Quantification of epicardial fat volume using low-dose cardiac scan in patients with low calcium score
Introduction
Epicardial adipose tissue (EAT), a visceral thoracic adipose tissue located in the pericardium, is a biochemically and metabolically active tissue. The primary function of EAT is to store lipids as energy reserves. However, EAT also has an immune function, releasing hormones and inflammatory cytokines that have inflammatory effects on coronary arteries (1). Under pathological conditions in humans, EAT recruits inflammatory cells and induces apoptosis in intraplaque smooth muscle cells through a series of pathways. These pathways promote the formation of large lipid pools, thin fibrous caps, and punctate calcifications as well as lead to an increase in plaque volume. This gradually increases the rate of positive remodeling, eventually resulting in the formation of vulnerable plaques (2,3). The sudden rupture and shedding of vulnerable plaques is the main cause of several acute coronary syndromes, such as unstable angina and acute myocardial infarction, and even sudden death (4).
The American Heart Association recommends the use of statins for treating patients with a medium-to-high coronary artery calcium score (CACS) of ≥100 AU. However, for patients with low-to-mild calcification (CACS <100 AU), no definite guidelines have been recommended yet, and treatment depends on the individual status of the patient (5,6). Because patients with vulnerable plaques in coronary arteries often have mild calcification scores (CACS <100 AU), their symptoms may not receive the timely attention of their physicians, eventually resulting in the development of acute coronary syndrome.
Recently, EAT volume (EATV) has been recognized as an independent predictor of vulnerable plaques in coronary arteries and is believed to have strong predictive ability (3,7). Multislice spiral computed tomography (CT) has become the preferred method for measuring EATV because of its high spatial resolution, high scanning speed, and reproducibility (8,9). Most studies on the association between EATV and coronary arteriosclerosis have relied on coronary CT angiography (CTA) (10,11). However, the large dosage of radiation and the requirement for injection of contrast agents are major concerns for coronary CTA. Recently, low-dose CT has been widely used to screen for diseases of the lung and other organs. It provides high spatial resolution, which is adequate to resolve small features of pericardial fat, without using radiographic contrast agents (12,13). We hypothesized that EATV can be measured using low-dose cardiac nonenhanced scan (EATVcardiac scan), with accuracy and validity comparable to those of EATV measured using CTA (EATVCTA), making the method a viable alternative diagnostic tool for patients with mild calcification.
To address this hypothesis, we compared the accuracies of low-dose cardiac scan and coronary CTA for the quantification of EATV. Low-dose cardiac scan refers to the application of a low radiation dose during cardiac scan using a coronary calcium integral scan scheme for continuous transverse scans. We also evaluated the clinical utility of EATVcardiac scan in predicting coronary heart disease in patients with low-to-mild calcification (CACS <100 AU). The application of this technology will enable the early clinical evaluation of plaques and their stabilization using interventional therapy, thereby greatly reducing the occurrence of acute coronary syndrome. We present the following article in accordance with the STRAD reporting checklist (available at https://qims.amegroups.com/article/view/10.21037/qims-22-664/rc).
Methods
Patients and sampling criteria
The study was conducted in accordance with the Declaration of Helsinki (as revised in 2013). The study was approved by Ethics Committee of the Fifth Affiliated Hospital of Southern Medical University and individual consent for this retrospective analysis was waived. In this study, we retrospectively screened 266 patients with clinical signs (chest pain, epigastric pain, or shortness of breath) of coronary heart disease and low-to-mild calcification (CACS <100 AU) who consecutively underwent low-dose cardiac scan and coronary CTA between June 1, 2020, and December 31, 2021. All scans were performed at the Department of Imaging Diagnostics of the Fifth Affiliated Hospital of Southern Medical University. The following patients were excluded from this study: (I) patients who were pregnant or lactating (n=1); (II) had previously undergone cardiac surgery (n=24), including coronary stent implantation, coronary artery bypass surgery, or pacemaker implantation; (III) had a history of other severe heart diseases (n=15), including valve disease, cardiomyopathy, pericardial effusion, or myocardial infarction; (IV) had an allergy to contrast medium (n=1); (V) had severe hepatic and renal insufficiency (n=4); (VI) had severe thyroid disease (n=1); (VII) had severe arrhythmia (n=3); or (VIII) had scans of poor image quality that interfered with coronary artery assessment (n=13). The remaining 204 patients [aged 32–90 years; 100 (48%) men] were enrolled in this study. The patients’ selection was shown in the Figure S1.
As clinically indicated, written informed consent was obtained from all patients to undergo CT scan and CAT, and individual consent for this retrospective analysis was waived. All data for this study were analyzed anonymously.
