CT features and risk factors of pulmonary cement embolism after vertebroplasty or kyphoplasty in patients with vertebral compression fracture: a retrospective cohort study
Introduction
For patients with vertebral compression fractures, percutaneous vertebroplasty (PVP) or percutaneous kyphoplasty (PKP) are currently considered minimally invasive treatment options because they can significantly alleviate pain symptoms and prolong the survival time of patients as compared with nonsurgical treatment (1-6). In an attempt to correct vertebral deformity and control cement distribution, PKP uses orthopedic inflatable bone tamps before bone cement injection, while PVP is similar, using needles to deliver bone cement without orthopedic balloons. However, the incidence of complications after PVP has been reported to be between 1% and 10% (7-9). In a large retrospective study, 69.1% of patients had postoperative cement leakage, and 0.72% had clinical complications (10). Pulmonary cement embolism (PCE) caused by cement leakage is one of these complications. Wang et al. (11) reported the incidence of PCE during PVP to be 1.9% to 26%. Krueger et al. (12) reported the incidence of PCE after PVP and PKP ranging from 3.5% to 23% for osteoporotic fractures. Most existing studies on this subject consist of case reports using conventional chest radiograph. However, there are few reports on the computed tomography (CT)-derived imaging characteristics of PCE, the relative incidences of PVP- vs. PKP-induced PCE, and the specific risk factors involved, especially the risk of each vertebra for PCE. Zou et al. (13) reported that patients who underwent PVP had a significantly increased PCE risk than did those who underwent PKP. In contrast, a randomized control trial indicated that PKP had fewer cement leakages than did PVP and had a similar incidence of cement embolism (14). The difference in PCE caused by PVP and PKP remains unknown. Therefore, the purpose of our study was to summarize the characteristics of PCE on chest CT, compare the incidence d of PCE between PVP and PKP, and analyze the incidence and risk factors of PCE. We present the following article in accordance with the STROBE reporting checklist (available at https://qims.amegroups.com/article/view/10.21037/qims-22-569/rc).
Methods
Study cohort and design
This single-center, retrospective cohort study was conducted in accordance with the Declaration of Helsinki (as revised in 2013). The study was approved by the institutional ethics board of the China-Japan Friendship Hospital (No. 2022-KY-048), and individual consent for this retrospective analysis was waived. First, we retrospectively searched the electronic medical record system of China-Japan Friendship Hospital from January 2017 to December 2020. We screened 472 cases (157 males and 315 females; mean age, 78.0±9.4 years) with vertebral compression fracture or PVP or PKP. Based on the patients’ information, we only included the patients with preoperative vertebral X-ray and chest CT images and a postprocedural chest CT (including a dedicated chest CT with or without intravenous contrast, low-dose chest CT, or CT pulmonary angiography) through the Picture Archiving and Communication Systems (PACS; Carestream Health). A total of 99 patients were excluded because there was no postprocedural chest CT in our PACS, and then 373 patients were finally included in this research. Each patient’s demographic and clinical information, including operation type, operation level, the number of involved vertebrae, and the time interval from the operation to the first postoperative chest CT, were collected by 3 residents. The detailed flowchart of patient screening is shown in Figure 1.
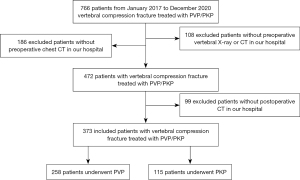
Image analysis
Two radiologists with 6 years of experience reviewed chest CT images together. They determined whether PCE could be diagnosed and the distribution of cement emboli. If there was a disagreement of opinion, the final diagnosis was made by a third chest radiologist with 15 years of experience.
PCE was diagnosed on the basis of a history of PVP or PKP and chest CT findings. PCE was defined as tubular, linear, or branching radiopaque densities with an attenuation >500 HU located along the course of the pulmonary artery on chest CT (15,16). Central PCE involved the main, right, and left as well as the lobar pulmonary arteries, while peripheral PCE only involved segmental and subsegmental pulmonary arteries.
