The diagnostic performance of AccuFFRangio for evaluating coronary artery stenosis under different computational conditions
Introduction
With the growing body of evidence attesting to the superiority of physiology assessment in guiding percutaneous coronary intervention (PCI) compared with angiographic guidance alone (1-4), fractional flow reserve (FFR) has become a guideline-recommended method for identifying lesions amenable to revascularization (5). However, the uptake of FFR in clinical applications remains low due to several limitations, including the complications attributed to pressure wire instrumentation, the pressure wire cost, the additional procedural time, and the operators’ confidence in anatomic assessment (6-8).
Alternative computational FFR approaches without a pressure wire have been established based on cardiac angiography and computational fluid dynamics (CFD). As one of these methods, AccuFFRangio has been recently reported as having a satisfactory agreement with wire-based FFR (9,10). AccuFFRangio computation takes roughly 5 minutes using 2 angiographic views for 3-dimensional (3D) vessel reconstruction, where the input aortic pressure (Pa) is from the patient-specific measurement at the coronary ostium, and the contrast flow velocity is derived from the thrombolysis in myocardial infarction (TIMI) frame count (11). However, the patient-specific aortic pressure measurement and 2 angiographic views are not always available in the clinical routine, which might affect the diagnostic accuracy of AccuFFRangio. A single-center study observed decreased diagnostic performance using a fixed Pa instead of patient-specific measurement (10). In contrast, Tu et al. (12) calculated the quantitative flow ratio (QFR) based on a single angiographic view and reported a similar diagnostic performance compared to that of QFR using 2 angiographic views. Therefore, it is necessary to systematically investigate the diagnostic performance of AccuFFRangio with the alteration of specific boundary conditions to facilitate the potential widespread use of functional assessment.
This study aimed to compare the diagnostic performance of AccuFFRangio and its variations using different boundary conditions or model reconstruction approaches; that is, input pressure with a fixed aortic pressure of Pa =100 mmHg (13,14) (pAccuFFRangio), input velocity with a fixed empiric hyperemic velocity of v = 0.35 m/s (15) (vAccuFFRangio), and a single angiographic view for vessel reconstruction (sAccuFFRangio). We present the following article in accordance with the STARD reporting checklist (available at https://qims.amegroups.com/article/view/10.21037/qims-22-893/rc).
Methods
Study design
This study was a retrospective, dual-center, observational study performed at The Second Affiliated Hospital, Zhejiang University School of Medicine and Zhejiang Hospital. The study aimed to compare the diagnostic accuracy, sensitivity, and specificity of AccuFFRangio under different conditions. The study was conducted in accordance with the Declaration of Helsinki (as revised in 2013). The study was approved by the ethics committee of The Second Affiliated Hospital, Zhejiang University School of Medicine and Zhejiang Hospital, and individual consent for this retrospective analysis was waived.
Patient population
Consecutive patients with stable angina pectoris who underwent invasive coronary angiography (ICA) and FFR assessment from June 2017 to September 2020 were enrolled in this study. The inclusion criteria were the following: (I) percentage diameter stenosis (DS%) between 30% and 90% by visual estimation and (II) angiographic projections ≥25° apart. The exclusion criteria were the following: (I) overlapping interrogated vessels with excessive foreshortening without preferred references in proximal and/or distal vessels, (II) insufficient injected contrast for quantitative coronary angiography (QCA) analysis, and (III) the location of the target lesion at the ostium of the left or right coronary artery. Patients were excluded if they had (I) acute myocardial infarction within 72 h, (II) severe asthma or chronic obstructive pulmonary disease, (III) an allergy to contrast media or adenosine, (IV) atrial fibrillation, (V) excessive pressure drift (absolute difference >3 mmHg), or (VI) a left main artery lesion.