CT scans
All scans were performed using a Philips Brilliance 256-slice iCT scanner. The resting heart rate of the patients should be <80 beats/min before the examination. Patients with an elevated heart rate (>80 beats/min) were given metoprolol (25 mg) 60 min before the examination. All patients were sublingually administered with nitroglycerin (0.5 mg) 5 min before the examination dilate the coronary arteries.
The low-dose cardiac scan was performed using a coronary calcium integral scan scheme for continuous transverse scans combined with retrospective electrocardiogram (ECG) gating. Data were collected during the 60–80 RR period. The scanning parameters were as follows: tube voltage of 120 kV, tube current of 50 mA, and gantry rotation time of 0.35 s/360°. The slice thickness was 2.5 mm, and the reconstruction interval was 2.5 mm. The scanning range extended from the tracheal bifurcation to the cardiac septum, with a scan time of 0.7 s, matrix of 512×512, and field of view (FOV) of 250 mm. Based on the dose-length product, the mean effective radiation dose was determined as 0.8 mSv in each patient.
The CTA was generally applied in patients immediately after the low-dose cardiac scan. For CTA, we used a retrospective ECG-gated scanning method. The scanning parameters were as follows: tube voltage of 120 kV, tube current of 550 mA, detector spacing of 128 × 0.625 mm, slice thickness of 0.90 mm, spacing of 0.45 mm, pitch of 0.18, and FOV of 250.0 mm, matrix of 512×512, and iDose4 levels of 3°–4°. We adjusted the tube current, tube voltage, iDose4 level, cardiac convolution function, contrast agent volume, and flow rate according to the patient’s body mass index (BMI). Based on the dose–length product, the mean effective radiation dose was estimated to be 8 mSv in each patient. The scanning range extended up to the tracheal carina and down to the top of the left hepatic lobe. The contrast agent iopromide (370 mg/mL) was injected via the antecubital vein at a rate of 5 mL/sand total dose of 50–60 mL using a 20G intravenous indwelling needle of a double-barrel high-pressure syringe. After injecting the contrast agent, 30 mL of normal saline was injected with the same proportion. We used automatic tracking scanning technology and considered the ascending aortic root as the observation area under a trigger threshold of 110 HU.
Using the contrast medium tracking technology, 8 seconds after the contrast medium injection, we start monitoring the CT value at the aortic root every 1.1 seconds. When the CT value in the sensing area reaches or exceeds the threshold of 110 HU, 6 seconds later, we started the coronary scan of the heart. During the scan process, ECG gating equipment was used to monitor patient heart rate changes.
Soft plaque classification
Based on the 18-segment model of the Society of Cardiovascular Computed Tomography (14), we used CTA to measure the number of plaques in 18 coronary arteries. Two board-certified radiologists with 7 and 10 years of experience, respectively, independently evaluated the results of cardiac scans using curved multiplanar reconstructions and cross-sectional images. Any discrepancies were discussed and resolved. Using CTA scans, we assessed atherosclerotic plaques in the coronary arteries of all patients. Based on their physical characteristics, we used the following four degrees of calcification: dense calcium plaque (density of >350 HU), fibrous plaque (density of 131–350 HU), fibrofatty plaque (density of 31–130 HU), and necrotic core plaque (density of −30 to 30 HU) (15). Patients were categorized into the plaque group if any of these plaques was detected in the coronary artery, whereas those without any plaques were included in the nonplaque group.
High-risk coronary plaques exhibited one or more of the following four morphological characteristics: low-attenuation density (with average plaque attenuation of <30 HU and area of >1 mm2), positive remodeling plaque (with a ratio of the diameter of plaque to the mean of proximal and distal normal diameters of >1.1), spotty calcification (showing focal calcification within the coronary artery wall, with a maximum diameter of <3 mm in any direction), and napkin ring sign (with low-attenuation density of <30 HU in the central area of the plaque and slightly higher density, which was <130 HU, in the periphery). Patients with coronary plaques exhibiting two or more high-risk morphological features were defined as having vulnerable plaques (6,15).
EATV measurement
We measured and analyzed EATV using Philips workstation Volumer software. The threshold density for adipose tissue was set at −190 to 30 HU. The measurement ranged from the origin of the left pulmonary artery at the midpoint of the transverse pericardium to the lower edge of the left ventricular apex. The software automatically identified adipose tissue, obtained a three-dimensional image of epicardial fat, and then calculated its volume (14) (Figure 1). Both the assessors of the low-dose cardiac scan and the assessors of the CTA were unavailable to the clinical information of the patients. Also, the assessors of the low-dose cardiac scan did not know the CTA results.