Statistical analysis
The clinical and imaging data were analyzed using SPSS 26.0 (IBM Corp., New York, United States). Continuous variables are presented as mean ± SD. Comparisons between groups were performed using the t test or χ2 test. Univariate and binary logistic regression analysis was performed on possible risk factors for the occurrence of PCE. A 2-tailed P value <0.05 was considered statistically significant.
Results
The demographics and clinical information
Of the 373 patients (96 males, 277 females; mean age 76.2±9.4 years, age range 31–97 years), 258 patients underwent PVP, and the remaining 115 patients underwent PKP, involving a total of 472 vertebrae. After PVP or PKP, 274 patients underwent noncontrast chest CT, 19 patients underwent low-dose chest CT, 67 patients underwent high-resolution CT (HRCT), and 13 patients underwent CT pulmonary angiography (CTPA). PCE was detected using the postprocedural chest CT in 64 cases (16 males and 48 females; mean age 75.8±11.0 years) with an incidence of 17.2%. The time interval between PVP/PKP and the first chest CT after PVP/PKP was 2 to 1,482 days (median 412 days; 95% CI: 111–802 days). CTPA was performed in 13 cases within 2 days after PVP, in whom 4 and 2 patients were respectively diagnosed with PCE and acute pulmonary thromboembolism (APE). Figure 2 shows the distribution of surgical sites and the number of patients. Table 1 shows the demographics and clinical information of all enrolled patients.
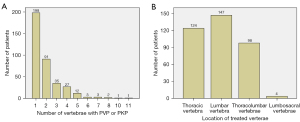
Table 1
Clinical information | Patients with pulmonary cement embolism | P value | ||
---|---|---|---|---|
Sum (N=373) | Positive (n=64) | Negative (n=309) | ||
Gender | 0.882 | |||
Male | 96 | 16 | 80 | |
Female | 277 | 48 | 229 | |
Age (years), mean ± SD | 76.2±9.4 | 75.8±11.0 | 76.3±9.1 | 0.708 |
Puncture method | 0.406 | |||
Percutaneous vertebroplasty | 258 | 47 | 211 | |
Percutaneous kyphoplasty | 115 | 17 | 98 | |
Location of treated vertebrae | 0.365 | |||
Thoracic | 124 | 24 | 100 | |
Lumbar | 147 | 19 | 128 | |
Thoracolumbar | 98 | 20 | 78 | |
Lumbosacral | 4 | 1 | 3 | |
Treated vertebrae | ||||
T1 vertebra | 0 | 0 | 0 | |
T2 vertebra | 1 | 0 | 1 | |
T3 vertebra | 1 | 0 | 1 | |
T4 vertebra | 2 | 1 | 1 | |
T5 vertebra | 9 | 2 | 7 | |
T6 vertebra | 11 | 5 | 6 | |
T7 vertebra | 22 | 8 | 14 | |
T8 vertebra | 28 | 7 | 21 | |
T9 vertebra | 29 | 13 | 16 | |
T10 vertebra | 27 | 10 | 17 | |
T11 vertebra | 76 | 21 | 55 | |
T12 vertebra | 137 | 28 | 108 | |
L1 vertebra | 148 | 23 | 125 | |
L2vertebra | 103 | 27 | 76 | |
L3 vertebra | 53 | 13 | 40 | |
L4 vertebra | 52 | 11 | 41 | |
L5 vertebra | 29 | 7 | 22 | |
Sacral vertebra | 3 | 1 | 2 | |
Number of treated vertebrae | 730 | 177 | 553 | 0.001 |
Cement leakage in the azygos vein | <0.001 | |||
Positive | 103 | 39 | 64 | |
Negative | 270 | 25 | 245 | |
Cement leakage in the inferior vena cava | <0.001 | |||
Positive | 8 | 6 | 2 | |
Negative | 365 | 58 | 307 | |
Cement leakage in the renal vein | ||||
Positive | 1 | 1 | 0 | |
Negative | 372 | 63 | 309 |
SD, standard deviation.