Wire-based FFR measurement
FFR was measured using a coronary pressure wire (St. Jude Medical, St. Paul, MN, USA) in all patients. The pressure wire was advanced to the distal of the stenosis after calibration and equalization. Maximum hyperemia was induced by injecting adenosine triphosphate at a concentration of 150–180 µg/kg/min. The pressure sensor was placed at least 2 to 3 cm beyond the stenosis, where its exact location was documented. The proximal pressure at the coronary ostium and the distal coronary pressure at the pressure sensor were recorded simultaneously. Meanwhile, FFR was recorded as the ratio of the mean distal-to-proximal coronary pressure during maximum hyperemia. The sensor was then pulled back to the tip of the catheter to verify the pressure drift. The total time duration of the pressure recording varied between 1 and 4 min to cover the baseline, the hyperemic phase, and the recovery phase.
AccuFFRangio computation
AccuFFRangio V1.0 (ArteryFlow Technology, Hangzhou, China) was used for computation by participating physicians and technicians blinded to the wire-based FFR. AccuFFRangio used 2 angiographic views with projections ≥25° apart at the end-diastolic frame for the segmented vessel 3D reconstruction. The contrast flow velocity was derived with the TIMI frame count method. The input aortic pressure was measured at the coronary ostium. QCA data were also obtained from the software after the whole computation procedure.
To compare the diagnostic accuracy of AccuFFRangio using different conditions or reconstruction methods, we studied 3 variations of the standard AccuFFRangio. Each variation altered 1 condition in the standard AccuFFRangio computation process (Figure 1). In this study, we considered (I) a fixed value of aortic pressure of Pa =100 mmHg (pAccuFFRangio); (II) a contrast flow velocity (v) set to 0.35 m/s (vAccuFFRangio); and (III) 3D reconstruction using a single angiographic view (sAccuFFRangio), where the optimal view with minimal overlap of the interrogated vessel was selected by experienced radiologists. The assessments of AccuFFRangio and each variation were performed separately by different technicians to reduce bias. Details of the standard operating procedure for AccuFFRangio and its variations are provided in Figure S1 and elsewhere (9).
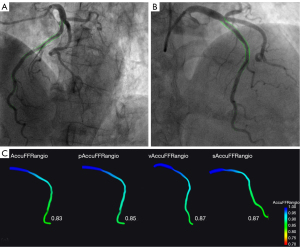
Statistical analysis
Continuous variables are expressed as the mean and standard deviation if normally distributed or median with interquartile range if nonnormally distributed. Categorical variables are expressed as percentages. The sample size calculation was based on the following assumptions: an accuracy of 85% for the variations of AccuFFRangio with the null hypothesis value set as 75%, type I error (α) =0.05 (2-sided), and statistical power (1 – β) =90%. At least 171 participants were required in the final analysis for AccuFFRangio and its variations. Assuming that 80% of the data had sufficient quality for analysis, at least 214 participants were required for enrollment. The data were analyzed on a per-vessel basis. Pearson correlation was used to measure the correlation between wire-based FFR and AccuFFRangio under different conditions. A Bland-Altman plot was used to measure the agreement between the wire-based FFR and AccuFFRangio under different conditions. We used wire-based FFR ≤0.8 as the reference standard, and this cutoff value was also applied to each type of AccuFFRangio diagnosis in this study. The performance of AccuFFRangio under different conditions for predicting functionally significant stenosis was evaluated according to diagnostic accuracy, sensitivity, specificity, positive predictive value (PPV), and negative predictive value (NPV). The area under the curve (AUC) of the receiver operating characteristic (ROC) analysis was used to assess the diagnostic accuracy of AccuFFRangio under different conditions. ROC curves were compared using the DeLong method. The statistical analysis was performed using MedCalc (MedCalc Software Inc., Ostend, Belgium).
Results
Patient characteristics
Figure 2 shows the enrollment flow chart of this study. Initially, 256 patients with 256 vessels were included, and 26 patients were excluded due to incomplete data, overlapping vessels, insufficient injected contrast, poor image quality, left main coronary artery lesion, 2 projections less than 25° apart, and excessive pressure wire drift. Therefore, 230 patients with 230 vessels were included in the final analysis. Table 1 summarizes the baseline clinical characteristics of the enrolled patients. The average age was 64.8±9.8 years, and 144 (62.6%) patients were male.
