no conflicts of interest to declare.
Ethical Statement: The authors are accountable for all aspects of the work in ensuring that questions related to the accuracy or integrity of any part of the work are appropriately investigated and resolved. The study was conducted in accordance with the Declaration of Helsinki (as revised in 2013). The Institutional Review Board of Sichuan Provincial People’s Hospital approved the protocol of this observational study (No. 2021215), and the informed consent was waived due to the retrospective nature of the study.
Open Access Statement: This is an Open Access article distributed in accordance with the Creative Commons Attribution-NonCommercial-NoDerivs 4.0 International License (CC BY-NC-ND 4.0), which permits the non-commercial replication and distribution of the article with the strict proviso that no changes or edits are made and the original work is properly cited (including links to both the formal publication through the relevant DOI and the license). See: https://creativecommons.org/licenses/by-nc-nd/4.0/.
References
- Zhu Y, Lingala B, Baiocchi M, Tao JJ, Toro Arana V, Khoo JW, Williams KM, Traboulsi AA, Hammond HC, Lee AM, Hiesinger W, Boyd J, Oyer PE, Stinson EB, Reitz BA, Mitchell RS, Miller DC, Fischbein MP, Woo YJ, Type A. Aortic Dissection-Experience Over 5 Decades: JACC Historical Breakthroughs in Perspective. J Am Coll Cardiol 2020;76:1703-13. [Crossref] [PubMed]
- Chen X, Zhou J, Fang M, Yang J, Wang X, Wang S, Li L, Zhu T, Ji L, Yang L. Incidence- and In-hospital Mortality-Related Risk Factors of Acute Kidney Injury Requiring Continuous Renal Replacement Therapy in Patients Undergoing Surgery for Acute Type a Aortic Dissection. Front Cardiovasc Med 2021;8:749592. [Crossref] [PubMed]
- Helgason D, Helgadottir S, Ahlsson A, Gunn J, Hjortdal V, Hansson EC, Jeppsson A, Mennander A, Nozohoor S, Zindovic I, Olsson C, Ragnarsson SO, Sigurdsson MI, Geirsson A, Gudbjartsson T. Acute Kidney Injury After Acute Repair of Type A Aortic Dissection. Ann Thorac Surg 2021;111:1292-8. [Crossref] [PubMed]
- Park KH, Lim C, Choi JH, Chung E, Choi SI, Chun EJ, Sung K. Midterm change of descending aortic false lumen after repair of acute type I dissection. Ann Thorac Surg 2009;87:103-8. [Crossref] [PubMed]
- Deng L, Qin H, Guan Z, Mu Q, Xia Q, Wang M, Huang WH, Gu K. Computational numerical analysis of different cannulation methods during cardiopulmonary bypass of type A aortic dissection model based on computational fluid dynamics. Ann Transl Med 2021;9:667. [Crossref] [PubMed]
- Moorthy PSK, Sakijan AS. Malperfusion in acute type A aortic dissection: how we handle the challenge? Indian J Thorac Cardiovasc Surg 2022;38:122-31. [Crossref] [PubMed]
- Jiang Q, Yu T, Huang K, Liu L, Zhang X, Hu S. Feasibility, safety, and short-term outcome of totally thoracoscopic mitral valve procedure. J Cardiothorac Surg 2018;13:133. [Crossref] [PubMed]
- Wan Ab Naim WN, Sun Z, Liew YM, Chan BT, Jansen S, Lei J, Ganesan PB, Hashim SA, Sridhar GS, Lim E. Comparison of diametric and volumetric changes in Stanford type B aortic dissection patients in assessing aortic remodeling post-stent graft treatment. Quant Imaging Med Surg 2021;11:1723-36. [Crossref] [PubMed]
- Yamashita Y, Joo K, Okamoto K, Nakata Y, Ochiai Y, Tokunaga S. Postoperative Maximal Aortic Diameter is a Significant Predictor of Dilation of the Residual Dissected Aorta after Aortic Replacement for Acute Debakey Type I Aortic Dissection. Ann Vasc Surg 2022;81:121-8. [Crossref] [PubMed]
