The role of the angiography-derived index of microcirculatory resistance in the prognosis of patients with dilated cardiomyopathy
Introduction
Dilated cardiomyopathy (DCM), which is the third most common cause of heart failure (HF) after coronary artery disease (CAD) and hypertension, is the most frequent form of primary myocardial disease. DCM is characterized by ventricular dilation and systolic dysfunction induced by diverse genetic and non-genetic causes (1). DCM is linked to poor prognosis, and heart transplantation is often required (2). In clinical practice, patients with HF and cardiac enlargement tend to be diagnosed with DCM when other possible etiologies are excluded (e.g., CAD, hypertension, and valve diseases). Coronary angiography is the first choice to identify ischemic heart disease in the diagnostic work-up for the etiology assessment of DCM. Nevertheless, coronary angiography can only determine the ischemic myocardium caused by epicardial coronary artery stenosis, and investigation of microvascular disease is lacking in patients with DCM.
A few studies have demonstrated the presence of coronary microvascular dysfunction (CMD) in patients with DCM (3-7). The degree of CMD also has a direct impact on the prognosis of the patient (3-5). Although several techniques provide superior conditions for assessing CMD, their limitations cannot be ignored. Coronary float velocity reserve (CFVR) assessed using transthoracic doppler echocardiography (TTDE) is typically restricted to the left anterior descending (LAD) artery area, and cardiovascular risks are also elevated with the use of the stress dobutamine examination (3,8,9). Limited availability, associated costs, and lengthy procedure restrict the use of positron emission tomography (PET) and cardiac magnetic resonance (CMR) to evaluate myocardial blood flow (MBF) in routine clinical practice (10).
The index of microvascular resistance (IMR), which is defined as the minimum microcirculatory resistance to peak blood flow through the target vessel, is a quantitative and reproducible measure of CMD. In obtaining this index, the operator simultaneously measures the distal intracoronary pressure (Pd) and the mean conduction time (Tmn) of the intracoronary projectile saline injection by means of a pressure guidewire in a state of maximal filling of the myocardial microcirculation. The IMR value is then obtained by multiplying Pd by Tmn. Adverse events in patients with stable CAD can be predicted with IMR (11,12). However, due to this approaches’ complexity, time-consuming operation, and need for decongestant drugs, a coronary angiography-derived index of microcirculatory resistance (caIMR) has been proposed for the physiological evaluation of CMD. caIMR correlates well with traditional wire-based IMR and can achieve an 85% accuracy in diagnosis (13,14). For patients with ST-segment elevated myocardial infarction in the acute postoperative period, caIMR was shown to be consistent with CMR in assessing microvascular dysfunction (15).
We speculated that caIMR is associated with the outcome of the patients with DCM. Therefore, this diagnostic study was conducted to assess the role of the caIMR in the prognosis of patients with DCM. We present the following article in accordance with the STARD reporting checklist (available at https://qims.amegroups.com/article/view/10.21037/qims-22-1060/rc).
Methods
Study population
This retrospective and diagnostic study enrolled all consecutive patients with DCM in the Department of Cardiology, the First Affiliated Hospital of Nanjing Medical University, Nanjing, China, from January 2013 to January 2018. The inclusion criteria of patients in this study were as follows: (I) clinical evidence of congestion; (II) an elevated level of B-type natriuretic peptide (BNP ≥35 pg/mL) or N-terminal prohormone of B-type natriuretic peptide (NT-proBNP ≥125 pg/mL) (16); (III) echocardiographic left ventricular diameter of diastole (LVDd) >55 mm for males and LVDd >50 mm for females; (IV) reduced left ventricular systolic function defined by left ventricular ejection fraction (LVEF) <45% (17), and (V) normal or near normal coronary artery (<30% diameter stenosis) confirmed by coronary angiography. The exclusion criteria of patients were as follows: (I) a poor coronary angiogram that affected the caIMR calculation, including a blurry coronary angiography image, coronary arteries in fewer than two angiographic positions, or twisted and overlapped coronary arteries; and (II) coronary heart disease, hypertensive heart disease, rheumatic heart valve disease, myocarditis, etc.
The present study complied with the Helsinki Declaration (as revised in 2013) and was endorsed by the local institutional ethics committee of the First Affiliated Hospital of Nanjing Medical University. All patients provided written informed consent.
Measurement of the caIMR
The caIMR (Rainmed Ltd., Suzhou, China) was calculated by a skilled operator who was blind to the clinical data. In order to accurately obtain the length of target vessels, two angiographic images of the target vessels with a projection angle >30° were used to rebuild a 3-dimensional vessel. Invasive aortic blood pressure was reviewed and input into the console. The caIMR was measured with FlashAngio software (Rainmed Ltd.) with a proprietary fluid dynamic algorithm that was described earlier (13). The formula of caIMR calculation was as follows: caIMR = Pdhyp × (L/K × Vdiastole), where Pdhyp is the mean invasive blood pressure, L is the length of the target vessel measured through a 3-dimensional vessel’s model, Vdiastole is the mean coronary flow velocity assessed by the frame counts, and K is a constant (K equals 2.1). The feasibility and high accuracy of caIMR measurement are well-established (16). We also recorded the coronary angiography-derived fractional flow reserve (caFFR) from the FlashAngio system. The evaluation of caFFR was conducted as described in a previous study (18).
