Technical comparison of continuous versus intermittent perfusion computed tomography computed tomography scans of the human pancreas
Introduction
Perfusion computed tomography (CT) imaging offers functional information about specific tissue or organs by continuously injecting a contrast medium into the vein. It is an increasingly attractive adjunct to conventional CT for neoplastic grading, predicting prognosis, and evaluating the response to therapy (1-4). With the advancement of CT equipment and technology, pancreatic perfusion CT has been used to effectively differentiate pancreatic adenocarcinoma and neuroendocrine tumors (5), as well as to predict the development of pancreatic necrosis from early-stage acute pancreatitis (6,7). However, perfusion CT is normally associated with high radiation exposure to patients due to the requirement for repeated scanning. To optimize the clinical application of perfusion CT in pancreatic diseases, we conducted a retrospective analysis using the electronic medical records of 40 patients who underwent different perfusion CT imaging techniques in The First Affiliated Hospital of Zhengzhou University to determine identify the significant influence of different perfusion scanning patterns on certain perfusion parameters. Our results demonstrated the feasibility of the intermittent perfusion scan, which has advantages over the continuous perfusion scan for detecting pancreatic disease.
Methods
Patients
Forty patients who had undergone perfusion CT scans of the pancreas from December 2017 to December 2018 at the First Affiliated Hospital of Zhengzhou University were identified in our database and included in this retrospective analysis. The inclusion criteria were as follows: (I) patients without diagnosed pancreatic disease and other diseases that affected their pancreatic blood supply and (II) with acquired CT images without severe motion artifacts or metal artifacts that could affect the assessment. These patients were suspected of having pancreatic lesions due to elevated CA199 or other abnormal biochemical markers of pancreatic function and were grouped into groups A and B (n=20 in each group). Patients in group A underwent continuous pancreatic perfusion CT, while patients in group B underwent 1-stop whole pancreas CT perfusion imaging combined with enhancement. The study was conducted in accordance with the Declaration of Helsinki (as revised in 2013). This retrospective study was reviewed and approved by the ethics committee of the First Affiliated Hospital of Zhengzhou University. All participants had a signed written informed consent.
CT imaging techniques
The 2 scanning methods were performed on a GE Revolution CT (GE Healthcare) according to standard protocols. Patients fasted for 4 to 6 hours before the CT scan and drank 800 to 1,000 mL of water 5 to 10 minutes before they underwent scanning. To reduce respiratory motion artifacts, all patients were trained to take a slightly shallow breath and were fixed with abdominal belts before the scan. Nonionic contrast agent (70 mL, iopamidol, 350 mg I/mL) was injected at a flow rate of 5 mL/s by mechanical power injector with bolus tracking. Then, 20 mL of saline was injected with bolus tracking to ensure all contrast medium reached the central veins, serving as a bolus chaser to increase peak arterial enhancement. The perfusion scope covered the whole pancreas. The continuous perfusion scan method in group A was an axial pattern, and the Z-axis scanning length was about 150 mm. The tube voltage was 100 kV, and the tube current was 100 mA. The adaptive statistical iterative reconstruction-V (ASIR-V) was 50%, the rotation time was 0.5 s, the scanning thickness was 5 mm, and the pitch was 0.992:1. The scan time for group A was 2 seconds per scan, the exposure time was 1.5 s, and the interval time was 0.5 s. The total scanning time was 50 s (25 passes; Figure 1A). The intermittent perfusion scan method in group B started helical scanning after 20.5 s (perfusion scanning occurred 8 times after 6 s during injection of the nonionic contrast agent). The time of helical scanning and conversion between the helical mode and axial mode was about 8.6 to 13.7 s. Helical scanning was arterial phase scanning; perfusion scanning occurred 13 times for the whole pancreas in the axial pattern and then was converted to helical pattern for venous phase scanning. The helical pattern tube voltage was 120 kVp with automatic tube current modulation (Auto mA). The axial pattern was the same as that of group A. The total scanning time was 64.6 to 70.0 s (25 passed; Figure 1B).