- Kim JH, Lee SH, Lee S, Youn YN, Yoo KJ, Joo HC. Role of False Lumen Area Ratio in Late Aortic Events After Acute Type I Aortic Dissection Repair. Ann Thorac Surg 2022;114:2217-24. [Crossref] [PubMed]
- Nishigawa K, Fukui T, Uemura K, Takanashi S, Shimokawa T. Preoperative renal malperfusion is an independent predictor for acute kidney injury and operative death but not associated with late mortality after surgery for acute type A aortic dissection. Eur J Cardiothorac Surg 2020;58:302-8. [Crossref] [PubMed]
- Jiang Q, Wang Z, Guo J, Yu T, Zhang X, Hu S. Retrospective Comparison of Endoscopic Versus Open Procedure for Mitral Valve Disease. J Invest Surg 2021;34:1000-6. [Crossref] [PubMed]
- Jiang Q, Li H, Huang X, Yu L, Lueck S, Hu S. Postnatal exposure to hypobaric hypoxia and its impact on inflammation and injury indexes after a cardiac valve procedure. Interact Cardiovasc Thorac Surg 2020;31:789-95. [Crossref] [PubMed]
- Wang Z, Ge M, Chen T, Chen C, Zong Q, Lu L, Wang D. Independent risk factors and the long-term outcomes for postoperative continuous renal replacement treatment in patients who underwent emergency surgery for type a acute aortic dissection. J Cardiothorac Surg 2020;15:100. [Crossref] [PubMed]
- Norton EL, Khaja MS, Williams DM, Yang B. Type A aortic dissection complicated by malperfusion syndrome. Curr Opin Cardiol 2019;34:610-5. [Crossref] [PubMed]
- Kuroda Y, Uchida T, Ohba E, Yamashita A, Nakai S, Kobayashi K, Ochiai T, Sadahiro M. Aortic remodelling effect of the frozen elephant trunk technique on Stanford type A acute aortic dissection. Interact Cardiovasc Thorac Surg 2021;32:789-91. [Crossref] [PubMed]
- Kusadokoro S, Kimura N. Double arterial cannulation: a classical yet useful cannulation strategy-comment on cannulation strategy in frozen elephant trunk for type A aortic dissection: double arterial cannulation approach. Eur J Cardiothorac Surg 2022;62:ezac231. [Crossref] [PubMed]
- Zhang H, Xie W, Lu Y, Pan T, Zhou Q, Xue Y, Wang D. Double arterial cannulation versus right axillary artery cannulation for acute type A aortic dissection: a retrospective study. J Cardiothorac Surg 2021;16:326. [Crossref] [PubMed]
- Heo W, Lee GH, Kim TH, Lee Y, Huh H, Ha H, Song SW, Yoo KJ. Quantification of visceral perfusion and impact of femoral cannulation: in vitro model of aortic dissection. Eur J Cardiothorac Surg 2022;62:ezab508. [Crossref] [PubMed]
- Kim TH, Ko YG, Kwon SW, Choi D, Lee DY, Shim WH, Hyon MS. Large false lumen area is a predictor of failed false lumen volume reduction after stent-graft repair in type B aortic dissection. J Endovasc Ther 2014;21:697-706. [Crossref] [PubMed]
- Sato H, Fukada J, Tamiya Y, Mikami T. Morphometric Predictors of Aortic Remodeling after Frozen Elephant Trunk Repair of Type A Dissection. Ann Vasc Surg 2022;84:179-86. [Crossref] [PubMed]
- Jiang Q, Xiang B, Wang H, Huang K, Kong H, Hu S. Remote ischaemic preconditioning ameliorates sinus rhythm restoration rate through Cox maze radiofrequency procedure associated with inflammation reaction reduction. Basic Res Cardiol 2019;114:14. [Crossref] [PubMed]
- Jiang Q, Yu T, Huang K, Huang X, Zhang Q, Hu S. The impact of medical insurance reimbursement on postoperative inflammation reaction in distinct cardiac surgery from a single center. BMC Health Serv Res 2022;22:494. [Crossref] [PubMed]
- Higashigaito K, Sailer AM, van Kuijk SMJ, Willemink MJ, Hahn LD, Hastie TJ, Miller DC, Fischbein MP, Fleischmann D. Aortic growth and development of partial false lumen thrombosis are associated with late adverse events in type B aortic dissection. J Thorac Cardiovasc Surg 2021;161:1184-1190.e2. [Crossref] [PubMed]