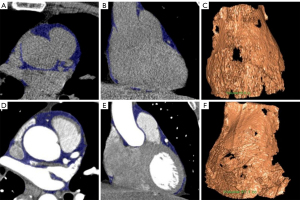
Considering the allergy to contrast agent for several patients, all patients would sign a content to the CAT measure and had a skin test of contrast agent before CTA.
CACS
In accordance with the Agatston score, we defined the total calcium score as the sum of the scores of each coronary artery. We used the following four categories of CACS: no calcification (CACS =0), slight calcification (1≤ CACS<100), mild-to-moderate calcification (100≤ CACS <400), and severe calcification (CACS ≥400) (16).
Statistical analysis
Continuous variables are represented as the mean ± standard deviation or median (25th and 75th quartiles), as appropriate. Student’s t-test or Wilcoxon test was used to compare data between groups. Categorical variables are represented as frequency and percentages. We used chi-square test to determine differences between two groups. Pearson correlation coefficient was used to evaluate the strength of correlation between EATVcardiac scan and EATVCTA. Bland-Altman test was performed to determine consistency between these two measurements. A multiple logistic regression model was used to identify the potential predictors of coronary and vulnerable plaques. The receiver operating characteristic (ROC) curve was constructed to evaluate the predictive efficacy of EATVcardiac scan and EATVCTA for coronary and vulnerable plaques. Furthermore, the areas under the curve (AUCs) were calculated and compared for the two EATV measurements.
All statistical analyses were performed using statistical software packages SPSS (version 25.0; IBM Corp.) and MedCalc (version 18; MedCalc Software). A P value of <0.05 was considered to indicate statistical significance.
Results
In total, 204 patients with suspected coronary heart disease underwent low-dose CT and coronary CTA to obtain EATVcardiac scan and EATVCTA, respectively (Table 1). Among all patients, 126 (61.8%) were diagnosed with coronary plaques; they were more likely to be women and were older than those without plaques (P<0.05 for both comparisons). Patients with coronary plaques had a more prevalent history of hypertension (P=0.893) and lower hemoglobin levels (P=0.359) than those without plaques; however, the difference was not statistically significant. Further, patients with plaques had significantly higher EATVcardiac scan and EATVCTA than those without plaques (P<0.001 for both). Moreover, 54% [68] of patients with plaques showed vulnerable plaques. However, no significant differences were observed in sex (P=0.164); age (P=0.863); BMI (P=0.313); laboratory measurements (all P values >0.05); or history of hypertension (P=0.893), diabetes (P=0.181), or dyslipidemia (P=0.893) between patients with vulnerable and nonvulnerable plaques.
Table 1
Characteristics | Without plaque (n=78) | Plaque (n=126) | P value | Vulnerable plaque (n=68) | Non-vulnerable plaque (n=58) | P value |
---|---|---|---|---|---|---|
EATVCardiac scan (cm3) | 86.46±26.60 | 110.83±46.27 | <0.001* | 111.38±36.91 | 110.18±55.62 | 0.889 |
EATVCTA (cm3) | 89.03±26.08 | 114.18±45.97 | <0.001* | 114.53±39.11 | 113.77±53.25 | 0.929 |
Females | 26 (33.33) | 72 (57.14) | 0.001* | 35 (51.47) | 37 (63.79) | 0.164 |
Age, year | 52.01±10.06 | 64.53±11.16 | <0.001* | 64.69±11.28 | 64.34±11.10 | 0.863 |
BMI (kg/m2) | 24.38±3.62 | 23.93±5.48 | 0.523 | 23.48±4.17 | 24.47±6.70 | 0.313 |
Hemoglobin (g/L) | 135.26±20.92 | 129.74±19.27 | 0.056 | 131.21±17.43 | 128.03±21.26 | 0.359 |
Total cholesterol (mmol/L) | 4.85±1.13 | 4.79± 1.17 | 0.719 | 4.84±1.10 | 4.72±1.26 | 0.569 |
LDL (mmol/L) | 2.77±0.97 | 2.82 ±1.03 | 0.717 | 2.85±1.01 | 2.78±1.06 | 0.671 |
HDL (mmol/L) | 1.25±0.32 | 1.23±0.36 | 0.626 | 1.22±0.35 | 1.24±0.37 | 0.720 |
TG (mmol/L) | 1.88±1.09 | 1.93±1.85 | 0.825 | 1.96±1.57 | 1.90±2.16 | 0.852 |
Hypertension | 39 (50.00) | 79 (62.70) | 0.074 | 43 (63.24) | 36 (62.07) | 0.893 |
Diabetes | 11 (14.10) | 26 (20.63) | 0.239 | 11 (16.18) | 15 (25.86) | 0.181 |
Dyslipidemia | 46 (58.97) | 79 (62.70) | 0.596 | 43 (63.24) | 36 (62.07) | 0.893 |
Data are expressed as mean ± standard deviation or n (%). *P<0.05. EATVCardiacscan, low-dose cardia plain scan to examine epicardial fat volume; EATVCTA, epicardial fat volume was quantified by coronary CTA; BMI, body mass index; LDL, low density lipid; HDL, high density lipid; TG, triglyceride.