End point and follow‑up
The study end point was composite events including HF-related rehospitalization, device implantation, heart transplantation, and cardiac death during the follow-up. Criteria for HF rehospitalization were a significant elevation of BNP levels, typical dyspnea, the presence of wet rales in the lungs, decreased blood oxygen saturation (<95%), and, particularly, the use of intravenous diuretics. Device implantation included cardiac resynchronization therapy (CRT) and CRT with a defibrillator. Patients were followed up through outpatient visits, inpatient chart reviews, and/or telephone interviews. The end point events were confirmed by reviewing medical records or contacting patients, their family members, and/or their physicians.
Statistical analysis
The Kolmogorov-Smirnov test was used to determine the distribution of numerical data. Variables with a normal distribution are presented as the mean ± standard deviation and compared using the independent samples t-test. Variables with skewed distribution are reported as the median with interquartile range (IQR) and were compared using the nonparametric Mann-Whitney test. Categorical variables are calculated using counts and percentages and were assessed using the chi-squared test or Fisher exact test as appropriate. For variables with a normal or nonnormal distribution, the Pearson R correlation coefficients and Spearman rank correlation coefficients were used, respectively, to analyze the correlation between the caIMR among three vessels. Based on the data collection results, the threshold of the caIMR was further explored. The receiver operating curve (ROC) analysis was used to identify the best cutoff value of the caIMR to predict the end points. Then, patients were divided into two groups according to the threshold of the caIMR. The cumulative incidence was estimated with the Kaplan-Meier method and compared between the higher caIMR and lower caIMR groups using the log-rank test. Cox proportional hazards models were adopted to calculate the hazard ratios (HRs) of the caIMR for the primary end point. The covariates in this model included age, NT-proBNP, atrial fibrillation, LVDd, the use of beta-blockers, and the use of angiotensin-converting enzyme inhibitors or angiotensin receptor blockers (ACEI/ARB). Clinical information and end point composite event results were unavailable to those conducting the experiment. A 2-sided P value <0.05 was considered statistically significant. All analyses were performed using R version 4.0.5 (The R Foundation for Statistical Computing, Vienna, Austria) and SPSS 25.0 (IBM Corp, Armonk, NY, USA).
Results
Figure 1 shows the flowchart of this study. A total of 683 patients with DCM were initially screened, and 95 patients satisfied the inclusion criteria and were finally enrolled in this study. During a median follow-up time of 43.2 months, 36 end point events occurred. Patients were then divided into the event group and the nonevent group for comparisons of baseline characteristics.
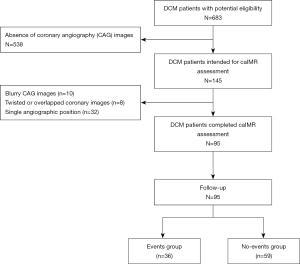
Baseline characteristics of the study population
As shown in Table 1, more patients in the nonevent group used a beta-blocker (89.8% vs. 66.7%; P=0.005) and ACEI/ARB (84.7% vs. 66.7%; P=0.039). Patients in the event group had a higher level of LDL-C (2.99±0.92 vs. 2.63±0.65 mmol/L; P=0.024), caIMR of the LAD artery (31.6±8.9 vs. 21.3±5.7; P<0.001), caIMR of left circumflex (LCX) artery (31.9±10.2 vs. 24.7±10.0; P=0.001), and caIMR of right coronary artery (RCA) (31.8±11.8 vs. 23.4±7.7; P<0.001). No differences were found in age, history of disease, or echocardiographic parameters.
Table 1
Variables | Events group (n=36) | No-events group (n=59) | P value |
---|---|---|---|
Age (years) | 64 [53, 68] | 63 [50, 68] | 0.890 |
Male, n (%) | 30 (83.3) | 43 (72.9) | 0.241 |
Diabetes, n (%) | 3 (8.3) | 11 (18.6) | 0.237 |
Stroke, n (%) | 1 (2.8) | 3 (5.1) | 1.000 |
Atrial fibrillation, n (%) | 11 (30.6) | 9 (15.3) | 0.076 |
Smoker, n (%) | 25 (69.4) | 29 (49.2) | 0.053 |
SBP (mmHg) | 115±17 | 118±16 | 0.345 |
DBP (mmHg) | 74±11 | 70±11 | 0.186 |
NT-proBNP (pg/mL) | 1,286 [766, 2,602] | 1,143 [535, 2,360] | 0.203 |
TC (mmol/L) | 4.55±1.24 | 4.18±0.99 | 0.111 |
LDL-C (mmol/L) | 2.99±0.92 | 2.63±0.65 | 0.024 |
FBG (mmol/L) | 5.07 [4.62, 5.71] | 5.27 [4.80, 5.83] | 0.273 |
LAD (mm) | 47.2±7.3 | 45.2±5.8 | 0.134 |
LVDs (mm) | 57.9±10.0 | 58.0±9.3 | 0.952 |
LVDd (mm) | 68.0±9.7 | 68.9±9.0 | 0.624 |
RAD (mm) | 38.9±7.0 | 38.9±7.1 | 0.953 |
RVDd (mm) | 40.1±8.6 | 37.1±8.6 | 0.106 |
LVEF (%) | 32.3±7.3 | 33.1±7.7 | 0.615 |
Beta-blocker, n (%) | 24 (66.7) | 53 (89.8) | 0.005 |
ACEI/ARB, n (%) | 24 (66.7) | 50 (84.7) | 0.039 |
LAD-caIMR | 31.6±8.9 | 21.3±5.7 | <0.001 |
LCX-caIMR | 31.9±10.2 | 24.7±10.0 | 0.001 |
RCA-caIMR | 31.8±11.8 | 23.4±7.7 | <0.001 |
Data are expressed as n (%) or median [Q1, Q3] or mean ± standard deviation. SBP, systolic blood pressure; DBP, diastolic blood pressure; NT-proBNP, N-terminal prohormone of B-type natriuretic peptide; TC, total cholesterol; LDL-C, low-density lipoprotein cholesterol; FBG, fast blood glucose; LAD, left atrial diameter; LVDs, left ventricular diameter of systole; LVDd, left ventricular diameter of the diastole; RAD, right atrial diameter; RVDd, right ventricular diameter of the diastole; LVEF, left ventricular ejection fraction; ACEI, angiotensin-converting enzyme inhibitor; ARB, angiotensin receptor blockers; LAD-caIMR, coronary angiography-derived index of microcirculatory resistance for left anterior descending artery; LCX-caIMR, coronary angiography-derived index of microcirculatory resistance for left circumflex artery; RCA-caIMR, coronary angiography-derived index of microcirculatory resistance for right coronary artery.
