Cortical connectivity of cholinergic basal forebrain in Parkinson’s disease with mild cognitive impairment
Introduction
Cognitive impairment is the most common non-motor symptom of Parkinson’s disease (PD) and is associated with severely reduced quality of life (1,2). PD with mild cognitive impairment (PD-MCI), the early-stage cognitive deficit of PD, is a known risk factor for PD with dementia (PDD). According to previous studies, more than 80% of patients with PD and 90% of patients with PD-MCI gradually develop PDD in the late stages (3). Therefore, early identification of cognitive impairment in PD may provide a window of time to intervene and delay the progression to PDD. However, the mechanism of cognitive impairment in PD is unclear, and it may be affected by multiple factors, such as Lewy body deposition, Alzheimer-type pathology, and dysfunction of neurotransmitter systems. The dual syndrome hypothesis states that cholinergic deficit probably leads to dementia in PD (4). Therefore, it is very important to explore the role of the dysfunction of cholinergic basal forebrain (BF) in patients with PD-MCI.
The BF contains cholinergic cell populations in the brain, which are the major source of cholinergic fibers to the hippocampus, amygdala, and cortex (5). The BF consists of 4 different subdivisions, namely, the medial septal nucleus (Ch1), the nucleus of the vertical limb of the diagonal band (Ch2), the nucleus of the horizontal limb of the diagonal band (Ch3), and the nucleus basalis of Meynert (NBM/Ch4) (6). Among these subdivisions, Ch4 provides most of the projections to the amygdala and several cortical regions, whereas the hippocampus receives projections from Ch1-2 and the olfactory bulb from Ch3 (5). Previous studies have shown that Ch4 is impaired specifically in PD (7), and that Ch4 atrophy correlates with cognitive impairment progression in PD (8,9). Moreover, decreased functional connection has been found between Ch3-4 and the cortex in PD (10). However, in PD-MCI, the change of functional connection between BF and its subdivisions, especially the Ch4, is still unknown. Previous studies have utilized bilateral Ch4 as a region of interest (ROI) to investigate functional connectivity (FC) (10). However, impaired awareness in mild cognitive impairment (MCI) predicted [18F] fluorodeoxyglucose hypometabolism in the left BF but not in the right BF over a 24-month period (11), which may indicate the difference in the left and right BF in neurodegenerative diseases.
Moreover, previous studies have indicated that different frequency bands of neural activity in the brain can be used to reflect distinct physiological functions of brain activity (12,13). The low-frequency range has been subdivided into 4 subfrequency bands, among which gray matter (GM) signals are mainly concentrated on the slow 4 (0.027–0.073 Hz) and slow 5 (0.01–0.027 Hz) subbands. Recent studies have suggested frequency-specific FC changes in other neurological disorders and shown that FC in functional magnetic resonance imaging (fMRI) with specific frequency bands might provide sensitive information on pathophysiological mechanisms (14). Wang et al. found that patients with amnestic MCI showed decreased FC of their brain connectome in the frequency interval 0.031 to 0.063 Hz (15). The amplitude of low-frequency fluctuations in the inferior temporal gyrus and middle frontal gyrus in slow 4 was found to provide the highest classification accuracy in distinguishing patients with PD with freezing of gait from those with PD without freezing (16). However, little is known about FC of BF in patients with PD-MCI with different frequency bands. Therefore, we conducted resting-state functional magnetic resonance imaging (rs-fMRI) analyses in early-stage cognitive impairment of patients with PD, followed by seed-based analysis to explore the FC of BF and its subdivisions in each hemisphere. The following article is presented in accordance with the STROBE reporting checklist (available at https://qims.amegroups.com/article/view/10.21037/qims-22-582/rc).