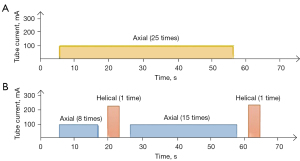
Imaging analysis and measurement
Images were analyzed by 2 radiologists on the GE AW4.7 professional workstation (GE Healthcare). The images were sent to the postprocessing workstation for motion artifact correction and loaded into CT perfusion 4D software for analysis. The abdominal aorta was selected as the inflow artery, and pancreatic tissue was sketched. Regions of interest were positioned at normal pancreatic tissue by avoiding vessels and calcification, and values of CT perfusion parameters were obtained, including blood flow (BF) per 100 g of tissue per minute (mL/100 g/min), blood volume (BV) per 100 g of tissue (mL/100 g), permeability surface (PS) per 100 g of tissue per minute (mL/100 g/min), mean transit time (MTT), time to peak (TTP), arrival pulse residual function peak transit time (Tmax), the delay time of arrival of contrast agent (IRF T0), mean slope of increase (MSI), and positive enhanced integral (PEI).
The system automatically recorded the dose-length product (DLP; mGy × cm) after scanning and then calculated the effective radiation dose (ED; mSv) based on the following formula: ED = k × DLP (where k = 0.015 mSv × mGy−1 × cm−1; the adult abdominal CT scan conversion coefficient).
Statistical analysis
Statistical analysis was performed using SPSS 21.0 (IMB Corp). Intraclass correlation coefficient (ICC) was used to test the consistency of the 2 observers’ measurements (ICC ≥0.75, very good consistency; 0.5≤ ICC <0.75, good consistency; ICC <0.5, very poor consistency). The basic data are expressed as mean ± standard deviation or median and interquartile range. The chi-squared test was used to compare the sex difference of the participants. The normality of the distribution of data was assessed using the Shapiro-Wilk test, and equal variance was assessed using the Levene test. An independent sample t-test or nonparametric test was used to compare the difference in perfusion parameters between different groups. A P value <0.05 was considered statistically significant.
Results
Basic characteristics of patients between the 2 groups
In the study, 20 participants (11 men and 9 women; median age 59.5 years; age range, 22–80 years) were included in group A, and 20 participants (8 men and 12 women; median age 50 years; age range, 28–76 years) were included in group B. There were no statistically significant differences in age or sex characteristics between the 2 groups (P>0.05, respectively; Table 1).
Table 1
Group | Number of participants | Age (years) | Sex (male/female) |
---|---|---|---|
Group A | 20 | 59.5 (22 to 80) | 11/9 |
Group B | 20 | 50 (28 to 76) | 8/12 |
χ2/t | –0.690 | 2.000 | |
P | 0.490 | 0.157 |
Age is expressed as the median (interquartile range). Group A underwent continuous perfusion scanning; group B underwent intermittent perfusion scanning.
ICC of perfusion parameters between the 2 radiologists
As shown in Table 2, high interobserver reliability was observed for different pancreatic perfusion parameters across different pancreatic locations. Except for the pancreatic body’s PS (ICC =0.688) and tail’s MTT (ICC =0.734) in group B, which had good consistency between the 2 observers (0.5≤ ICC <0.75), all other parameters had very good consistency (ICC ≥0.75).