The two EATV measurements, EATVcardiac scan and EATVCTA, were strongly correlated with each other among all patients (r=0.960, P<0.001; Figure 2A), particularly among patients with coronary plaques (r=0.952, P<0.001; Figure 2B). Bland–Altman plots (Figure 2C) revealed that the mean difference between EATVcardiac scan and EATVCTA was −3.05 among all patients, and only a small number of patients (10/204, 4.9%) had values outside the 95% confidence interval (CI) range (−26.15 to 20.04, P for agreement =0.0003). A similar significant agreement was observed between EATVcardiac scan and EATVCTA among patients with plaques, with an estimated difference of −3.354, and 6.35% (8/126) of patients had values outside the 95% CI range (−31.37 to 24.66, P for agreement =0.0095) (Figure 2D).
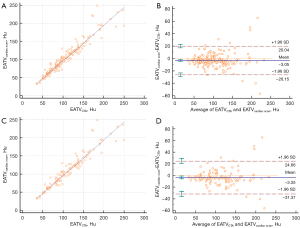
The results of univariate and multivariate logistic regression models revealed a significant association of EATVcardiac scan (P<0.001) and EATVCTA (P<0.001) with coronary plaques (Table 2). After adjustment for age and sex, the odds ratio for coronary plaques (Table 2) was 1.019 (95% CI: 1.010–1.029; P<0.001) and 1.020 (95% CI: 1.011–1.030; P<0.001), respectively. However, among patients with plaques, neither of the two EATV measurements was significantly associated with vulnerable plaques (P=0.903 for EATVCardiac scan and P=0.932 for EATVCTA; Table 3). The results of ROC analysis were consistent with those of logistic regression. In particular, the ROC curves revealed that EATVcardiac scan and EATVCTA were predictors of coronary plaques in patients with suspected plaques; however, we could not distinguish patients with vulnerable plaques from those with nonvulnerable plaques (Figure 3). The AUC of EATVcardiac scan for coronary and vulnerable plaques was 0.662 and 0.550, respectively, whereas that of EATVCTA was 0.670 and 0.530, respectively. However, EATVcardiac scan and EATVCTA showed similar inefficiency in diagnosing coronary plaques (P=0.433) and distinguishing vulnerable plaques (P=0.216).
Table 2
Risk factors | Crude model | Model I | Model II | |||||
---|---|---|---|---|---|---|---|---|
OR (95% CI) | P value | OR (95% CI) | P value | OR (95% CI) | P value | |||
EATVCardiac scan (cm3) | 1.018 (1.009–1.027) | <0.001* | 1.019 (1.010–1.029) | <0.001* | – | – | ||
EATVCTA (cm3) | 1.019 (1.009–1.028) | <0.001* | – | – | 1.020 (1.011–1.030) | <0.001* | ||
Females | 2.667 (1.481–4.803) | 0.001* | 2.986 (1.599–5.577) | 0.001* | 2.980 (1.591–5.580) | 0.001* | ||
Age, year | 1.114 (1.077–1.152) | <0.001* | 1.137 (1.090–1.186) | <0.001* | 1.137 (1.090–1.187) | <0.001* |
Model I and model II: adjusted for age and gender. *P<0.05. CI, confidence interval; EATVcardiacscan: low-dose cardia plain scan to examine epicardial fat volume, EATVCTA, epicardial fat volume was quantified by coronary CTA.