CT findings of PCE
Noncontrast chest CT indicates cement emboli as branching hyperdense or radiopaque lesions confined within the pulmonary artery courses (Figure 3). CTPA can also clearly show the linear hyperdense cement emboli in the pulmonary artery (Figure 4). There were 11 cases with PCE with the central type and 53 cases with the peripheral type. Cement embolisms were mainly involved in the anterior segment of the upper lobe of bilateral lungs; there were 39 cases in the anterior segment of the upper lobe of the right lung and 23 cases in the anterior segment of the upper lobe of the left lung. Cement embolisms were also present in the apical segment of the upper lobe of the right lung (20 cases), the upper lingual segment of the upper lobe of the left lung (18 cases), the posterior segment of the upper lobe of the right lung (16 cases), and the medial segment of the middle lobe of the right lung (17 cases). CT further showed that 103 cases had cement emboli in the azygos vein (Figure 5), and 8 cases had cement emboli in the inferior vena cava (Figure 6).
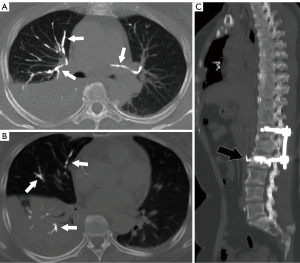
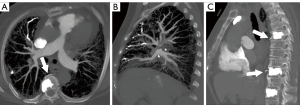
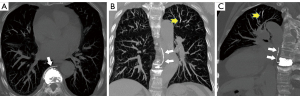
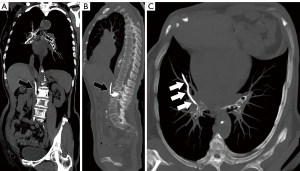
The diameter of the main pulmonary artery trunk in the PCE group was 27.6±4.8 cm, and there was no significant difference between the PCE and non-PCE groups (27.9±4.8 cm; t=0.454; P=0.650). The ratio of the main pulmonary artery diameter to aorta diameter in the PCE group (0.78±0.13) was comparable to that of the non-PCE group (0.79±0.13; t=0.905; P=0.366).
Risk factors of PCE
There was no significant gender difference between the PCE and non-PCE groups (χ2=0.022; P=0.882). The age in the PCE and non-PCE groups was comparable (t=0.375; P=0.708). The patients who underwent PVP and PKP between the PCE and non-PCE groups were similar (χ2=0.660; P=0.460). As shown in Table 1, there was no significant difference in the distribution of the treated vertebrae (thoracic vertebra, lumbar vertebra, thoracolumbar vertebrae, lumbosacral vertebrae) between the PCE and non-PCE groups (χ2=3.175; P=0.365). However, for each vertebra, PVP or PKP on T6 (χ2=6.385; P=0.026), T7 (χ2=6.067; P=0.021), T9 (χ2=16.937; P<0.001), T10 (χ2=8.092; P=0.008), T11 (χ2=7.366; P=0.007), and L2 vertebrae (χ2=8.209; P=0.004) in the PCE and non-PCE groups were significantly different. Between the PCE and non-PCE groups, there was also a significant difference in the number of treated vertebrae (χ2=23.71; P=0.001), azygos vein emboli (χ2=41.93, P<0.001), and inferior vena cava cement emboli (χ2=14.29; P<0.001). Binary logistic regression analysis (Table 2) showed that PVP or PKP on the T9 vertebra [odds ratio (OR) =4.222; 95% CI: 1.490–11.966], azygos vein emboli (OR =7.647; 95% CI: 3.937–14.856), and inferior vena cava cement emboli (OR =42.701; 95% CI: 7.525–242.302) were risk factors for PCE.