The threshold of the caIMR in predicting the end points
Figure 2 shows the accuracy of the caIMR of the LAD, LCX, and RCA in predicting end points in patients with DCM. The best cutoff value of the caIMR of the LAD for end points was >29.8, with an area under the curve (AUC) of 0.828, a sensitivity of 63.9%, a specificity of 96.6%, a positive predictive value (PPV) of 92.0%, and a negative predictive value (NPV) of 81.4% (Table 2). The optimal predictive value of the caIMR of the LCX was >25.5 with an AUC of 0.720 (P<0.001). Additionally, the threshold of the caIMR of the RCA to determine end points was >29.7 with an AUC of 0.717 (P<0.001).
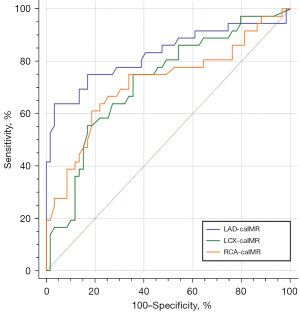
Table 2
Index | Positive threshold | AUC | Sen (%) | Spe (%) | PPV (%) | NPV (%) | P value |
---|---|---|---|---|---|---|---|
LAD-caIMR | >29.8 | 0.828 | 63.9 | 96.6 | 92.0 | 81.4 | <0.001 |
LCX-caIMR | >25.5 | 0.720 | 75.0 | 64.4 | 56.3 | 80.8 | <0.001 |
RCA-caIMR | >29.7 | 0.717 | 61.1 | 81.4 | 66.7 | 77.4 | <0.001 |
caIMR, coronary angiography-derived index of microcirculatory resistance; LAD-caIMR, coronary angiography-derived index of microcirculatory resistance for left anterior descending artery; LCX-caIMR, coronary angiography-derived index of microcirculatory resistance for left circumflex artery; RCA-caIMR, coronary angiography-derived index of microcirculatory resistance for right coronary artery; AUC, area under the curve; Sen, sensitivity; Spe, specificity; PPV, positive predictive value; NPV, negative predictive value.
Survival analysis based on the caIMR
The median follow-up period of all patients was 43.2 months, and 36 (37.9%) patients had their end point during this period. Specifically, there were 22 HF-related rehospitalizations, 6 device implantations, 1 heart transplantation, and 7 deaths. When patients were divided into the higher caIMR and lower caIMR groups according to the cutoff value of each coronary artery, Kaplan-Meier analysis showed that patients with a higher caIMR had an increased cumulative risk of an end point regardless of the cutoff values of the LAD, LCX, and RCA (Figure 3; all log-rank P values <0.001). Furthermore, we evaluated the differences in each component of the end points in the higher caIMR and lower caIMR groups stratified by the 3 coronary arteries (Figure S1). We found that patients with a higher caIMR had a greater frequency of HF-related rehospitalization (all P values <0.001). There were no differences in device implantation, heart transplantation, or death between the groups.
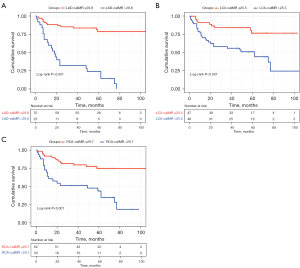
Effect of the caIMR on the end points
The use of beta-blockers, atrial fibrillation, LAD-caIMR, LCX-caIMR, and RCA-caIMR were significantly associated with the end points of patients with DCM in the unadjusted model. After adjustment for confounders, LAD-caIMR remained significant in predicting adverse outcomes in patients with DCM, indicating that LAD-caIMR was an independent predictor for primary end points. Each 1 unit raised in LAD-caIMR contributed to an 11% increased risk of primary end points (Table 3; HR =1.11, 95% CI: 1.06–1.16).