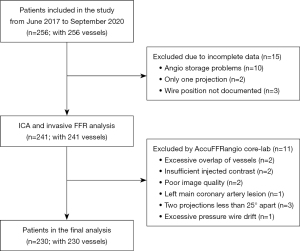
Table 1
Characteristic | Data |
---|---|
Age, year | 64.8±9.8 |
Male | 144 (62.6) |
Weight, kg | 68.2±33.5 |
Height, cm | 164.5±8.6 |
BMI, kg/m2 | 25.0±14.6 |
Systolic blood pressure, mmHg | 131.2±18 |
Diastolic blood pressure, mmHg | 77.3±13.8 |
Hypertension | 120 (52.2) |
Hypercholesterolemia | 25 (10.9) |
Kidney disease | 22 (9.6) |
Diabetes mellitus | 63 (27.4) |
Smoking history | 55 (23.9) |
Family history of coronary heart disease | 16 (7.0) |
Old myocardial infarction | 11 (4.8) |
Previous PCI | 49 (21.3) |
Previous CABG | 1 (0.4) |
Values are expressed as the mean ± standard deviation or as number (frequency); BMI, body mass index; PCI, percutaneous coronary intervention; CABG, coronary artery bypass graft.
Figure 3 shows the distribution of invasive FFR. The average FFR was 0.85±0.11. Two-dimensional QCA indicated that the average DS% was 44.5%±11.8%. FFR ≤0.80 was observed in 54 (23.5%) vessels. The vessel characteristics are listed in Table 2.
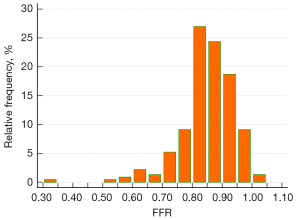
Table 2
Characteristic | Data |
---|---|
Vessels | |
LAD | 144 (62.6) |
LCX | 15 (6.5) |
RCA | 68 (29.6) |
First OM | 3 (1.3) |
Anatomy | |
Diameter stenosis, % | 44.5±11.8 |
Lesion length, mm | 22.43±8.10 |
Physiology | |
FFR (per vessel) | 0.85±0.11 |
Vessels with FFR ≤0.80 | 54 (23.5) |
Vessels with FFR >0.80 | 176 (76.5) |
Values are expressed as the mean ± standard deviation or as number (frequency). LAD, left anterior descending artery; LCX, left circumflex artery; RCA, right coronary artery; OM, obtuse marginal artery; FFR, fractional flow reserve.
Correlation and agreement between AccuFFRangio and FFR
Figure 4 shows the correlation coefficient between the standard AccuFFRangio calculation and the wire-based FFR was 0.82 (P<0.001). The correlation coefficient decreased to 0.75 when Pa =100 mmHg was used, to 0.65 when v =0.35 m/s was used, and to 0.63 when a single angiographic view was used. As shown in Figure 5, AccuFFRangio and its variations (pAccuFFRangio, vAccuFFRangio, and sAccuFFRangio) demonstrated agreement with FFR (mean difference: 0.004±0.112, –0.030±0.119, 0.035±0.192, and –0.027±0.165, respectively).
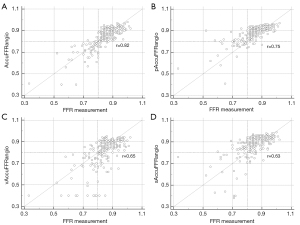
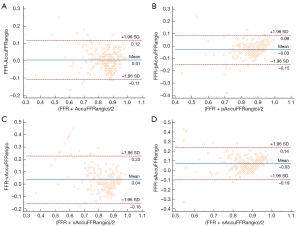
Diagnostic performance of AccuFFRangio
The accuracy, sensitivity, specificity, PPV, and NPV of the standard AccuFFRangio were 93.91%, 90.74%, 94.89%, 84.48%, and 97.09%, respectively. For pAccuFFRangio, these values were 86.52%, 51.85%, 97.16%, 84.85%, and 86.80%, respectively. For vAccuFFRangio, these values were 81.74%, 83.33%, 81.25%, 57.69%, and 94.08%, respectively. For sAccuFFRangio, these values were 83.48%, 46.30%, 94.89%, 73.53%, and 85.20%, respectively (Table 3). Among the 4 AccuFFRangio calculations, the standard AccuFFRangio had the highest accuracy, sensitivity, PPV, and NPV, but not specificity, which was 2.27% lower than that of pAccuFFRangio. The comparison of accuracy, sensitivity, and specificity between standard AccuFFRangio and its variations is presented in Table S1.