Methods
Participants
In this retrospective study, to control for the potentially confounding effects of age and gender on FC, 66 patients with PD and 33 age- and sex-matched healthy controls (HC) were recruited from the outpatient and inpatient clinics of the Department of Guangdong Neuroscience Institute, Guangdong Provincial People’s Hospital, between October 2017 and January 2020. The participant recruitment process flowchart is displayed in Figure 1. The same sample of patients and controls were analyzed in our previous study from the perspective of fractional amplitude of low-frequency fluctuation and degree centrality (17). The educational level of participants enrolled in this study was higher than primary school to control for the potentially confounding effect of years of education on cognitive assessment. All patients with PD enrolled in this study were clinically diagnosed according to the Queen Square Brain Bank criteria (18). In addition, the onset age of PD was over age 50 for all patients. The exclusion criteria were as follows: Hoehn and Yahr (H-Y) stage >3, Hamilton Anxiety Scale (HAMA) >29 points, Hamilton Depression Scale (HAMD) >35 points, use of acetylcholinesterase inhibitors drugs, other diagnosed neurological diseases, and tumors and dementia according to the Movement Disorders Society criteria (19). The levodopa equivalent dose of each patient with PD was calculated according to the formula: LEED = levodopa dose + levodopa dose × 1/3 if on entacapone + piribedil (mg) + controlled release levodopa (mg) × 0.75 + pramipexole (mg) × 100 + selegiline (mg) × 10 + amantadine (mg). The Mini-Mental State Examination (MMSE) was used to assess the cognitive function of participants, and only those whose MMSE scores were higher than the cut-off value of the Chinese version of the instrument were recruited as HC (20). All participants signed informed consent prior to the study, which was in accordance with the ethical standards of the Declaration of Helsinki (as revised in 2013) and approved by the Ethics Committee of Guangdong Provincial People’s Hospital (No. GDREC2018338H [R1]).
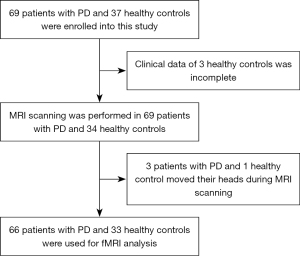
Clinical and neuropsychological evaluation
The Movement Disorder Society-sponsored Revision of the Unified Parkinson’s Disease Rating Scale part III (MDS-UPDRS-III) and H-Y scale were used to evaluate the severity of symptoms of patients with PD (21). The HAMA and HAMD were used for neurobehavioral assessment (22,23). Global cognitive function was evaluated using the MMSE and Montreal Cognitive Assessment (MoCA) (24). According to the Level II criteria of the MDS Task Force guidelines, participants with a score ≥1.5 standard deviation (SD) below the mean in at least 2 tests in 5 domains were identified as having PD-MCI (25). Executive functions were evaluated using the picture arrangement from the Wechsler Adult Intelligence Scale (WAIS-R) (26) and animal fluency test from the MoCA; memory was evaluated by immediate memory and logical memory tests from the Wechsler Memory Scale (WMS) (27); attention and working memory were evaluated using digit symbol and digit span tests from the WAIS-R; language was evaluated using the vocabulary and similarities subtests from the WAIS-R; visuospatial function was evaluated using block design and object assembly from the WAIS-R. Accordingly, 66 patients with PD were divided into 2 groups: 42 patients with PD with normal cognition (PD-NC) and 24 patients with PD with mild cognitive impairment (PD-MCI).
Neuroimaging
Magnetic resonance imaging (MRI) data acquisition and protocol
MRI data acquisition and postprocessing were conducted as described previously (17). Briefly, a 3.0 T scanner with an 8-channel head coil (Signa Excite HD; GE Healthcare, Milwaukee, WI, USA) was used to collect MRI data. To avoid pharmacological effects on neural activity, all patients with PD completed MRI scans in the morning during OFF state without taking antiparkinsonian medications for >12 h. High-resolution 3D T1-weighted images were acquired with repetition time (TR) =8.4 ms, echo time (TE) =3.3 ms, matrix =256×256, flip angle =13°, slice thickness =1 mm, and voxel size =0.94 mm × 0.94 mm ×1 mm).