Table 3
Variables | Unadjusted analysis | Adjusted analysis | |||
---|---|---|---|---|---|
HR (95% CI) | P value | HR (95% CI) | P value | ||
Age | 1.00 (0.97–1.04) | 0.744 | 1.01 (0.98–1.05) | 0.478 | |
NT-proBNP | 1.00 (1.00–1.00) | 0.142 | 1.00 (1.00–1.00) | 0.004 | |
AF | 2.21 (1.08–4.54) | 0.031 | 2.17 (0.96–4.96) | 0.061 | |
LVDd | 0.99 (0.96–1.03) | 0.710 | 1.04 (1.00–1.09) | 0.064 | |
Beta-blockers | 0.39 (0.20–0.79) | 0.008 | 0.52 (0.24–1.13) | 0.098 | |
ACEI/ARB | 0.56 (0.28–1.12) | 0.102 | 0.63 (0.29–1.35) | 0.231 | |
LAD-caIMR | 1.11 (1.07–1.15) | <0.001 | 1.11 (1.06–1.16) | <0.001 | |
LCX-caIMR | 1.04 (1.01–1.06) | 0.003 | 0.99 (0.95–1.03) | 0.591 | |
RCA-caIMR | 1.05 (1.02–1.07) | 0.001 | 1.04 (1.00–1.07) | 0.053 |
caIMR, coronary angiography-derived index of microcirculatory resistance; DCM, dilated cardiomyopathy; NT-proBNP, N-terminal prohormone of B-type natriuretic peptide; AF, atrial fibrillation; LVDd, left ventricular diameter of the diastole; ACEI, angiotensin-converting enzyme inhibitor; ARB, angiotensin receptor blockers; LAD-caIMR, coronary angiography-derived index of microcirculatory resistance for left anterior descending artery; LCX-caIMR, coronary angiography-derived index of microcirculatory resistance for left circumflex artery; RCA-caIMR, coronary angiography-derived index of microcirculatory resistance for right coronary artery; HR, hazard ratio; CI, confidence interval.
Consistency of the caIMR among the LAD, LCX, and RCA regions
In order to explore the relationships between the caIMR values among the 3 coronary artery regions, we used a pie chart (Figure 4) and scatter plots (Figure 5) to compare the values of the caIMR in the three vessels. We divided the caIMR into high and low groups based on the cutoff values of each vessel from the ROC analyses. Figure 4 shows the distribution of the eight categories. The consistency with high-high-high and low-low-low accounted for 36.38% and 10.11% of total distribution of the caIMR among the three vessels, respectively. An almost 50% inconsistency of the caIMR was observed in 3 coronary artery domains. Furthermore, Figure 5 shows the correlations among the three vessels based on scatter plots. These findings showed a moderate correlation for each artery (R for LAD: 0.409; R for LCX: 0.461; R for RCA: 0.413; P<0.001).
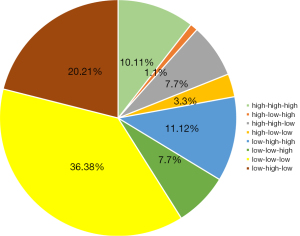
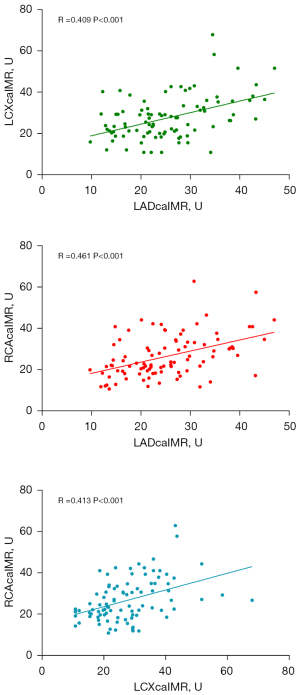
Discussion
This study is the first to assess the impact of coronary microvascular function on patients with DCM using the caIMR. The primary conclusion of our study was that caIMR could be a good predictor of adverse effects in patients with DCM. This new finding suggests that the measurement of the caIMR may provide an objective method of risk stratification for patients with DCM.
Histological examination of the hearts of patients with DCM shows unequal interstitial fibrosis, degenerated cardiomyocytes, and dilated heart chambers as the main pathological disorders of this disease (19). Myocardial ischemia was not previously assumed to play a role in the progression of the disease because it contains anatomically normal epicardial coronary arteries. However, rapidly evolving microcirculation assessment techniques, such as TTDE, CMR, and PET, have provided powerful support to conflict with traditional perceptions (20,21). Previous coronary endothelial dysfunction studies have shown that vascular abnormalities are closely related to myocardial fibrosis in patients with DCM (22). These studies indicated that DCM is also associated with vascular dysfunction for development and progression and represents a major predictor of adverse events even in the absence of CAD. However, current methods for assessing microcirculation in DCM are not suitable for large-scale clinical development. As a result, an ideal assessment method for assessing the severity of the disease and determining a worse prognosis may be useful for making treatment decisions.
In addition to the above-mentioned methods, existing literature has demonstrated the convenience of IMR as a standard for identifying microvascular damage (23). However, due to the increased procedural time, procedural costs, complexity of wire-derived IMR, and other factors affecting the results, IMR has not been extensively used in clinical diagnosis and therapy (24,25). With the development of computational fluid dynamics technology, caIMR, a type of imaging-derived functional indicator, has advanced the evaluation of CMD. It is well documented that caIMR has excellent diagnostic accuracy and consistency with CMR and traditional IMR for patients with ST-segment elevation myocardial infarction (STEMI) (13,14). Furthermore, caIMR is capable of predicting the risk of cardiac death and the readmission rate for patients with STEMI after they undergo percutaneous coronary intervention (PCI) (26,27). For myocardial infarction with non-obstructive coronary arteries (MINOCA), CMD has also been widely identified and can be detected in 30–56% of those patients treated in a catheterization laboratory (28,29). The prognostic value of the caIMR based on cutoff values also has clinical importance, with the best cutoff being >43 U (30). However, the predictive value of the caIMR in DCM is unclear. Our findings showed that the caIMR was significantly high in patients with DCM, and higher levels of LAD-caIMR (>29.8 U) were linked to lower survival rates, providing further support for the clinical importance of the caIMR in diagnosing and treating DCM.
Currently, the range of normal values for caIMR is inconclusive. As the caIMR was in good agreement with IMR, we may be able to derive the corresponding trends in the caIMR from the changes in IMR. Melikian et al. (31) analyzed 101 cases of epicardial coronary lesions and found that the normal value of IMR should be less than 25. The average IMR of patients with normal coronary angiograms found in a study of Europeans was 19±5, while that of a normal Chinese population reported by Luo et al. (32) was 18.8±5.6.