Table 3
Diagnostic characteristic | Standard AccuFFRangio ≤0.8 | pAccuFFRangio ≤0.8 | vAccuFFRangio ≤0.8 | sAccuFFRangio ≤0.8 | DS% ≤50% |
---|---|---|---|---|---|
Accuracy, % | 93.91 (90.00–96.63) | 86.52 (81.42–90.66) | 81.74 (76.13–86.51) | 83.48 (78.04–88.04) | 70.43 (64.08–76.25) |
Sensitivity, % | 90.74 (79.70–96.92) | 51.85 (37.84–65.66) | 83.33 (70.71–92.08) | 46.30 (32.62–60.39) | 42.59 (29.23–56.79) |
Specificity, % | 94.89 (90.51–97.64) | 97.16 (93.50–99.07) | 81.25 (74.69–86.73) | 94.89 (90.51–97.64) | 78.98 (72.21–84.75) |
Positive predictive value, % | 84.48 (74.13–91.19) | 84.85 (69.45–93.24) | 57.69 (49.51–65.48) | 73.53 (58.02–84.81) | 38.33 (28.96–48.66) |
Negative predictive value, % | 97.09 (93.54–98.72) | 86.80 (83.28–89.67) | 94.08 (89.71–96.66) | 85.20 (81.77–88.09) | 81.76 (77.88–85.10) |
Positive likelihood ratio | 17.74 (9.34–33.72) | 18.25 (7.41–44.96) | 4.44 (3.20–6.18) | 9.05 (4.50–18.20) | 2.03 (1.33–3.09) |
Negative likelihood ratio | 0.10 (0.04–0.23) | 0.50 (0.38–0.65) | 0.21 (0.11–0.37) | 0.57 (0.44–0.73) | 0.73 (0.57–0.93) |
Values inside the parentheses represent the 95% CI. DS, diameter stenosis.
The AUC with wire-measured FFR as the reference was 0.971 (95% CI: 0.940 to 0.989), 0.928 (95% CI: 0.887 to 0.958), 0.892 (95% CI: 0.844 to 0.929), and 0.870 (95% CI: 0.820 to 0.911) for the standard AccuFFRangio, pAccuFFRangio, vAccuFFRangio, and sAccuFFRangio, respectively (Figure 6). AccuFFRangio demonstrated superior diagnostic performance compared to the other variations (P<0.05 for all comparisons). Even so, the AUC for each AccuFFRangio variation was significantly higher than that of the QCA-derived DS% (AUC =0.716; 95% CI: 0.653 to 0.773) (P<0.001 for all comparisons).
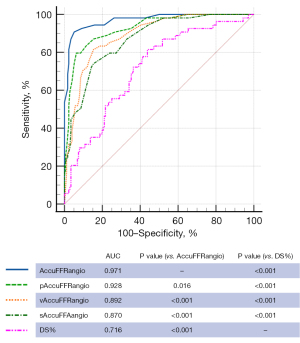
Discussion
In general, to acquire higher diagnostic accuracy, the segmented vessel, calculated average volume flow velocity during hyperemia, and aortic pressure are required to calculate AccuFFRangio distribution through the pressure drop equation. Three-dimensional reconstruction of the segmented vessel can be obtained using 2 images of ICA with projections ≥25° apart with minimal foreshortening and overlap of vessels. Flow velocity under baseline conditions can be calculated by performing the TIMI frame count in an angiographic run. Patient-specific aortic pressure is usually used as the input aortic pressure, and it can be obtained from the pressure measurement at the coronary ostium.