Table 2
Factors | P value | OR | 95% CI | |
---|---|---|---|---|
Lower limit | Upper limit | |||
T6 vertebra | 0.059 | 4.322 | 0.947 | 19.723 |
T7 vertebra | 0.287 | 1.996 | 0.599 | 7.119 |
T9 vertebra | 0.007 | 4.222 | 1.490 | 11.966 |
T10 vertebra | 0.250 | 1.990 | 0.616 | 6.429 |
T11 vertebra | 0.121 | 1.862 | 0.849 | 4.083 |
L2 vertebra | 0.088 | 1.998 | 0.902 | 4.428 |
Number of treated vertebrae | 0.917 | 0.985 | 0.746 | 1.301 |
Cement emboli in the azygos vein | <0.001 | 7.647 | 3.937 | 14.856 |
Cement emboli in inferior vena cava | <0.001 | 42.701 | 7.525 | 242.302 |
PVP, percutaneous vertebroplasty; PKP, percutaneous kyphoplasty; OR, odds ratio; CI, confidence interval.
Discussion
In our study, the incidence of PCE from PVP or PKP was 17.2%, and the incidence of PCE after PVP compared to that after PKP was similar. Postprocedural chest CT clearly indicated PCE as branching hyperdense or radiopaque lesions confined within the pulmonary artery courses. Cement emboli were mainly distributed in the upper lobes of both lungs. PVP or PKP in the T9 vertebra and cement emboli in the azygos vein or the inferior vena cava were risk factors of PCE after PVP and PKP.
Cement in the vertebra leaks into paravertebral venous plexus, from the azygos vein into the superior vena cava, or through the left renal vein and inferior vena cava, so as to enter the right atrium, right ventricle, or pulmonary artery, resulting in PCE (17-21). PCE has been extensively reported as a complication of PVP or PKP techniques, with the majority of the related literature being the form of case reports in which chest radiography was used for evaluation. Choe et al. (22) reported PCE is confirmed radiographically in 4.6% of patients after PVP or PKP. Another study retrospectively reviewed postprocedural chest radiographs, and 5 of 73 patients (6.8%) had PCE (8). Using follow-up CT, Venmans et al. (23) reported the incidence of PCE after PVP to be 26% (95% CI: 16–39%). In osteoporotic vertebral compression fractures, PCE was detected with CT in 23% of PVP sessions, developed in the distal to third-order pulmonary arteries, and was related to leakage into the inferior vena cava (16,24). In a systematic review, the incidence of PCE ranged widely, from 1.9% to 26% (11). In our study, the incidence of PCE from PVP and PKP was 17.2%. However, a chest radiograph or CT is not mandatory after PVP or PKP, so the true incidence of PCE may be underestimated (8,18). In cases of asymptomatic patients with peripheral PCE, Krueger et al. (12) recommended no treatment besides clinical follow-up. However, in cases of symptomatic emboli, they recommended proceeding according to the guidelines regarding the treatment of thrombotic pulmonary emboli, which included initial heparinization and a subsequent 6-month coumarin therapy. In our study, the median time interval between PVP or PKP and a chest CT scan after PVP or PKP was 412 days (95% CI: 111–802 days), indicating that asymptomatic PCE was a common condition during PVP and PKP and that asymptomatic patients did not receive any special treatment. Moreover, although PVP and PKP are two different percutaneous puncture procedures aimed at relieving pain rand reinforcing the bone of the vertebrae, our results found that the incidence of PCE from PVP and PKP was similar. This finding is consistent with the reports of Dohm et al. (14). However, Zou et al. (13) reported that patients who underwent PVP had a significantly increased risk of PCE compared to those who underwent PKP.
PCE on noncontrast CT is shown as linear, branching hyperdense, or radiopaque lesions in the pulmonary artery course. In contrast, the intensity of cement is higher than that derived with an iodine contrast agent. Thus, CTPA also can clearly display the high-density cement emboli in the pulmonary artery. However, cement emboli may be underestimated due to the influence of high-intensity contrast agents in the pulmonary artery (15,16). Density measurement can also be helpful to overcome this drawback, as contrast agents usually have lower attenuation than do cement emboli. Kim et al. (16), Venmans et al. (23), and Cianciulli et al. (25) all reported cement emboli that were small and scattered in peripheral portions of the lungs without specific lobar distribution and with no cement deposits in the heart and large vessels. In contrast, our study had 11 cases with central-type PCE and 53 cases with peripheral-type PCE. Cement emboli were mainly involved in the anterior segment of the upper lobe of both lungs. Patients underwent PVP or PKP in the prone position and remained bed in the prone position after surgery. Therefore, we speculate that the prone position may be the main reason for the specific distribution of PCE.