Previous studies have demonstrated that the IMR of diseased vessels in patients with STEMI may be generally greater than 40 (26,27). For patients with MINOCA, an IMR greater than 43 is a key risk stratification indicator (30). The elevated caIMR shows the presence of CMD and myocardial ischemic charge. To our knowledge, ours is the first study to assess the role of the caIMR in the DCM population. LAD-caIMR >29.8 was found to be an independent influencing factor of poor prognosis in our study. As for the reasons for the lower caIMR values in patients with dilated heart disease compared to those with myocardial infarction, we speculate that this may be due to poor myocardial perfusion caused by a greater number of plaque remnants and degree of thrombus dislodging into the distal microvasculature. In this study, we used offline caIMR assessments, so intracoronary nitroglycerin was not administered prior to angiography, which might be more biased compared to online caIMR. However, a study using offline caIMR to estimate the accuracy of the caIMR indicated that offline caIMR had excellent consistency with wire-based IMR, with an AUC of 0.919 (13). We surmised that the offline caIMR used in our study is capable of assessing CMD. In our study, there was a significantly higher level of low-density lipoprotein-cholesterol (LDL-C) in the event group than in the nonevent group. Atorvastatin has been reported to be effective in improving the performance of LVEF, inhibiting inflammation, and alleviating endothelium damage and endothelial dysfunction, which helps improve CMD in patients with DCM (33,34). Lovastatin was also shown to improve endothelial dysfunction and cellular cross-talk in DCM (35). We observed higher use of ACEI/ARB and beta-blockers in the no-events group, which might have been due to their positive effect on microcirculation as reported in previous studies (36,37).
ACEI/ARB beneficially influences cardiac function through mechanisms that improve CMD, including anti-inflammatory effects and anti-oxidative stress, strengthening both endothelial and microvascular function and rebalancing sympathetic dysregulation. Beta-blockers with vasodilatory effects improve the left ventricular filling pressure. Although ACEI and beta-blocker usage was lower in the events group and the protective effect of beta-blockers was confirmed with univariate regression, LAD-IMR remained strongly associated with adverse events after these variables were adjusted for, suggesting that high LAD-IMR remains an independent factor of poor prognosis in patients with DCM. Other reasons explaining the lower caIMR in patients with DCM were attributed to the lack of ischemia–reperfusion injury caused by PCI after vessel opening, which could have led to the destruction of the microcirculation compared in contrast to myocardial infarction.
We maintain a positive outlook on the treatment of CMD in patients with DCM. In addition to the drugs and devices commonly used in clinical practice, it has been reported that transplantation of CD34 (+) stem cells can promote the formation of new blood vessels in ischemic tissue by immediately incorporating cells brought into the creating vascular system or using producing and secreting angiogenic cytokines (38,39). Inhibitors of cyclic nucleotide phosphodiesterase PDE3 with vasodilatory actions may also be considered an adjunct therapy for DCM (40). Vascular endothelial growth factor B (VEGF-B) gene therapy also demonstrated its intracoronary cytoprotective function in a preclinical animal model of DCM (41). Furthermore, we noticed that the corrected thrombolysis in the myocardial infarction frame count (CTFC) correlated well with the caIMR and had a strong predictive power to identify CMD (42). We look forward to the development of more precise and convenient treatment tools for patients with CMD (42).
Several limitations of this study should be noted. First, the retrospective, single-center design possibly generated bias. Second, the main rationale for using CMR is to exclude confusing diagnoses, but this approach was rarely used in our study, which might have affected the accuracy of the diagnosis of DCM. It would be intriguing to explore the relationship between myocardial fibrosis and microcirculatory disorders. CMR is a promising tool for estimating myocardial fibrosis in DCM in clinical practice (43). However, we were not able to explore the relationship between CMD and fibrosis because the study contained a limited amount of cardiac MR data. A prospective cohort study is required to trace the time sequence of the myocardial fibrosis and CMD during a long-term follow-up in DCM. Doing so could better determine whether CMD is a side-effect of fibrosis or a potential pathophysiologic link in CMD-induced DCM. Finally, the caIMR was measured using blood pressure and coronary flow at rest, which might have biased the results from the invasive wire-based IMR using adenosine at hyperemia.
Conclusions
The caIMR was significantly associated with a poor prognosis in patients with DCM. Active assessment of the caIMR in patients with DCM may help in improved risk stratification and targeted treatment.
Acknowledgments
We would like to thank Rainmed Ltd., Suzhou, China, for supporting the computation of the caIMR.
Funding: This work was supported by the Science Foundation of Gusu School (No. GSKY20220102 to Xiangqing Kong), the Natural Science Foundation of Jiangsu Province (No. BK2012648 to Hui Wang), the Nanjing Science and Technology Innovation Projects for Overseas Graduates and the National Science Fund for Distinguished Young Scholars (No. 8220021578 to Zhi Zuo), and the General Scientific Research Project of Jiangsu Provincial Health Commission (No. M2020083 to Zhiyong Zhang).
Footnote
Reporting Checklist: The authors have completed the STARD reporting checklist. Available at https://qims.amegroups.com/article/view/10.21037/qims-22-1060/rc
Conflicts of Interest: All authors have completed the ICMJE uniform disclosure form (available at https://qims.amegroups.com/article/view/10.21037/qims-22-1060/coif). XK received funding from the Science Foundation of Gusu School (No. GSKY20220102); HW received funding from the Natural Science Foundation of Jiangsu Province (No. BK2012648). Zhi Zuo received funding from the Nanjing Science and Technology Innovation Projects for Overseas Graduates and the National Science Fund for Distinguished Young Scholars (No. 8220021578). Zhiyong Zhang received funding from the General Scientific Research Project of Jiangsu Provincial Health Commission (No. M2020083). The other authors have no conflicts of interest to declare.