Table 3
Risk factors | Crude model | Model I | Model II | |||||
---|---|---|---|---|---|---|---|---|
OR (95% CI) | P value | OR (95% CI) | P value | OR (95% CI) | P value | |||
EATVCardiac scan (cm3) | 0.999 (0.992–1.007) | 0.884 | 0.999 (0.991–1.008) | 0.903 | – | – | ||
EATVCTA (cm3) | 1.000 (0.992–1.007) | 0.926 | – | – | 1.000 (0.992–1.008) | 0.932 | ||
Females | 1.661 (0.812–3.400) | 0.165 | 1.681 (0.807–3.504) | 0.166 | 1.681 (0.807–3.505) | 0.166 | ||
Age, year | 0.997 (0.966–1.029) | 0.862 | 1.003 (0.969–1.038) | 0.868 | 1.003 (0.969–1.038) | 0.878 |
Model I and model II: adjusted for age and gender. *P<0.05. CI, confidence interval; EATVCardiac scan, low-dose cardia plain scan to examine epicardial fat volume; EATVCTA, epicardial fat volume was quantified by coronary CTA.
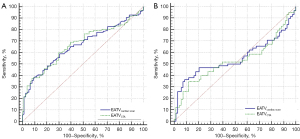
Discussion
EATV measurement using coronary CTA for predicting coronary heart disease has gained nationwide popularity in recent years. However, this use of CTA remains limited because of the side effects of radiation and contrast media (17). Our retrospective analysis of dually scanned patients provides quantitative evidence with an intrinsic control that EATV measurements using low-dose cardiac scans and CTA were consistent, equally accurate, and significantly and strongly correlated in patients with low-to-mild calcification (CACS <100 AU). This finding suggests that low-dose cardiac scans can be used as a preferred alternative to coronary CTA for diagnosing coronary plaques in patients with mild coronary calcification.
Recently, several studies have used non-CTA methods to measure EATV (14,18,19). Accordingly, we used low-dose chest CT scans to measure EATV and found that these values were significantly and strongly correlated with those measured using CTA. Our result is consistent with that of the study by Cheng et al., who retrospectively evaluated the efficacy of noncontrast CT scans for EATV measurements in 40 Chinese patients and revealed that these values were strongly correlated with those measured using CTA (19). Similar evidence regarding the associations between EATV and the progression of coronary artery calcification has been reported (15,20,21). However, these investigators used conventional doses during cardiac scans. Furthermore, a study involving 117 patients in Nagayama, Japan (14), revealed a close agreement between EATV measured using ECG noncontrast CT and that measured using CTA. However, in a study measuring pericardial fat area around the left and right main coronary artery initial segments using low-dose chest CT (18), the authors reported a modest correlation (r<0.73) between the pericardial fat area and epicardial fat volume in both segments. Our findings suggest that compared with CTA, a low-dose cardiac scan in patients with a low calcium score provides a clinical diagnosis with reduced radiation dose but similar diagnostic efficiency.
Quantification of EATV using coronary CTA has become a recognized method. However, several investigators believe that EATV measured using this technique underestimates the real volume because of the volume effect between the high-density coronary contrast agent and the low-density fat shadow around the coronary arteries (14). Even under a low-dose condition (with a tube voltage of 120 kV and tube current of 50 mA), different tube voltages could show differences in estimated fat density around the coronary arteries (22,23), which makes it impossible to exclude the possibility of EATV underestimation. Thus, these two types of CT imaging techniques have the potential to underestimate EATV.
Low-dose chest CT has become an important method for the early diagnosis of chest diseases (12,13), with a growing popularity because of limited patient exposure to radiation (24,25). Among our patients with mild calcification (CACS <100 AU), we revealed that the EATV measured using both low-dose cardiac scan and coronary CTA were independently associated with coronary arteriosclerosis. Vascular inflammation is a key factor in atherosclerotic plaque formation and plaque rupture (26). It has been reported that EAT with a larger volume contains more immature adipocytes, which exacerbate the inflammation of the vessel wall and instability of intimal plaques by releasing inflammatory factors (3,27), resulting in acute coronary syndrome. Both Gao et al. [2022] and Nerlekar et al. [2017] (3,28) reported that EATV was significantly associated with vulnerable plaques. Notably, among our patients with mild calcification (CACS <100 AU), the proportion of patients with vulnerable plaques was higher than that of patients with nonvulnerable plaques. Although this difference was not statistically significant, these patients were more likely to develop acute coronary syndrome. A previous study suggests that vulnerable plaques increase the risk of major adverse cardiac events by 4–5-fold (29). In this study, EATV measured using both low-dose cardiac scan and CTA had equivalent predictive value for coronary arteriosclerosis. Moreover, we found no significant difference in AUC between the two methods.
Although the quantification of EATV using coronary CTA has been widely recognized, other methods are in their initial stage. Considering the increasing attention and benefits of CT using a low radiation dose and low contrast agent, we believe that the measurement of EATV using this method is useful for the early clinical treatment and stabilization of plaques, particularly in patients with mild calcification (CACS <100 AU).