In a comparable study with the use of CT to detect PCE, Kim et al. (16) found cement leakage to the inferior cava vein as the only relevant risk factor. Hsieh et al. (10) reported a statistically significant correlation between the leakage of cement into the inferior vena cava and PCE, while Choe et al. (22) reported an association between PCE and paravertebral venous cement leakage. Venmans et al. (23) indicated that cement in the azygos vein on postprocedural CT of the treated vertebrae to be the only risk factor for PCE. In the study by Fadili Hassani et al. (19), a higher number of treated vertebrae during the PV procedure was an independent risk factor for the occurrence of intracardiac cement embolism. Zou et al. (13) indicated that patients with a thoracic fracture have a significantly increased risk of PCE than do those with a thoracolumbar fracture. In our study, we first analyzed the risk of each vertebra for PCE and found PVP or PKP in the T9 vertebra to be a risk factor for PCE. It is possible that the middle thoracic spine, including the T9 vertebra, is a more common vertebra of PCE because it is in close proximity to the inferior vena cava and the vena cava. However, the specific mechanism of PCE from PVP or PKP in the T9 vertebra remains unclear. A greater number of treated vertebrae was also found in the PCE group. Moreover, PVP or PKP in the T9 vertebra and cement emboli in the azygos vein or the inferior vena cava were found to be risk factors for PCE. Fadili Hassani et al. (19) reported the incidence of intracardiac cement embolism during PVP was low (3.9%), and concomitant pulmonary artery cement leakage was detected in 86.1% of the cases examined. However, no intracardiac cement embolism was found in our study, and only 1 case with cement leakage in the renal vein was found. Moreover, in cases of asymptomatic patients after PKP/PVP, we believe that chest CT is not mandatory to diagnose or exclude PCE. In cases of symptomatic patients after PKP or PVP, noncontrast CT would be the best method for evaluating PCE. For patients with suspected pulmonary thromboembolism after PKP or PVP, contrast-enhanced CT can clearly demonstrate PCE and differentiate PCE from a pulmonary thromboembolism.
Limitations
There are several limitations to our study. First, we employed a single-center, retrospective design, and only a relatively small number of patients who underwent chest CT after PVP or PKP were included; therefore, the incidences of PCE after PVP or PKP might have been underestimated. Although the incidences of PCE after PVP and PKP were similar, the effect of the volume of cement and the time from the operation to PCE were not analyzed. Additionally, the time interval of PVP or PKP to postoperative chest CT scan was 2 to 1,482 days (median, 412 days; 95% CI: 111–802), indicating that most cases of PCE were asymptomatic. Ultimately, the postoperative clinical symptoms or biomarkers that can suggest PCE remain unknown.
Conclusions
In this retrospective study, the incidence of PCE from PVP or PKP was 17.2% while the incidences of PCE after PVP and PKP were similar. PVP or PKP in the T9 vertebra and cement emboli in the azygos vein or the inferior vena cava were risk factors for PCE.
Acknowledgments
The abstract was accepted by 2022 Annual World Congress of the Pulmonary Vascular Research Institute as an electronic poster.
Funding: This work was supported by the National High Level Hospital Clinical Research Funding (No. 2022-NHLHCRF-LX-01), the Elite Medical Professionals Project of China-Japan Friendship Hospital (No. ZRJY2021-BJ02), the Medical And Health Science and Technology Innovation Project of the Chinese Academy of Medical Sciences (No. 2021-I2M-1-049), and the National Natural Science Foundation of China (No. 81871328).
Footnote
Reporting Checklist: The authors have completed the STROBE reporting checklist. Available at https://qims.amegroups.com/article/view/10.21037/qims-22-569/rc
Conflicts of Interest: All authors have completed the ICMJE uniform disclosure form (available at https://qims.amegroups.com/article/view/10.21037/qims-22-569/coif). All authors report that this work was supported by National High Level Hospital Clinical Research Funding (No. 2022-NHLHCRF-LX-01); Elite Medical Professionals Project of China-Japan Friendship Hospital (No. ZRJY2021-BJ02); Medical and health science and technology innovation project of Chinese Academy of Medical Science (No. 2021-I2M-1-049) and the National Natural Science Foundation of China (No. 81871328). The authors have no other conflicts of interest to declare.