Table 2
Perfusion parameters | Group A (ICC value), head/neck/body/tail | Group B (ICC value), head/neck/body/tail |
---|---|---|
BF (mL/100 g/min) | 0.856/0.894/0.937/0.916 | 0.848/0.856/0.871/0.837 |
BV (mL/100 g) | 0.949/0.873/0.944/0.853 | 0.954/0.949/0.858/0.847 |
PS (mL/100 g/min) | 0.842/0.930/0.928/0.905 | 0.904/0.810/0.688*/0.915 |
MTT (s) | 0.894/0.875/0.893/0.810 | 0.896/0.839/0.828/0.734* |
TTP (s) | 0.985/0.922/0.894/0.944 | 0.917/0.947/0.856/0.812 |
Tmax (s) | 0.909/0.892/0.897/0.880 | 0.820/0.896/0.914/0.827 |
IRF T0 (s) | 0.902/0.849/0.971/0.823 | 0.812/0.836/0.800/0.825 |
MSI | 0.959/0.834/0.871/0.889 | 0.916/0.939/0.969/0.943 |
PEI | 0.894/0.886/0.938/0.846 | 0.933/0.854/0.857/0.863 |
Data are the ICC value compared between different parameters. Group A underwent continuous perfusion scanning; group B underwent intermittent perfusion scanning. *, an ICC value of between 0.5 and 0.75. BF, blood flow; BV, blood volume; PS, permeability surface; MTT, mean transit time; TTP, time to peak; Tmax, arrival pulse residual function peak transit time; IRF T0, the delay time of arrival of contrast agent; MSI, mean slope of increase; PEI, positive enhanced integral; ICC, interclass correlation coefficient.
Comparison of perfusion parameters between different parts of the pancreas
Intragroup comparison of the perfusion parameters across different parts of the pancreas showed that the MSIs in group A were significantly different (P=0.028). The pancreas head had the lowest value, and the tail had the highest value (about 20% difference). The PS parameter in group A showed a marginal difference, with a P value of 0.058. Other than these 2 parameters, other parameters were not statistically different in each group at each pancreas location (Table 3).
Table 3
Parameters | Parts | |||||
---|---|---|---|---|---|---|
Head | Neck | Body | Tail | F value | P value | |
BF (mL/100 g/min) | ||||||
Group A | 89.697±18.795 | 99.159±15.866 | 94.848±19.920 | 96.246±16.814 | 0.976 | 0.409 |
Group B | 94.149±14.905 | 99.720±21.366 | 94.067±14.042 | 99.490±11.042 | 0.808 | 0.493 |
BV (mL/100 g) | ||||||
Group A | 15.256±2.925 | 13.940±2.691 | 16.142±4.006 | 16.446±3.709 | 2.214 | 0.093 |
Group B | 16.953±3.602 | 17.173±3.918 | 18.401±2.513 | 17.374±3.781 | 0.671 | 0.573 |
PS (mL/100 g/min) | ||||||
Group A | 34.205±9.612 | 34.898±11.592 | 28.861±10.448 | 27.823±8.228 | 2.599 | 0.058 |
Group B | 24.377±8.413 | 25.794±8.149 | 22.158±6.017 | 21.509±7.768 | 1.345 | 0.266 |
MTT (s) | ||||||
Group A | 10.617±3.132 | 9.064±2.497 | 10.552±2.527 | 10.446±3.381 | 1.295 | 0.282 |
Group B | 11.047±1.852 | 11.133±2.327 | 10.945±2.627 | 11.065±2.524 | 0.219 | 0.883 |
TTP (s) | ||||||
Group A | 17.116±3.827 | 17.290±3.751 | 16.337±2.942 | 16.450±3.539 | 0.362 | 0.781 |
Group B | 17.818±2.696 | 17.757±3.219 | 17.337±2.173 | 18.046±2.760 | 0.234 | 0.873 |
Tmax (s) | ||||||
Group A | 5.304±1.429 | 4.870±1.348 | 5.191±1.183 | 5.236±1.502 | 0.394 | 0.758 |
Group B | 5.684±0.721 | 5.241±0.999 | 5.551±0.955 | 5.709±1.503 | 0.791 | 0.503 |
IRF T0 (s) | ||||||
Group A | 0.314±0.395 | 0.303±0.438 | 0.267±0.505 | 0.335±0.344 | 1.395 | 0.251 |
Group B | 0.591±0.827 | 0.271±0.365 | 0.220±0.250 | 0.350±0.363 | 0.457 | 0.713 |
MSI | ||||||
Group A | 5.271±1.147 | 5.388±0.966 | 5.871±1.504 | 6.340±1.215 | 3.205 | 0.028* |
Group B | 4.955±1.234 | 5.170±1.414 | 5.243±1.500 | 5.440±1.555 | 0.392 | 0.759 |
PEI | ||||||
Group A | 0.307±0.050 | 0.304±0.088 | 0.305±0.093 | 0.304±0.057 | 0.011 | 0.412 |
Group B | 0.344±0.060 | 0.361±0.051 | 0.342±0.048 | 0.350±0.073 | 0.412 | 0.745 |
Data are expressed as numbers (mean ± standard deviation) compared between different parts of the pancreas. The P value was calculated with the F test. Group A underwent continuous perfusion scanning; group B underwent intermittent perfusion scanning. *, a P value <0.05. BF, blood flow; BV, blood volume; PS, permeability surface; MTT, mean transit time; TTP, time to peak; Tmax, arrival pulse residual function peak transit time; IRF T0, the delay time of arrival of contrast agent; MSI, mean slope of increase; PEI, positive enhanced integral.