All participants were asked to keep their eyes open and rest quietly during rs-fMRI scanning. rs-fMRI was performed with gradient-echo echo planer imaging (GRE-EPI) sequence [TR: 2,000 ms, TE: 30 ms, field of view (FOV): 240×240 mm, matrix: 64×64, slice thickness: 4 mm, voxel size: 3.75 mm × 3.75 mm × 4 mm, interslice space: 1 mm, NEX:1, and time points: 186, 5,580 images, and 30 axial slices covering the entire brain were acquired]. Axial scans were parallel to the anterior-posterior commissure (AC-PC) line.
MRI data preprocessing
The rs-fMRI data analyses were carried out with SPM12 software (http://www.fil.ion.ucl.ac.uk/spm) and RESTplus Version 1.2 (http://www.restfmri.net/forum/RESTplusV1.2) on the Matlab 2014a platform as previously described (17). To eliminate the scanner noise, the first 10 time points were removed. Next, slice timing, realignment, and spatial normalization were performed when preprocessing the data. All individual structural images were coregistered to the mean functional image and then were segmented into GM, white matter (WM), and cerebrospinal fluid (CSF). The realigned functional images of all participants were normalized with DARTEL into the standard Montreal Neurological Institute space. The normalized functional images were sampled to 3 mm × 3 mm × 3 mm. After removing the linear trend, head motion parameters, WM signal, and CSF signal were regressed out as covariates. Band-pass filtering was performed to exact fMRI signals in conventional (0.01–0.08 Hz) and subfrequency bands, including slow 5 (0.01–0.027 Hz), and slow 4 (0.027–0.073 Hz). Time courses were filtered using a (0.01–0.08 Hz) band to reduce high-frequency noise and low-frequency drifts.
There are 5 subfrequency bands in the full frequency range (0–0.25 Hz): slow 2: 0.198–0.25 Hz, slow 3: 0.073–0.198 Hz, slow 4: 0.027–0.073 Hz, slow 5: 0.01–0.027 Hz, and slow 6: 0–0.01 Hz. In this study, frequency-specific FC changes were performed. Therefore, in addition to the conventional frequency band (0.01–0.08 Hz), the subfrequency bands (including slow 4 and slow 5) were finally chosen to be further investigated, because slow 2, slow 3, and slow 6 subbands were affected by WM signals, low-frequency drifting, and high-frequency physiological noise (18,19).
Seed-based FC analysis
We selected the 4 ROIs including bilateral Ch1-3 and Ch4 to investigate FC. The BF consists of magnocellular cholinergic cell complex and is divided histologically into Ch1, Ch2, Ch3, and Ch4 (2). The masks of bilateral Ch1-3 and Ch4 were identified using probabilistic anatomical map obtained from the SPM Anatomy Toolbox (27,28). The probabilistic anatomical map is based on microscopic delineations of postmortem human brain and has been made available (28). The bilateral Ch1-3 and Ch4 were created using the SPM Anatomy Toolbox. We used DPABI Version 4.0 toolkit (http://rfmri.org/dpabi) to analyze seed-based FC. An automated anatomical labeling (AAL) atlas, dividing the brain into 116 cortical and subcortical ROIs, was employed to define the brain areas (29). The mean time series across all voxels within ROIs were obtained for each participant and each seed. The correlation coefficients, which were acquired between the mean time series and the time series of each other voxels in the whole brain, were converted to z scores using Fisher’s z transformation. The zFC maps were smoothed with a 4-mm FWHM isotropic Gaussian kernel. The value of each voxel throughout the whole brain represents the relative degree of FC with each seed.