The standard AccuFFRangio uses 2 angiographic views for the 3D vessel reconstruction, then combined with a fast CFD method, it allows for a computation time of 5 minutes. In our study, patient-specific aortic pressure and TIMI frame count were used in the CFD computation. The diagnostic accuracy of the standard AccuFFRangio was 93.91%, which was comparable to previous studies, such as WIFI II, FAVOR II Europe-Japan, FAVOR II China, FLASH-FFR, FAST-FFR, and FAST II (16-21). The sensitivity, specificity, and AUC were 90.74%, 94.89%, and 0.971, respectively. However, the patient-specific aortic pressure measurement and 2 angiographic views are not always available in clinical settings.
In this study, we compared the standard AccuFFRangio with its 3 different variations. The standard AccuFFRangio had the highest accuracy of 93.91%. The accuracy decreased to 86.52% when Pa was set to a fixed value of 100 mmHg, to 81.74% when the inlet velocity was set to v =0.35 m/s, and to 83.48% when a single view for 3D reconstruction was used. The sensitivity, specificity, and AUC were 51.85%, 97.16%, and 0.928 in the Pa =100 mmHg condition; 83.33%, 81.25%, and 0.892 for the v =0.35 m/s condition; and 46.30%, 94.89%, and 0.870 for the single-view condition.
Compared to the standard AccuFFRangio, using a fixed value of aortic pressure of Pa =100 mmHg decreased the sensitivity from 90.74% to 51.85%. This significantly lower sensitivity can lead to the underestimation of the stenosis severity and untreated lesions. One of the reasons for the decreased sensitivity could be the significant difference in patients’ aortic pressure distribution. In this study, the mean Pa was 81 mmHg with a standard deviation of 12 mmHg. In incompressible flows, only the pressure gradient matters. However, a specific reference pressure still affects the AccuFFRangio value. For example, for a distal end pressure of 81 mmHg, the measured Pa of 102 mmHg results in AccuFFRangio =0.79, while the fixed Pa of 100 mmHg results in pAccuFFRangio =0.81. A 2-mmHg deviation from the measurement can change the classification result.
Compared to the standard AccuFFRangio, vAccuFFRangio decreased the accuracy (93.91% to 81.74%), sensitivity (90.74% to 83.33%), and specificity (94.89% to 81.25%). This decreased diagnostic performance is not surprising since CFD computation is sensitive to inlet boundary conditions. Thus, using the TIMI frame count method is recommended if time permits and angiography is available. Nevertheless, there is no need for additional user operation in vAccuFFRangio computation, enabling immediate assessment after 3D QCA analysis. The convenience and rapidity of this methodology might benefit the adoption of physiology guidance in the catheterization laboratory since it has shown better diagnostic performance than angiography guidance alone.
We now discuss the AccuFFRangio calculation using a single angiographic view, sAccuFFRangio. This method decreased the accuracy (93.91% to 83.48%) and sensitivity (90.74% to 46.30%). Similar to pAccuFFRangio, the sensitivity decreased significantly, leading to a significant number of untreated lesions. This finding might have resulted from information loss when only a single view was used for reconstruction, especially when the lesion complexity was severe. For example, a lesion with eccentricity and irregularity, viewed from a single angle, cannot reveal all the important characteristics of the lesion and their influences on blood flow (22). However, Tu et al. (12) showed that the derived FFR using a single angiographic view had a comparable diagnostic accuracy compared to that of FFR computation using 3D angiographic reconstruction from 2 angiographic views. This inconsistency needs to be further investigated, but the results in our study showed decreased overall performance when a single angiographic view was used to compare to a dual-view of angiography, especially the accuracy and sensitivity, which should be noted in daily clinical use.