This study has several limitations. First, for measuring EATV, the same adipose threshold (−190 to −30 HU) was set for both low-dose EATV cardiac scan and coronary CTA. This choice might have introduced a bias by measuring lower values using low-dose cardiac scan. Several researchers have already used the ordered subset expectation maximization algorithm to improve the quality of low-dose CT images (30), thereby providing an approach to improve the accuracy of the total EATV measurements. Second, various studies have indicated the correlation between pericoronary adipose tissue (PCAT) and coronary arteriosclerosis (3,16,31). The current study is a part of a series of future studies in which we plan to combine EATV and PCAT to detect vulnerable coronary plaques. Third, the accurate measurement of pericardial adipose volume currently requires a long time in manually controlled offline analysis. To shorten the measurement time in the future, the continuous advancement of artificial intelligence applications in the field of medical imaging may provide promising alternatives to current practices (32). Finally, this study is a single-center study with a small sample size; thus, the findings warrant further evaluation with larger sample sizes.
EATV can be measured using low-dose cardiac scans with accuracy comparable to that of coronary CTA. In addition, the two methods provide comparable values for predicting coronary arteriosclerosis in patients with low-to-mild calcification (CACS <100 AU).
Acknowledgments
Funding: This work was supported by Medical Scientific Research Foundation of Guangdong Province, China (grant No. B2020223).
Footnote
Reporting Checklist: The authors have completed the STARD reporting checklist. Available at https://qims.amegroups.com/article/view/10.21037/qims-22-664/rc
Conflicts of Interest: All authors have completed the ICMJE uniform disclosure form (available at https://qims.amegroups.com/article/view/10.21037/qims-22-664/coif). All authors report that this work was supported by Medical Scientific Research Foundation of Guangdong Province, China (grant No. B2020223). The authors have no other conflicts of interest to declare.
Ethical Statement: The authors are accountable for all aspects of the work in ensuring that questions related to the accuracy or integrity of any part of the work are appropriately investigated and resolved. The study was conducted in accordance with the Declaration of Helsinki (as revised in 2013). The study was approved by Ethics Committee of the Fifth Affiliated Hospital of Southern Medical University and individual consent for this retrospective analysis was waived.
Open Access Statement: This is an Open Access article distributed in accordance with the Creative Commons Attribution-NonCommercial-NoDerivs 4.0 International License (CC BY-NC-ND 4.0), which permits the non-commercial replication and distribution of the article with the strict proviso that no changes or edits are made and the original work is properly cited (including links to both the formal publication through the relevant DOI and the license). See: https://creativecommons.org/licenses/by-nc-nd/4.0/.
References
- Senoo A, Kitagawa T, Torimaki S, Yamamoto H, Sentani K, Takahashi S, Kubo Y, Yasui W, Sueda T, Kihara Y. Association between histological features of epicardial adipose tissue and coronary plaque characteristics on computed tomography angiography. Heart Vessels 2018;33:827-36. [Crossref] [PubMed]
- Mazurek T, Kochman J, Kobylecka M, Wilimski R, Filipiak KJ, Królicki L, Opolski G. Inflammatory activity of pericoronary adipose tissue may affect plaque composition in patients with acute coronary syndrome without persistent ST-segment elevation: preliminary results. Kardiol Pol 2014;72:410-6. [Crossref] [PubMed]
- Gao Z, Zuo Y, Jia L, Yin Y, Yang X, Fan Y, Liu H. Association between epicardial adipose tissue density and characteristics of coronary plaques assessed by coronary computed tomographic angiography. Int J Cardiovasc Imaging 2022;38:673-81. [Crossref] [PubMed]
- Oikonomou EK, Marwan M, Desai MY, Mancio J, Alashi A, Hutt Centeno E, et al. Non-invasive detection of coronary inflammation using computed tomography and prediction of residual cardiovascular risk (the CRISP CT study): a post-hoc analysis of prospective outcome data. Lancet 2018;392:929-39. [Crossref] [PubMed]
- Grundy SM, Stone NJ, Bailey AL, Beam C, Birtcher KK, Blumenthal RS, et al. 2018 AHA/ACC/AACVPR/AAPA/ABC/ACPM/ADA/AGS/APhA/ASPC/NLA/PCNA Guideline on the Management of Blood Cholesterol: A Report of the American College of Cardiology/American Heart Association Task Force on Clinical Practice Guidelines. J Am Coll Cardiol 2019;73:e285-350. [Crossref] [PubMed]
- Senoner T, Plank F, Langer C, Beyer C, Steinkohl F, Barbieri F, Adukauskaite A, Widmann G, Friedrich G, Dichtl W, Feuchtner GM. Smoking and obesity predict high-risk plaque by coronary CTA in low coronary artery calcium score (CACS). J Cardiovasc Comput Tomogr 2021;15:499-505. [Crossref] [PubMed]
- Tan Y, Zhou J, Zhou Y, Yang X, Wang J, Chen Y. Epicardial adipose tissue is associated with high-risk plaque feature progression in non-culprit lesions. Int J Cardiovasc Imaging 2017;33:2029-37. [Crossref] [PubMed]
- Taha DA, El Shafey RAA, Hamesa MF, Abu-Dewan KAE-W, Nagy HA. Relationship between epicardial fat volume measured by multi-detector computed tomography and coronary artery disease. Egyptian Journal of Radiology and Nuclear Medicine 2021;52:1-16. [Crossref]
- Li H, Zhao R, Li X, Ding C, Wang T, Yu C, Wang W, Wang H, Liu B. Relationship of epicardial adipose tissue volume with coronary atherosclerosis and myocardial bridge. Chinese Journal of Medical Imaging Technology 2020:64-7.