Comparison of the perfusion parameters between the 2 groups
Intergroup comparison of perfusion parameters across different parts of pancreas showed that. In group A compared to group B, the BV of the pancreatic head was smaller (15.256±2.925 vs. 16.953±3.602), the PEI was smaller (0.307±0.050 vs. 0.344±0.060) and the PS was larger (34.205±9. 612 vs. 24.377±8.413); BV of the pancreatic neck was smaller (13.940±2.691 vs. 17.173±3.918), PEI was smaller (0.304±0.088 vs. 0.361±0.051) and PS was larger (34.898±11.592 vs. 25.794±8. 149); BV of the pancreatic body was smaller (16.142±4.006 vs. 18.401±2.513), PEI was smaller (0.305±0.093 vs. 0.342±0. 048) and PS was larger (28.861±10.448 vs. 22.158±6. 017); the BV of the pancreatic tail was smaller (16.446±3.709 vs. 17.374±3.781), the PEI was smaller (0.304±0.057 vs. 0.350±0.073), and the PS was larger (27.823±8.228 vs. 21.509±7.768) (P<0.05). BV and PEI were consistently higher in group B than those in group A at all parts of pancreas (P<0.05), while PS was consistently lower in group B than that in group A at all parts of pancreas (P<0.05) (Figure 2). Other parameters are not statistically different between the two groups at any location of pancreas (Table 3). The effective radiation dose of continuous scanning mode is 17.973±3.698 mSv, which is slightly higher than that of intermittent scanning mode (16.657±2.259 mSv).
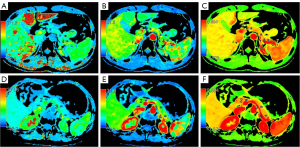
Discussion
A pancreatic CT perfusion scan is a dynamic and noninvasive technique for discerning information about pancreatic diseases because of its capacity to measure the hemodynamics of pancreatic tissue. There have been many advancements in pancreatic CT perfusion after the Miles report was published in 1995 (8). The method has been increasingly used to diagnose and grade pancreatic tumors, predict prognoses and response to therapy, and assess other aspects of various pancreatic diseases (9-11). In this study, our comparative analysis of different clinical scanning methods of pancreatic perfusion CT suggested that an intermittent perfusion scan can offer consistent perfusion parameters and thus satisfy the clinical diagnosis of pancreatic diseases by helical scanning.