Based on the diagnostic performances of standard AccuFFRangio, pAccuFFRangio, vAccuFFRangio, and sAccuFFRangio, it was evident that standard AccuFFRangio showed the best overall performance compared to the others. This finding indicates that using 2 angiographic views of ICA for 3D reconstruction, calculated velocity derived from the TIMI frame count, and patient-specific pressure is a more suitable and accurate combination when calculating AccuFFRangio distribution. Specifically, dual-view angiography with projections ≥25° apart with minimal foreshortening and overlapping vessels provided a more accurate and comprehensive view of the lesion area compared to a single view of angiography. Constant flow velocity cannot represent the average flow velocity during hyperemia for all the patients; therefore, using calculated average flow velocity is a more suitable choice for calculating pressure drop. As for the input aortic pressure, it is more precise to use patient-specific aortic pressure than a fixed value. The reasoning behind this is similar to that using calculated velocity versus constant velocity because it could cause underestimation or overestimation of the results. Thus, dual-view angiography with projections greater than 25° apart, calculated average volume flow velocity, and patient-specific aortic pressure are needed to obtain more accurate diagnostic performance of angiography-based FFR. Although it had suboptimal diagnostic performance, simplified AccuFFRangio distinguished itself from QCA-based assessment even with some missing information. Hence, these variations of AccuFFRangio still retain their clinical value, especially in relatively underdeveloped areas where equipment or medical resources may be limited and cannot meet the optimal requirements for AccuFFRangio computation.
This study had several limitations. First, the constant values selected for Pa and velocity in the pAccuFFRangio and vAccuFFRangio conditions, respectively, were based on literatures. The values might not be the most representative pressure and flow velocity, the optimal value might be different in real-world clinical practice. Second, the optimal view of angiography was selected manually in the sAccuFFRanigo condition, which might have introduced selection bias. Third, this study was an observational study with a limited sample size. Further studies are warranted to identify the diagnostic performance of different AccuFFRangio types in a broader range of patients.
Conclusions
This blinded, retrospective, and dual-center clinical study aimed to compare the diagnostic accuracy of AccuFFRangio with different configurations. The wire-based FFR ≤0.8 was used as the reference standard. The comparison suggested that the overall performance of the standard AccuFFRangio was superior to that of other configurations. However, the variations of AccuFFRangio demonstrated better diagnostic performance compared to angiographic assessment alone.
Acknowledgments
Funding: This work was supported by the National Natural Science Foundation of China (No. 82170332, and 82170329), the Zhejiang Provincial Public Welfare Technology Research Project (No. LGF20H020012), the Zhejiang Provincial Key Research and Development Plan (No. 2020C03016), and the Major Project of Social Development of Jinhua Science and Technology Project (No. 2021-3-028).
Footnote
Reporting Checklist: The authors have completed the STARD reporting checklist. Available at https://qims.amegroups.com/article/view/10.21037/qims-22-893/rc
Conflicts of Interest: All authors have completed the ICMJE uniform disclosure form (available at https://qims.amegroups.com/article/view/10.21037/qims-22-893/coif). XS received grants from the Major Project of Social Development of Jinhua Science and Technology Project (No. 2021-3-028). LD received grants from the National Natural Science Foundation of China (No. 82170329). CL received grants from the Zhejiang Provincial Public Welfare Technology Research Project (No. LGF20H020012). JJ received grants from the National Natural Science Foundation of China (No. 81100141, 81570322, and 82170332) and grants from the Zhejiang Provincial Key Research and Development Plan (No. 2020C03016). YH and XY are the employees of ArteryFlow. Xiaochang Leng is a cofounder of ArteryFlow. JX is the chief executive officer of ArteryFlow. The other authors have no conflicts of interest to declare.
Ethical Statement: The authors are accountable for all aspects of the work in ensuring that questions related to the accuracy or integrity of any part of the work are appropriately investigated and resolved. The study was conducted in accordance with the Declaration of Helsinki (as revised in 2013). The study was approved by the ethics committee of The Second Affiliated Hospital, Zhejiang University School of Medicine and Zhejiang Hospital, and individual consent for this retrospective analysis was waived.
Open Access Statement: This is an Open Access article distributed in accordance with the Creative Commons Attribution-NonCommercial-NoDerivs 4.0 International License (CC BY-NC-ND 4.0), which permits the non-commercial replication and distribution of the article with the strict proviso that no changes or edits are made and the original work is properly cited (including links to both the formal publication through the relevant DOI and the license). See: https://creativecommons.org/licenses/by-nc-nd/4.0/.