- Du Y, Yang L, Liu Y, Yang B, Lv S, Hu C, Zhu Y, Zhang H, Ma Q, Wang Z, Liu Y, Shi D, Zhao Y, Xu L, Zhou Y. Relation between quantity and quality of peri-coronary epicardial adipose tissue and its underlying hemodynamically significant coronary stenosis. BMC Cardiovasc Disord 2020;20:226. [Crossref] [PubMed]
- Weng J, Zeng Q, Tian T, He Y, Yu F, Huo R, Bai X, He Q. Correlation Between the Pericaridal Fat Volume and Coronary Artery Disease by CT Coronary Angiography. Journal of Clinical Radiology 2021;40:1307-11.
- Hwang EJ, Goo JM, Kim HY, Yi J, Kim Y. Optimum diameter threshold for lung nodules at baseline lung cancer screening with low-dose chest CT: exploration of results from the Korean Lung Cancer Screening Project. Eur Radiol 2021;31:7202-12. [Crossref] [PubMed]
- Erkmen CP, Dako F, Moore R, Dass C, Weiner MG, Kaiser LR, Ma GX. Adherence to annual lung cancer screening with low-dose CT scan in a diverse population. Cancer Causes Control 2021;32:291-8. [Crossref] [PubMed]
- Nagayama Y, Nakamura N, Itatani R, Oda S, Kusunoki S, Takahashi H, Nakaura T, Utsunomiya D, Yamashita Y. Epicardial fat volume measured on nongated chest CT is a predictor of coronary artery disease. Eur Radiol 2019;29:3638-46. [Crossref] [PubMed]
- Shaw LJ, Blankstein R, Bax JJ, Ferencik M, Bittencourt MS, Min JK, et al. Society of Cardiovascular Computed Tomography / North American Society of Cardiovascular Imaging - Expert Consensus Document on Coronary CT Imaging of Atherosclerotic Plaque. J Cardiovasc Comput Tomogr 2021;15:93-109. [Crossref] [PubMed]
- Ma R, van Assen M, Ties D, Pelgrim GJ, van Dijk R, Sidorenkov G, van Ooijen PMA, van der Harst P, Vliegenthart R. Focal pericoronary adipose tissue attenuation is related to plaque presence, plaque type, and stenosis severity in coronary CTA. Eur Radiol 2021;31:7251-61. [Crossref] [PubMed]
- Andreucci M, Solomon R, Tasanarong A. Side effects of radiographic contrast media: pathogenesis, risk factors, and prevention. Biomed Res Int 2014;2014:741018. [Crossref] [PubMed]
- Lee KC, Yong HS, Lee J, Kang EY, Na JO. Is the epicardial adipose tissue area on non-ECG gated low-dose chest CT useful for predicting coronary atherosclerosis in an asymptomatic population considered for lung cancer screening? Eur Radiol 2019;29:932-40. [Crossref] [PubMed]
- Cheng K, Zha Y, Hu L, Zeng F, Peng Z. A comparative study of Non-contrast Cardiac CT and Coronary CT Angiography in Quantitative Analysis of Epicardial Fat Volume. Computerized Tomography Theory and Applications 2019;28:585-91.