Ethical Statement: The authors are accountable for all aspects of the work in ensuring that questions related to the accuracy or integrity of any part of the work are appropriately investigated and resolved. The study was conducted in accordance with the Declaration of Helsinki (as revised in 2013) and was approved by the institutional ethics board of the China-Japan Friendship Hospital (No. 2022-KY-048). Individual consent for this retrospective analysis was waived.
Open Access Statement: This is an Open Access article distributed in accordance with the Creative Commons Attribution-NonCommercial-NoDerivs 4.0 International License (CC BY-NC-ND 4.0), which permits the non-commercial replication and distribution of the article with the strict proviso that no changes or edits are made and the original work is properly cited (including links to both the formal publication through the relevant DOI and the license). See: https://creativecommons.org/licenses/by-nc-nd/4.0/.
References
- Chen AT, Cohen DB, Skolasky RL. Impact of nonoperative treatment, vertebroplasty, and kyphoplasty on survival and morbidity after vertebral compression fracture in the medicare population. J Bone Joint Surg Am 2013;95:1729-36. [Crossref] [PubMed]
- Filippiadis DK, Marcia S, Masala S, Deschamps F, Kelekis A. Percutaneous Vertebroplasty and Kyphoplasty: Current Status, New Developments and Old Controversies. Cardiovasc Intervent Radiol 2017;40:1815-23. [Crossref] [PubMed]
- Garfin SR, Buckley RA, Ledlie JBalloon Kyphoplasty Outcomes Group. Balloon kyphoplasty for symptomatic vertebral body compression fractures results in rapid, significant, and sustained improvements in back pain, function, and quality of life for elderly patients. Spine (Phila Pa 1976) 2006;31:2213-20. [Crossref] [PubMed]
- Klazen CA, Lohle PN, de Vries J, Jansen FH, Tielbeek AV, Blonk MC, et al. Vertebroplasty versus conservative treatment in acute osteoporotic vertebral compression fractures (Vertos II): an open-label randomised trial. Lancet 2010;376:1085-92. [Crossref] [PubMed]
- Ma XL, Xing D, Ma JX, Xu WG, Wang J, Chen Y. Balloon kyphoplasty versus percutaneous vertebroplasty in treating osteoporotic vertebral compression fracture: grading the evidence through a systematic review and meta-analysis. Eur Spine J 2012;21:1844-59. [Crossref] [PubMed]
- Tsoumakidou G, Too CW, Koch G, Caudrelier J, Cazzato RL, Garnon J, Gangi A. CIRSE Guidelines on Percutaneous Vertebral Augmentation. Cardiovasc Intervent Radiol 2017;40:331-42. [Crossref] [PubMed]
- Mansour A, Abdel-Razeq N, Abuali H, Makoseh M, Shaikh-Salem N, Abushalha K, Salah S. Cement pulmonary embolism as a complication of percutaneous vertebroplasty in cancer patients. Cancer Imaging 2018;18:5. [Crossref] [PubMed]
- Duran C, Sirvanci M, Aydoğan M, Ozturk E, Ozturk C, Akman C. Pulmonary cement embolism: a complication of percutaneous vertebroplasty. Acta Radiol 2007;48:854-9. [Crossref] [PubMed]
- Hatzantonis C, Czyz M, Pyzik R, Boszczyk BM. Intracardiac bone cement embolism as a complication of vertebroplasty: management strategy. Eur Spine J 2017;26:3199-205. [Crossref] [PubMed]
- Hsieh MK, Kao FC, Chiu PY, Chen LH, Yu CW, Niu CC, Lai PL, Tsai TT. Risk factors of neurological deficit and pulmonary cement embolism after percutaneous vertebroplasty. J Orthop Surg Res 2019;14:406. [Crossref] [PubMed]