Pancreatic blood supply originates from branches of the gastroduodenal artery, superior mesenteric artery, and splenic artery. Perfusion CT can evaluate the blood perfusion of the whole pancreas. Previous studies reported that the perfusion parameters of the whole brain were not different under different scanning interval times (12,13). In this study, none of the 9 perfusion parameters differed between the 2 scanning methods among different pancreatic parts (Table 3). This finding is consistent with the results of the brain study. However, the MSI parameter of various parts of the pancreas within the continuous perfusion group showed a significant difference (P=0.028), and the pancreas head had the highest value, while the tail had the lowest value (about 20% difference). This finding may indicate a high sensitivity of the continuous perfusion scanning in differentiating the blood perfusion characteristics of different pancreas parts, as blood vessels in the pancreatic head form more arcades, while in the tail, the branches of all arteries form more anastomosis networks.
Significant differences in the values of the parameters BV, PS, and PEI of the pancreatic head, body, and tail between the 2 groups were observed (Table 3). This finding may be due to the kinetics of BF at different regions of the pancreas that lead to different peak perfusion times, which suggests it could be better to capture these parameters using the continuous perfusion scan protocol. Under intermittent perfusion scan, the values of parameters BV and PEI were significantly higher than those under the continuous perfusion scan, which could be attributed to the rich anastomotic loops of pancreatic vascularization. Recently, we reported that pancreatic neuroendocrine tumor lesions showed increased BV and PEI values under the perfusion scan in comparison to the tumor-free pancreatic parenchyma (3). Taken together, these findings indicate that the high sensitivity of intermittent perfusion scans might be beneficial in identifying perfusion abnormality under disease conditions. Moreover, the intermittent perfusion scan provides additional arteriovenous enhancement images while obtaining a great number of perfusion parameters, which could be excellent for improving the detection rate of negative tumor lesions (3).
One limitation of the pancreatic CT perfusion scan is the radiation dose, which linearly increases with the number of scanning series (14). The radiation dose is related to tube voltage, tube current, scanning time, and scanning scope. It is proportional to the square of tube voltage; therefore, reducing the tube voltage can reduce the radiation dose most effectively (15,16). However, doing so comprises the image quality, as the image noise rises. The Revolution CT instrument using ASIR-V could effectively reduce the image noise caused by the low tube voltage and improve the density resolution, thus providing images with superior anatomical detail and high diagnostic acceptability (17-19). In the current study, patients were scanned using the Revolution CT, and the tube voltage setting was 100 kVp in the perfusion scanning. Nakaura et al. (20) reported that the performance of an abdominal CT with a tube voltage setting of 100 kVp produced a 22% radiation dose reduction compared with the 120 kVp setting and achieved a similar mean signal-to-noise ratio. This supports the advantage of the relatively low dose of perfusion scan with good image quality in pancreas perfusion imaging.
The Z-axis scope of conventional perfusion scanning is usually limited. The Revolution CT has a 16-cm Z-axis coverage, which reduces scanning time and sometimes decreases radiation dose (21,22). In our analysis, intermittent perfusion scanning had a reduced perfusion time compared to that of continuous scanning. This not only satisfies the clinical need for an efficient diagnosis but also offers consistent perfusion parameters. These findings indicate that the perfusion CT scan in Revolution CT that is associated with a low tube voltage and low tube current not only reduces the radiation dose but also retains the imaging consistency. The value of a perfusion CT scan for the diagnosis of pancreatic diseases is well recognized. Our study provides a new scanning modality for CT perfusion of the pancreas that will further expand the application of CT perfusion in pancreatic diseases.
This study had a few limitations. The number of samples in each group was relatively small. Furthermore, the pancreatic perfusion CT scan protocol is controversial and not yet standardized. However, this study provided first-hand data that introduced the effect of the perfusion CT time interval on perfusion parameters in the pancreas.
The influence of scanning intervals on the whole pancreatic BV, PS, and PEI was statistically significant, while the influence of BF, MTT, TTP, Tmax, IRF T0, and MSI parameters was not. The study aimed to optimize the pancreatic perfusion CT scan. According to the findings of this study, intermittent pancreatic perfusion CT should be performed for the diagnosis of pancreatic disease.