References
- Pijls NH, van Schaardenburgh P, Manoharan G, Boersma E, Bech JW, van't Veer M, Bär F, Hoorntje J, Koolen J, Wijns W, de Bruyne B. Percutaneous coronary intervention of functionally nonsignificant stenosis: 5-year follow-up of the DEFER Study. J Am Coll Cardiol 2007;49:2105-11. [Crossref] [PubMed]
- Tonino PA, De Bruyne B, Pijls NH, Siebert U, Ikeno F, van't Veer M, Klauss V, Manoharan G, Engstrøm T, Oldroyd KG, Ver Lee PN, MacCarthy PA, Fearon WF. Fractional flow reserve versus angiography for guiding percutaneous coronary intervention. N Engl J Med 2009;360:213-24. [Crossref] [PubMed]
- Van Belle E, Rioufol G, Pouillot C, Cuisset T, Bougrini K, Teiger E, et al. Outcome impact of coronary revascularization strategy reclassification with fractional flow reserve at time of diagnostic angiography: insights from a large French multicenter fractional flow reserve registry. Circulation 2014;129:173-85. [Crossref] [PubMed]
- Zimmermann FM, Ferrara A, Johnson NP, van Nunen LX, Escaned J, Albertsson P, Erbel R, Legrand V, Gwon HC, Remkes WS, Stella PR, van Schaardenburgh P, Bech GJ, De Bruyne B, Pijls NH. Deferral vs. performance of percutaneous coronary intervention of functionally non-significant coronary stenosis: 15-year follow-up of the DEFER trial. Eur Heart J 2015;36:3182-8. [Crossref] [PubMed]
- Knuuti J, Wijns W, Saraste A, Capodanno D, Barbato E, Funck-Brentano C, et al. 2019 ESC Guidelines for the diagnosis and management of chronic coronary syndromes. Eur Heart J 2020;41:407-77. [Crossref] [PubMed]
- Costopoulos C, Timmins LH, Huang Y, Hung OY, Molony DS, Brown AJ, Davis EL, Teng Z, Gillard JH, Samady H, Bennett MR. Impact of combined plaque structural stress and wall shear stress on coronary plaque progression, regression, and changes in composition. Eur Heart J 2019;40:1411-22. [Crossref] [PubMed]
- van Varik BJ, Rennenberg RJ, Reutelingsperger CP, Kroon AA, de Leeuw PW, Schurgers LJ. Mechanisms of arterial remodeling: lessons from genetic diseases. Front Genet 2012;3:290. [Crossref] [PubMed]
- Tebaldi M, Biscaglia S, Fineschi M, Musumeci G, Marchese A, Leone AM, Rossi ML, Stefanini G, Maione A, Menozzi A, Tarantino F, Lodolini V, Gallo F, Barbato E, Tarantini G, Campo G. Evolving Routine Standards in Invasive Hemodynamic Assessment of Coronary Stenosis: The Nationwide Italian SICI-GISE Cross-Sectional ERIS Study. JACC Cardiovasc Interv 2018;11:1482-91. [Crossref] [PubMed]
- Jiang J, Tang L, Du C, Leng X, He J, Hu Y, Dong L, Sun Y, Li C, Xiang J, Wang J. Diagnostic performance of AccuFFRangio in the functional assessment of coronary stenosis compared with pressure wire-derived fractional flow reserve. Quant Imaging Med Surg 2022;12:949-58. [Crossref] [PubMed]
- Li C, Leng X, He J, Xia Y, Jiang W, Pan Y, Dong L, Sun Y, Hu X, Wang J, Xiang J, Jiang J. Diagnostic Performance of Angiography-Based Fractional Flow Reserve for Functional Evaluation of Coronary Artery Stenosis. Front Cardiovasc Med 2021;8:714077. [Crossref] [PubMed]
- Dodge JT Jr, Rizzo M, Nykiel M, Altmann J, Hobkirk K, Brennan M, Gibson CM. Impact of injection rate on the Thrombolysis in Myocardial Infarction (TIMI) trial frame count. Am J Cardiol 1998;81:1268-70. [Crossref] [PubMed]
- Tu S, Ding D, Chang Y, Li C, Wijns W, Xu B. Diagnostic accuracy of quantitative flow ratio for assessment of coronary stenosis significance from a single angiographic view: A novel method based on bifurcation fractal law. Catheter Cardiovasc Interv 2021;97:1040-7. [Crossref] [PubMed]
- Papafaklis MI, Muramatsu T, Ishibashi Y, Lakkas LS, Nakatani S, Bourantas CV, et al. Fast virtual functional assessment of intermediate coronary lesions using routine angiographic data and blood flow simulation in humans: comparison with pressure wire - fractional flow reserve. EuroIntervention 2014;10:574-83. [Crossref] [PubMed]
- Tu S, Bourantas CV, Nørgaard BL, Kassab GS, Koo BK, Reiber JH. Image-based assessment of fractional flow reserve. EuroIntervention 2015;11 Suppl V:V50-4.