- Wang LJ, Yang HL, Shi YX, Jiang WM, Chen L. Pulmonary cement embolism associated with percutaneous vertebroplasty or kyphoplasty: a systematic review. Orthop Surg 2012;4:182-9. [Crossref] [PubMed]
- Krueger A, Bliemel C, Zettl R, Ruchholtz S. Management of pulmonary cement embolism after percutaneous vertebroplasty and kyphoplasty: a systematic review of the literature. Eur Spine J 2009;18:1257-65. [Crossref] [PubMed]
- Zou D, Dong S, Du W, Sun B, Wu X. Risk factor analysis of pulmonary cement embolism during percutaneous vertebroplasty or kyphoplasty for osteoporotic vertebral compression fractures. J Orthop Surg Res 2021;16:312. [Crossref] [PubMed]
- Dohm M, Black CM, Dacre A, Tillman JB, Fueredi GKAVIAR investigators. A randomized trial comparing balloon kyphoplasty and vertebroplasty for vertebral compression fractures due to osteoporosis. AJNR Am J Neuroradiol 2014;35:2227-36. [Crossref] [PubMed]
- Huh S, Lee H. Pulmonary bone cement embolism: CT angiographic evaluation with material decomposition using gemstone spectral imaging. Korean J Radiol 2014;15:443-7. [Crossref] [PubMed]
- Kim YJ, Lee JW, Park KW, Yeom JS, Jeong HS, Park JM, Kang HS. Pulmonary cement embolism after percutaneous vertebroplasty in osteoporotic vertebral compression fractures: incidence, characteristics, and risk factors. Radiology 2009;251:250-9. [Crossref] [PubMed]
- Zohra A, Gonlugur U. Cement Pulmonary Embolism Due to Percutaneous Vertebroplasty. Curr Med Imaging 2021;17:559-61. [Crossref] [PubMed]
- Geraci G, Lo Iacono G, Lo Nigro C, Cannizzaro F, Cajozzo M, Modica G. Asymptomatic bone cement pulmonary embolism after vertebroplasty: case report and literature review. Case Rep Surg 2013;2013:591432. [Crossref] [PubMed]
- Fadili Hassani S, Cormier E, Shotar E, Drir M, Spano JP, Morardet L, Collet JP, Chiras J, Clarençon F. Intracardiac cement embolism during percutaneous vertebroplasty: incidence, risk factors and clinical management. Eur Radiol 2019;29:663-73. [Crossref] [PubMed]
- Kang HR, Kim TH, Chung CK, Lee CH. The impact of incidental pulmonary cement embolism on mortality risk. J Thromb Thrombolysis 2020;49:468-74. [Crossref] [PubMed]
- Rothermich MA, Buchowski JM, Bumpass DB, Patterson GA. Pulmonary cement embolization after vertebroplasty requiring pulmonary wedge resection. Clin Orthop Relat Res 2014;472:1652-7. [Crossref] [PubMed]
- Choe DH, Marom EM, Ahrar K, Truong MT, Madewell JE. Pulmonary embolism of polymethyl methacrylate during percutaneous vertebroplasty and kyphoplasty. AJR Am J Roentgenol 2004;183:1097-102. [Crossref] [PubMed]
- Venmans A, Klazen CA, Lohle PN, van Rooij WJ, Verhaar HJ, de Vries J, Mali WP. Percutaneous vertebroplasty and pulmonary cement embolism: results from VERTOS II. AJNR Am J Neuroradiol 2010;31:1451-3. [Crossref] [PubMed]
- Liu M, Tao XC, Zhai Z, Ma Z, Zhu L, Luo J. The filling defect of pulmonary artery, an imaging finding what we should know. Pulm Circ 2020;10:2045894020910687. [Crossref] [PubMed]
- Cianciulli TF, Mc Loughlin DE, Morita LA, Saccheri MC, Lax JA. Bone cement cardiac and pulmonary embolism. Echocardiography 2017;34:1239-41. [Crossref] [PubMed]