Conclusions
Intermittent perfusion scanning using Revolution CT was shown to provide a new scanning modality for CT perfusion of the pancreas. This new modality had a low tube voltage and a low tube current, which not only reduced the radiation dose but also retained the imaging consistency. This new modality will be more effective in expanding the application of pancreatic perfusion CT in the examination of pancreatic disease.
Acknowledgments
Funding: None.
Footnote
Conflicts of Interest: All authors have completed the ICMJE uniform disclosure form (available at https://qims.amegroups.com/article/view/10.21037/qims-22-888/coif). The authors have no conflicts of interest to declare.
Ethical Statement: The authors are accountable for all aspects of the work in ensuring that questions related to the accuracy or integrity of any part of the work are appropriately investigated and resolved. The study was conducted in accordance with the Declaration of Helsinki (as revised in 2013). This retrospective study was reviewed and approved by the ethics committee of the First Affiliated Hospital of Zhengzhou University. All participants signed informed consent.
Open Access Statement: This is an Open Access article distributed in accordance with the Creative Commons Attribution-NonCommercial-NoDerivs 4.0 International License (CC BY-NC-ND 4.0), which permits the non-commercial replication and distribution of the article with the strict proviso that no changes or edits are made and the original work is properly cited (including links to both the formal publication through the relevant DOI and the license). See: https://creativecommons.org/licenses/by-nc-nd/4.0/.
References
- O'Malley RB, Soloff EV, Coveler AL, Cox DH, Desai N, Busey JM, Valentin GM, Wang CL. Feasibility of wide detector CT perfusion imaging performed during routine staging and restaging of pancreatic ductal adenocarcinoma. Abdom Radiol (NY) 2021;46:1992-2002. [Crossref] [PubMed]
- Wan Y, Hao H, Chen Y, Zhang Y, Yue Q, Li Z. Application of spectral CT combined with perfusion scan in diagnosis of pancreatic neuroendocrine tumors. Insights Imaging 2022;13:145. [Crossref] [PubMed]
- Wan Y, Hao H, Meng S, Li Z, Yu F, Chi Meng, Chao Q, Gao J. Application of low dose pancreas perfusion CT combined with enhancement scanning in diagnosis of pancreatic neuroendocrine tumors. Pancreatology 2021;21:240-5. [Crossref] [PubMed]
- Yadav AK, Sharma R, Kandasamy D, Pradhan RK, Garg PK, Bhalla AS, Gamanagatti S, Srivastava DN, Sahni P, Upadhyay AD, Perfusion CT. Can it resolve the pancreatic carcinoma versus mass forming chronic pancreatitis conundrum? Pancreatology 2016;16:979-87. [Crossref] [PubMed]
- Klauss M, Stiller W, Pahn G, Fritz F, Kieser M, Werner J, Kauczor HU, Grenacher L. Dual-energy perfusion-CT of pancreatic adenocarcinoma. Eur J Radiol 2013;82:208-14. [Crossref] [PubMed]
- Tian C, Xu X. Multislice Spiral Perfusion Computed Tomography to Assess Pancreatic Vascularity in Mild Acute Pancreatitis. J Comput Assist Tomogr 2017;41:284-8. [Crossref] [PubMed]
- Smeets XJNM, Litjens G, Gijsbers K, Prokop M, Drenth JPH, Hermans J, van Geenen EJM. The Accuracy of Pancreatic Perfusion Computed Tomography and Angiography in Predicting Necrotizing Pancreatitis: A Systematic Review. Pancreas 2018;47:667-74. [Crossref] [PubMed]
- Miles KA, Hayball MP, Dixon AK. Measurement of human pancreatic perfusion using dynamic computed tomography with perfusion imaging. Br J Radiol 1995;68:471-5. [Crossref] [PubMed]