Ethical Statement: The authors are accountable for all aspects of the work in ensuring that questions related to the accuracy or integrity of any part of the work are appropriately investigated and resolved. The study protocol was approved by the independent institutional ethics committee of the First Affiliated Hospital of Nanjing Medical University and complied with the Declaration of Helsinki (as revised in 2013). Written informed consent was acquired from all patients.
Open Access Statement: This is an Open Access article distributed in accordance with the Creative Commons Attribution-NonCommercial-NoDerivs 4.0 International License (CC BY-NC-ND 4.0), which permits the non-commercial replication and distribution of the article with the strict proviso that no changes or edits are made and the original work is properly cited (including links to both the formal publication through the relevant DOI and the license). See: https://creativecommons.org/licenses/by-nc-nd/4.0/.
References
- Pinto YM, Elliott PM, Arbustini E, Adler Y, Anastasakis A, Böhm M, et al. Proposal for a revised definition of dilated cardiomyopathy, hypokinetic non-dilated cardiomyopathy, and its implications for clinical practice: a position statement of the ESC working group on myocardial and pericardial diseases. Eur Heart J 2016;37:1850-8. [Crossref] [PubMed]
- Weintraub RG, Semsarian C, Macdonald P. Dilated cardiomyopathy. Lancet 2017;390:400-14. [Crossref] [PubMed]
- Rigo F, Gherardi S, Galderisi M, Pratali L, Cortigiani L, Sicari R, Picano E. The prognostic impact of coronary flow-reserve assessed by Doppler echocardiography in non-ischaemic dilated cardiomyopathy. Eur Heart J 2006;27:1319-23. [Crossref] [PubMed]
- Rigo F, Gherardi S, Galderisi M, Sicari R, Picano E. The independent prognostic value of contractile and coronary flow reserve determined by dipyridamole stress echocardiography in patients with idiopathic dilated cardiomyopathy. Am J Cardiol 2007;99:1154-8. [Crossref] [PubMed]
- Neglia D, Michelassi C, Trivieri MG, Sambuceti G, Giorgetti A, Pratali L, Gallopin M, Salvadori P, Sorace O, Carpeggiani C, Poddighe R, L'Abbate A, Parodi O. Prognostic role of myocardial blood flow impairment in idiopathic left ventricular dysfunction. Circulation 2002;105:186-93. [Crossref] [PubMed]
- Abdelmoneim SS, Dhoble A, Bernier M, Erwin PJ, Korosoglou G, Senior R, Moir S, Kowatsch I, Xian-Hong S, Muro T, Dawson D, Vogel R, Wei K, West CP, Montori VM, Pellikka PA, Abdel-Kader SS, Mulvagh SL. Quantitative myocardial contrast echocardiography during pharmacological stress for diagnosis of coronary artery disease: a systematic review and meta-analysis of diagnostic accuracy studies. Eur J Echocardiogr 2009;10:813-25. [PubMed]
- Gulati A, Ismail TF, Ali A, Hsu LY, Gonçalves C, Ismail NA, et al. Microvascular Dysfunction in Dilated Cardiomyopathy: A Quantitative Stress Perfusion Cardiovascular Magnetic Resonance Study. JACC Cardiovasc Imaging 2019;12:1699-708. [Crossref] [PubMed]
- Cortigiani L, Rigo F, Gherardi S, Galderisi M, Bovenzi F, Sicari R. Prognostic meaning of coronary microvascular disease in type 2 diabetes mellitus: a transthoracic Doppler echocardiographic study. J Am Soc Echocardiogr 2014;27:742-8. [Crossref] [PubMed]
- Cortigiani L, Rigo F, Sicari R, Gherardi S, Bovenzi F, Picano E. Prognostic correlates of combined coronary flow reserve assessment on left anterior descending and right coronary artery in patients with negative stress echocardiography by wall motion criteria. Heart 2009;95:1423-8. [Crossref] [PubMed]
- Ong P, Safdar B, Seitz A, Hubert A, Beltrame JF, Prescott E. Diagnosis of coronary microvascular dysfunction in the clinic. Cardiovasc Res 2020;116:841-55. [Crossref] [PubMed]
- Nishi T, Murai T, Ciccarelli G, Shah SV, Kobayashi Y, Derimay F, Waseda K, Moonen A, Hoshino M, Hirohata A, Yong ASC, Ng MKC, Amano T, Barbato E, Kakuta T, Fearon WF. Prognostic Value of Coronary Microvascular Function Measured Immediately After Percutaneous Coronary Intervention in Stable Coronary Artery Disease: An International Multicenter Study. Circ Cardiovasc Interv 2019;12:e007889. [Crossref] [PubMed]
- Qi Y, Gu R, Xu J, Kang L, Liu Y, Wang L, Chen J, Zhang J, Wang K. Index of microcirculatory resistance predicts long term cardiac systolic function in patients with STEMI undergoing primary PCI. BMC Cardiovasc Disord 2021;21:66. [Crossref] [PubMed]
- Ai H, Feng Y, Gong Y, Zheng B, Jin Q, Zhang HP, Sun F, Li J, Chen Y, Huo Y, Huo Y. Coronary Angiography-Derived Index of Microvascular Resistance. Front Physiol 2020;11:605356. [Crossref] [PubMed]
- Mejia-Renteria H, Lee JM, Choi KH, Lee SH, Wang L, Kakuta T, Koo BK, Escaned J. Coronary microcirculation assessment using functional angiography: Development of a wire-free method applicable to conventional coronary angiograms. Catheter Cardiovasc Interv 2021;98:1027-37. [Crossref] [PubMed]
- Shin D, Kim J, Choi KH, Dai N, Li Y, Lee SH, Joh HS, Kim HK, Kim SM, Ha SJ, Jang MJ, Park TK, Yang JH, Song YB, Hahn JY, Choi SH, Choe YH, Gwon HC, Lee JM. Functional angiography-derived index of microcirculatory resistance validated with microvascular obstruction in cardiac magnetic resonance after STEMI. Rev Esp Cardiol (Engl Ed) 2022;75:786-96. [Crossref] [PubMed]
- Heidenreich PA, Bozkurt B, Aguilar D, Allen LA, Byun JJ, Colvin MM, et al. 2022 AHA/ACC/HFSA Guideline for the Management of Heart Failure: A Report of the American College of Cardiology/American Heart Association Joint Committee on Clinical Practice Guidelines. Circulation 2022;145:e895-e1032. [Crossref] [PubMed]
- Chinese Society of Cardiology, Chinese Myocarditis Cardiomyopathy Collaborative Group. Guidelines for the diagnosis and treatment of dilated cardiomyopathy in China. J Clinical Cardiology 2018;34:421-34.