The bilateral Ch1-3 and Ch4 volume was estimated using the same probabilistic anatomical map as previously described (30). Briefly, the brain was segmented into gray matter, WM, and CSF using the segmentation routine of the voxel-based morphometry (http://www.neuro.uni-jena.de/vbm/download/) and implemented in MATLAB R2018b (MathWorks, Natick, MA, USA). The segmented GM and WM were registered and normalized to Montreal Neurological Institute space using SPM’s DARTEL algorithm. The bilateral Ch1-3 and Ch4 volumes were calculated and normalized with respect to total intracranial volume.
Statistical analysis
The software SPSS =25.0 (IBM Corp., Armonk, NY, USA) was used to perform statistical analyses. Normality of each clinical variable was tested using the Kolmogorov–Smirnov test. Then, age, bilateral Ch1-3, and Ch4 volume were compared using one-way analysis of variance (ANOVA); education, HAMA, HAMD, MMSE, and MoCA were compared using the Kruskal–Wallis H test; the Mann–Whitney U test was used to compare differences in duration of disease, LEDD, MDS-UPDRS-III, picture arrangement, immediate memory, logical memory, digit symbol, vocabulary, similarities, block design, and object assembly test in PD-NC and PD-MCI; and the animal fluency test and digit span test were compared using the 2-sample t-test. Since the effects of head motion on FC were observed, mean framewise displacement (mFD) was calculated according to previous studies (31), and compared using the Kruskal–Wallis H test. The chi-square test was used to compare categorical variables.
To investigate differences in connectivity in these four ROIs among groups, 2-sample t-tests were performed using REST. If thresholds were given for each voxel, Monte Carlo simulations were used to define the random cluster size distribution. The resultant T-maps were thresholded using the Gaussian random field theory (GRF) correction (voxel P<0.005, cluster P<0.05) for multiple comparisons according to the distribution.
Correlations between FC and the clinical and neuropsychological evaluation were calculated. FC values (Z values) were extracted from regions that showed significant differences in connectivity to these four ROIs. Subsequently, to explore the relationship between the FC abnormalities and the functional impairments, Pearson correlation analysis was performed between FC within significant clusters and clinical variables in patients with PD-MCI and PD-NC, and multiple comparisons were adjusted for using the Bonferroni procedure with a factor of 28 to account for 2 groups of patients and 14 clinical variables (P<0.002). A corrected P<0.05 was considered statistically significant.
Results
Demographic and clinical characteristics
There were no differences in age (F=1.06, P=0.35), sex (Fisher exact test, P=0.33), and education (H=4.04, P=0.13) among the PD-NC, PD-MCI, and HC group. MMSE (H=13.91, P<0.001), HAMA (H=18.85, P<0.001), and HAMD (H=24.76, P<0.001) were significantly different between the groups. No difference was found in the duration of disease (Z=−0.23, P=0.82), MDS-UPDRS-III (Z=−0.75, P=0.46), H-Y stage (P=0.48), and levodopa equivalent daily dose (Z=−0.27, P=0.79) between the PD-NC and PD-MCI groups (Table 1).