- Lu N, Feng XY, Hao SJ, Liang ZH, Jin C, Qiang JW, Guo QY. 64-slice CT perfusion imaging of pancreatic adenocarcinoma and mass-forming chronic pancreatitis. Acad Radiol 2011;18:81-8. [Crossref] [PubMed]
- Zhu L, Li JY, Zhang YM, Kang HX, Chen H, Su H, Li J, Tang WF. Pharmacokinetics and pharmacodynamics of Shengjiang decoction in rats with acute pancreatitis for protecting against multiple organ injury. World J Gastroenterol 2017;23:8169-81. [Crossref] [PubMed]
- Vitali F, Pfeifer L, Janson C, Goertz RS, Neurath MF, Strobel D, Wildner D. Quantitative perfusion analysis in pancreatic contrast enhanced ultrasound (DCE-US): a promising tool for the differentiation between autoimmune pancreatitis and pancreatic cancer. Z Gastroenterol 2015;53:1175-81. [Crossref] [PubMed]
- Kandel S, Kloeters C, Meyer H, Hein P, Hilbig A, Rogalla P. Whole-organ perfusion of the pancreas using dynamic volume CT in patients with primary pancreas carcinoma: acquisition technique, post-processing and initial results. Eur Radiol 2009;19:2641-6. [Crossref] [PubMed]
- Benz MR, Szucs-Farkas Z, Froehlich JM, Stadelmann G, Bongartz G, Bouwman L, Schindera ST. Scan time adapted contrast agent injection protocols with low volume for low-tube voltage CT angiography: An in vitro study. Eur J Radiol 2017;93:65-9. [Crossref] [PubMed]
- Tang H, Yu N, Jia Y, Yu Y, Duan H, Han D, Ma G, Ren C, He T. Assessment of noise reduction potential and image quality improvement of a new generation adaptive statistical iterative reconstruction (ASIR-V) in chest CT. Br J Radiol 2018;91:20170521. [Crossref] [PubMed]
- Kim HG, Lee HJ, Lee SK, Kim HJ, Kim MJ, Head CT. Image quality improvement with ASIR-V using a reduced radiation dose protocol for children. Eur Radiol 2017;27:3609-17. [Crossref] [PubMed]
- Bernard A, Comby PO, Lemogne B, Haioun K, Ricolfi F, Chevallier O, Loffroy R. Deep learning reconstruction versus iterative reconstruction for cardiac CT angiography in a stroke imaging protocol: reduced radiation dose and improved image quality. Quant Imaging Med Surg 2021;11:392-401. [Crossref] [PubMed]
- Kwon H, Cho J, Oh J, Kim D, Cho J, Kim S, Lee S, Lee J. The adaptive statistical iterative reconstruction-V technique for radiation dose reduction in abdominal CT: comparison with the adaptive statistical iterative reconstruction technique. Br J Radiol 2015;88:20150463. [Crossref] [PubMed]
- Weir VJ, Zhang J. Radiation Dose Measurements in a 256-Slice Computed Tomography Scanner. J Med Phys 2018;43:85-92. [PubMed]
- Cao G, Zhou X, Xu H, Pan K, Chen W, Li R. Optimizing scan protocol in whole-brain vessel one-stop examination with 640-multislice computed tomography. Zhonghua Yi Xue Za Zhi 2014;94:1609-12. [PubMed]
- Nakaura T, Kidoh M, Nakamura S, Doi Y, Shiraishi S, Awai K, Harada K, Yamashita Y. Low-dose abdominal CT protocols with a tube voltage setting of 100 kVp or 80 kVp: Performance of radiation dose reduction and influence on visual contrast. Clin Radiol 2014;69:804-11. [Crossref] [PubMed]
- Othman AE, Afat S, Brockmann MA, Nikoubashman O, Brockmann C, Nikolaou K, Wiesmann M. Radiation dose reduction in perfusion CT imaging of the brain: A review of the literature. J Neuroradiol 2016;43:1-5. [Crossref] [PubMed]
- Zeng Y, Geng D, Zhang J. Noise-optimized virtual monoenergetic imaging technology of the third-generation dual-source computed tomography and its clinical applications. Quant Imaging Med Surg 2021;11:4627-43. [Crossref] [PubMed]