- Li J, Gong Y, Wang W, Yang Q, Liu B, Lu Y, Xu Y, Huo Y, Yi T, Liu J, Li Y, Xu S, Zhao L, Ali ZA, Huo Y. Accuracy of computational pressure-fluid dynamics applied to coronary angiography to derive fractional flow reserve: FLASH FFR. Cardiovasc Res 2020;116:1349-56. [Crossref] [PubMed]
- Ciarambino T, Menna G, Sansone G, et al. Cardiomyopathies: An Overview. Int J Mol Sci 2021;22:7722. [Crossref] [PubMed]
- Morton G, Chiribiri A, Ishida M, Hussain ST, Schuster A, Indermuehle A, Perera D, Knuuti J, Baker S, Hedström E, Schleyer P, O'Doherty M, Barrington S, Nagel E. Quantification of absolute myocardial perfusion in patients with coronary artery disease: comparison between cardiovascular magnetic resonance and positron emission tomography. J Am Coll Cardiol 2012;60:1546-55. [Crossref] [PubMed]
- Knaapen P, Götte MJ, Paulus WJ, Zwanenburg JJ, Dijkmans PA, Boellaard R, Marcus JT, Twisk JW, Visser CA, van Rossum AC, Lammertsma AA, Visser FC. Does myocardial fibrosis hinder contractile function and perfusion in idiopathic dilated cardiomyopathy? PET and MR imaging study. Radiology 2006;240:380-8. [Crossref] [PubMed]
- Nakayama M, Yamamuro M, Takashio S, Uemura T, Nakayama N, Hirakawa K, Oda S, Utsunomiya D, Kaikita K, Hokimoto S, Yamashita Y, Morita Y, Kimura K, Tamura K, Tsujita K. Late gadolinium enhancement on cardiac magnetic resonance imaging is associated with coronary endothelial dysfunction in patients with dilated cardiomyopathy. Heart Vessels 2018;33:393-402. [Crossref] [PubMed]
- Suda A, Takahashi J, Hao K, Kikuchi Y, Shindo T, Ikeda S, Sato K, Sugisawa J, Matsumoto Y, Miyata S, Sakata Y, Shimokawa H. Coronary Functional Abnormalities in Patients With Angina and Nonobstructive Coronary Artery Disease. J Am Coll Cardiol 2019;74:2350-60. [Crossref] [PubMed]
- De Maria GL, Scarsini R, Shanmuganathan M, Kotronias RA, Terentes-Printzios D, Borlotti A, Langrish JP, Lucking AJ, Choudhury RP, Kharbanda R, Ferreira VM, Channon KM, Garcia-Garcia HM, Banning AP. Angiography-derived index of microcirculatory resistance as a novel, pressure-wire-free tool to assess coronary microcirculation in ST elevation myocardial infarction. Int J Cardiovasc Imaging 2020;36:1395-406. [Crossref] [PubMed]
- Scarsini R, Shanmuganathan M, Kotronias RA, Terentes-Printzios D, Borlotti A, Langrish JP, Lucking AJ, Ribichini F, Ferreira VM, Channon KM, Garcia-Garcia HM, Banning AP, De Maria GL. Angiography-derived index of microcirculatory resistance (IMR(angio)) as a novel pressure-wire-free tool to assess coronary microvascular dysfunction in acute coronary syndromes and stable coronary artery disease. Int J Cardiovasc Imaging 2021;37:1801-13. [Crossref] [PubMed]
- Kotronias RA, Terentes-Printzios D, Shanmuganathan M, Marin F, Scarsini R, Bradley-Watson J, Langrish JP, Lucking AJ, Choudhury R, Kharbanda RK, Garcia-Garcia HM, Channon KM, Banning AP, De Maria GL. Long-Term Clinical Outcomes in Patients With an Acute ST-Segment-Elevation Myocardial Infarction Stratified by Angiography-Derived Index of Microcirculatory Resistance. Front Cardiovasc Med 2021;8:717114. [Crossref] [PubMed]
- Choi KH, Dai N, Li Y, Kim J, Shin D, Lee SH, et al. Functional Coronary Angiography-Derived Index of Microcirculatory Resistance in Patients With ST-Segment Elevation Myocardial Infarction. JACC Cardiovasc Interv 2021;14:1670-84. [Crossref] [PubMed]
- Schroder J, Michelsen MM, Mygind ND, Suhrs HE, Bove KB, Bechsgaard DF, Aziz A, Gustafsson I, Kastrup J, Prescott E. Coronary flow velocity reserve predicts adverse prognosis in women with angina and no obstructive coronary artery disease: results from the iPOWER study. Eur Heart J 2021;42:228-39. [Crossref] [PubMed]