- Leisenring W, Alonzo T, Pepe MS. Comparisons of predictive values of binary medical diagnostic tests for paired designs. Biometrics 2000;56:345-51. [Crossref] [PubMed]
- Westra J, Tu S, Winther S, Nissen L, Vestergaard MB, Andersen BK, et al. Evaluation of Coronary Artery Stenosis by Quantitative Flow Ratio During Invasive Coronary Angiography: The WIFI II Study (Wire-Free Functional Imaging II). Circ Cardiovasc Imaging 2018;11:e007107. [Crossref] [PubMed]
- Li J, Gong Y, Wang W, Yang Q, Liu B, Lu Y, Xu Y, Huo Y, Yi T, Liu J, Li Y, Xu S, Zhao L, Ali ZA, Huo Y. Accuracy of computational pressure-fluid dynamics applied to coronary angiography to derive fractional flow reserve: FLASH FFR. Cardiovasc Res 2020;116:1349-56. [Crossref] [PubMed]
- Westra J, Andersen BK, Campo G, Matsuo H, Koltowski L, Eftekhari A, et al. Diagnostic Performance of In-Procedure Angiography-Derived Quantitative Flow Reserve Compared to Pressure-Derived Fractional Flow Reserve: The FAVOR II Europe-Japan Study. J Am Heart Assoc 2018; [Crossref] [PubMed]
- Xu B, Tu S, Qiao S, Qu X, Chen Y, Yang J, Guo L, Sun Z, Li Z, Tian F, Fang W, Chen J, Li W, Guan C, Holm NR, Wijns W, Hu S. Diagnostic Accuracy of Angiography-Based Quantitative Flow Ratio Measurements for Online Assessment of Coronary Stenosis. J Am Coll Cardiol 2017;70:3077-87. [Crossref] [PubMed]
- Masdjedi K, Tanaka N, Van Belle E, Porouchani S, Linke A, Woitek FJ, Bartorelli AL, Ali ZA, den Dekker WK, Wilschut J, Diletti R, Zijlstra F, Boersma E, Van Mieghem NM, Spitzer E, Daemen J. Vessel fractional flow reserve (vFFR) for the assessment of stenosis severity: the FAST II study. EuroIntervention 2022;17:1498-505. [Crossref] [PubMed]
- Kobayashi Y, Collet C, Achenbach S, Engstrøm T, Assali A, Shlofmitz RA, Fournier S, Kirtane AJ, Ali ZA, Kornowski R, Leon MB, De Bruyne B, Fearon WF. Diagnostic performance of angiography-based fractional flow reserve by patient and lesion characteristics. EuroIntervention 2021;17:e294-300. [Crossref] [PubMed]
- Takashima H, Waseda K, Gosho M, Kurita A, Ando H, Sakurai S, Maeda K, Kumagai S, Suzuki A, Amano T. Severity of morphological lesion complexity affects fractional flow reserve in intermediate coronary stenosis. J Cardiol 2015;66:239-45. [Crossref] [PubMed]