- Nakanishi R, Rajani R, Cheng VY, Gransar H, Nakazato R, Shmilovich H, Otaki Y, Hayes SW, Thomson LE, Friedman JD, Slomka PJ, Berman DS, Dey D. Increase in epicardial fat volume is associated with greater coronary artery calcification progression in subjects at intermediate risk by coronary calcium score: a serial study using non-contrast cardiac CT. Atherosclerosis 2011;218:363-8. [Crossref] [PubMed]
- D'Errico L, Salituri F, Ciardetti M, Favilla R, Mazzarisi A, Coppini G, Bartolozzi C, Marraccini P. Quantitative analysis of epicardial fat volume: effects of scanning protocol and reproducibility of measurements in non-contrast cardiac CT vs. coronary CT angiography. Quant Imaging Med Surg 2017;7:326-35. [Crossref] [PubMed]
- Ma R, Ties D, van Assen M, Pelgrim GJ, Sidorenkov G, van Ooijen PMA, van der Harst P, van Dijk R, Vliegenthart R. Towards reference values of pericoronary adipose tissue attenuation: impact of coronary artery and tube voltage in coronary computed tomography angiography. Eur Radiol 2020;30:6838-46. [Crossref] [PubMed]
- Yan C, Liang C, Xu J, Wu Y, Xiong W, Zheng H, Xu Y. Ultralow-dose CT with knowledge-based iterative model reconstruction (IMR) in evaluation of pulmonary tuberculosis: comparison of radiation dose and image quality. Eur Radiol 2019;29:5358-66. [Crossref] [PubMed]
- Chamberlin J, Kocher MR, Waltz J, Snoddy M, Stringer NFC, Stephenson J, Sahbaee P, Sharma P, Rapaka S, Schoepf UJ, Abadia AF, Sperl J, Hoelzer P, Mercer M, Somayaji N, Aquino G, Burt JR. Automated detection of lung nodules and coronary artery calcium using artificial intelligence on low-dose CT scans for lung cancer screening: accuracy and prognostic value. BMC Med 2021;19:55. [Crossref] [PubMed]
- Zhu Y, Wang Y, Gioia WE, Yip R, Jirapatnakul AC, Chung MS, Yankelevitz DF, Henschke CI. Visual scoring of aortic valve calcifications on low-dose CT in lung cancer screening. Eur Radiol 2020;30:2658-68. [Crossref] [PubMed]
- Zhu X, Chen X, Ma S, Zhou K, Hou Y. Dual-layer spectral detector CT to study the correlation between pericoronary adipose tissue and coronary artery stenosis. J Cardiothorac Surg 2021;16:325. [Crossref] [PubMed]
- Raggi P, Gadiyaram V, Zhang C, Chen Z, Lopaschuk G, Stillman AE. Statins Reduce Epicardial Adipose Tissue Attenuation Independent of Lipid Lowering: A Potential Pleiotropic Effect. J Am Heart Assoc 2019;8:e013104. [Crossref] [PubMed]
- Nerlekar N, Brown AJ, Muthalaly RG, Talman A, Hettige T, Cameron JD, Wong DTL. Association of Epicardial Adipose Tissue and High-Risk Plaque Characteristics: A Systematic Review and Meta-Analysis. J Am Heart Assoc 2017; [Crossref] [PubMed]
- Ferencik M, Mayrhofer T, Bittner DO, Emami H, Puchner SB, Lu MT, Meyersohn NM, Ivanov AV, Adami EC, Patel MR, Mark DB, Udelson JE, Lee KL, Douglas PS, Hoffmann U. Use of High-Risk Coronary Atherosclerotic Plaque Detection for Risk Stratification of Patients With Stable Chest Pain: A Secondary Analysis of the PROMISE Randomized Clinical Trial. JAMA Cardiol 2018;3:144-52. [Crossref] [PubMed]
- Miao Z, Yang H, Liu B, Li W. Correlation analysis of epicardial adipose tissue volume quantified by computed tomography images and coronary heart disease under optimized reconstruction algorithm. Pak J Med Sci 2021;37:1677-81. [Crossref] [PubMed]
- Dai X, Deng J, Yu M, Lu Z, Shen C, Zhang J. Perivascular fat attenuation index and high-risk plaque features evaluated by coronary CT angiography: relationship with serum inflammatory marker level. Int J Cardiovasc Imaging 2020;36:723-30. [Crossref] [PubMed]
- Slart RHJA, Williams MC, Juarez-Orozco LE, Rischpler C, Dweck MR, Glaudemans AWJM, et al. Position paper of the EACVI and EANM on artificial intelligence applications in multimodality cardiovascular imaging using SPECT/CT, PET/CT, and cardiac CT. Eur J Nucl Med Mol Imaging 2021;48:1399-413. [Crossref] [PubMed]