- Sara JD, Widmer RJ, Matsuzawa Y, Lennon RJ, Lerman LO, Lerman A. Prevalence of Coronary Microvascular Dysfunction Among Patients With Chest Pain and Nonobstructive Coronary Artery Disease. JACC Cardiovasc Interv 2015;8:1445-53. [Crossref] [PubMed]
- Abdu FA, Liu L, Mohammed AQ, Yin G, Xu B, Zhang W, Xu S, Lv X, Fan R, Feng C, Shi T, Huo Y, Xu Y, Che W. Prognostic impact of coronary microvascular dysfunction in patients with myocardial infarction with non-obstructive coronary arteries. Eur J Intern Med 2021;92:79-85. [Crossref] [PubMed]
- Melikian N, Vercauteren S, Fearon WF, Cuisset T, MacCarthy PA, Davidavicius G, Aarnoudse W, Bartunek J, Vanderheyden M, Wyffels E, Wijns W, Heyndrickx GR, Pijls NH, de Bruyne B. Quantitative assessment of coronary microvascular function in patients with and without epicardial atherosclerosis. EuroIntervention 2010;5:939-45. [Crossref] [PubMed]
- Luo C, Long M, Hu X, Huang Z, Hu C, Gao X, Du Z. Thermodilution-derived coronary microvascular resistance and flow reserve in patients with cardiac syndrome X. Circ Cardiovasc Interv 2014;7:43-8. [Crossref] [PubMed]
- Liu M, Wang F, Wang Y, Jin R. Atorvastatin improves endothelial function and cardiac performance in patients with dilated cardiomyopathy: the role of inflammation. Cardiovasc Drugs Ther 2009;23:369-76. [Crossref] [PubMed]
- Fu L, Shang X, Zhang X. The Impact of Atorvastatin on Cardiac Performance for Dilated Cardiomyopathy: A Meta-analysis of Randomized Controlled Studies. Heart Surg Forum 2020;23:E329-34. [Crossref] [PubMed]
- Sayed N, Liu C, Ameen M, Himmati F, Zhang JZ, Khanamiri S, Moonen JR, Wnorowski A, Cheng L, Rhee JW, Gaddam S, Wang KC, Sallam K, Boyd JH, Woo YJ, Rabinovitch M, Wu JC. Clinical trial in a dish using iPSCs shows lovastatin improves endothelial dysfunction and cellular cross-talk in LMNA cardiomyopathy. Sci Transl Med 2020; [Crossref] [PubMed]
- Bairey Merz CN, Pepine CJ, Shimokawa H, Berry C. Treatment of coronary microvascular dysfunction. Cardiovasc Res 2020;116:856-70. [Crossref] [PubMed]
- Pearson JT, Thambyah HP, Waddingham MT, Inagaki T, Sukumaran V, Ngo JP, Ow CPC, Sonobe T, Chen YC, Edgley AJ, Fujii Y, Du CK, Zhan DY, Umetani K, Kelly DJ, Tsuchimochi H, Shirai M. β-blockade prevents coronary macro- and microvascular dysfunction induced by a high salt diet and insulin resistance in the Goto-Kakizaki rat. Clin Sci (Lond) 2021;135:327-46. [Crossref] [PubMed]
- Matta A, Nader V, Galinier M, Roncalli J. Transplantation of CD34+ cells for myocardial ischemia. World J Transplant 2021;11:138-46. [Crossref] [PubMed]
- Diaz-Navarro R, Urrútia G, Cleland JG, Poloni D, Villagran F, Acosta-Dighero R, Bangdiwala SI, Rada G, Madrid E. Stem cell therapy for dilated cardiomyopathy. Cochrane Database Syst Rev 2021;7:CD013433. [PubMed]
- Movsesian MA, Alharethi R. Inhibitors of cyclic nucleotide phosphodiesterase PDE3 as adjunct therapy for dilated cardiomyopathy. Expert Opin Investig Drugs 2002;11:1529-36. [Crossref] [PubMed]
- Woitek F, Zentilin L, Hoffman NE, Powers JC, Ottiger I, Parikh S, et al. Intracoronary Cytoprotective Gene Therapy: A Study of VEGF-B167 in a Pre-Clinical Animal Model of Dilated Cardiomyopathy. J Am Coll Cardiol 2015;66:139-53. [Crossref] [PubMed]
- Li M, Su H, Jiang M, Zuo Z, Su Z, Hao L, Yang J, Zhang Z, Wang H, Kong X. Predictive value of thrombolysis in myocardial infarction frame count for coronary microvascular dysfunction evaluated with an angiography-derived index of microcirculatory resistance in patients with coronary slow flow. Quant Imaging Med Surg 2022;12:4942-52. [Crossref] [PubMed]
- Borra D, Andalò A, Paci M, Fabbri C, Corsi C. A fully automated left atrium segmentation approach from late gadolinium enhanced magnetic resonance imaging based on a convolutional neural network. Quant Imaging Med Surg 2020;10:1894-907. [Crossref] [PubMed]