Table 1
Variables | PD-NC | PD-MCI | HC | Z/F/H/χ2 | P value |
---|---|---|---|---|---|
Subjects | 42 | 24 | 33 | ||
Gender, females, n (%) | 16 (38.10%) | 7 (29.17%) | 16 (48.48%) | 2.22b | 0.33 |
Age (years) | 64.48 (6.87) | 65.75 (6.03) | 63.33 (5.31) | 1.06a | 0.35 |
Education | 10.83 (3.33) | 9.75 (3.47) | 11.39 (3.15) | 4.04c | 0.13 |
HAMD | 8.55 (9.05) | 10.08 (7.60) | 2.06 (2.08) | 24.76c | <0.001 |
HAMA | 7.33 (7.18) | 9.13 (6.93) | 2.85 (2.46) | 18.85c | <0.001 |
MMSE | 28.24 (1.89) | 26.14 (2.88) | 28.58 (1.50) | 13.91c | <0.001 |
MoCA | 23.26 (3.32) | 18.58 (4.44) | 26.21 (1.90) | 40.88c | <0.001 |
Duration of disease | 2.87 (2.19) | 2.81 (2.33) | – | −0.23d | 0.82 |
LEDD | 182.38 (336.56) | 147.08 (241.59) | – | −0.27d | 0.79 |
MDS-UPDRS-III | 31.17 (13.21) | 34.25 (16.82) | – | −0.75d | 0.46 |
H-Y stage | 2.00 (0.52) | 2.08 (0.64) | – | 3.52b | 0.48 |
Normalized volume of Ch1-3_L | 0.07 (0.01) | 0.06 (0.01) | 0.06 (0.01) | 0.36a | 0.70 |
Normalized volume of Ch1-3_R | 0.06 (0.01) | 0.06 (0.01) | 0.06 (0.01) | 1.23a | 0.30 |
Normalized volume of Ch4_L | 0.10 (0.01) | 0.09 (0.02) | 0.09 (0.02) | 0.55a | 0.58 |
Normalized volume of Ch4_R | 0.10 (0.01) | 0.09 (0.02) | 0.09 (0.02) | 1.82a | 0.17 |
mFD | 0.12 (0.09) | 0.14 (0.11) | 0.16 (0.11) | 3.39c | 0.18 |
The data are shown as the mean (standard deviation). a, one-way analysis of variance; b, Pearson’s chi-square (χ2), c, Kruskal-Wallis H test; d, Mann–Whitney U test. PD-NC, Parkinson’s disease with normal cognition; PD-MCI, Parkinson’s disease with mild cognitive impairment; HC, healthy control; HAMD, Hamilton Depression Scale; HAMA, Hamilton Anxiety Scale; MMSE, Mini-Mental State Examination; MoCA, Montreal Cognitive Assessment; LEDD, levodopa equivalent daily dose; MDS-UPDRS-III, Part III of the Movement Disorder Society-sponsored revision of the Unified Parkinson’s Disease Rating Scale; H-Y, Hoehn and Yahr Scale; Ch1-3_L, left Ch1-3; Ch1-3_R, right Ch1-3; Ch4_L, left Ch4; Ch4_R, right Ch4; mFD, mean framewise displacement.
The mFD (P=0.18), as well as volume of bilateral Ch1-3 (left, P=0.70; right, P=0.30) and Ch4 (left, P=0.58; right, P=0.17) were not significantly different among HC, PD-NC, and PD-MCI groups. There was no difference in the digit symbol test (Z=1.484, P=0.14) of the WAIS-R, which indicates no differences in attention and working memory between the PD-MCI and PD-NC, but the digit span test (t=−2.505, P=0.02) of the WAIS-R was worse in patients with PD-MCI compared with that of PD-NC. In addition, compared with PD-NC, patients with PD-MCI exhibited diminished executive function (picture arrangement, P<0.001; animal fluency test, P<0.001), memory (immediate memory, P<0.001; logical memory, P<0.001), language (vocabulary, P=0.03; similarities, P=0.002), and visuospatial function (block design, P=0.006; object assembly, P<0.001) (Table 2).
Table 2
Variables | PD-NC | PD-MCI | t/Z | P value |
---|---|---|---|---|
Picture arrangement | 8.79 (2.13) | 6.29 (2.33) | 3.885b | <0.001 |
Animal fluency test | 15.38 (2.87) | 11.13 (4.05) | −4.978a | <0.001 |
Immediate memory | 8.60 (2.93) | 2.04 (2.82) | 5.918b | <0.001 |
Logical memory | 6.29 (2.83) | 3.33 (1.17) | 4.909b | <0.001 |
Digit symbol | 7.19 (1.80) | 6.42 (2.10) | 1.484b | 0.14 |
Digit span | 10.55 (1.90) | 9.33 (1.88) | −2.505a | 0.02 |
Vocabulary | 12.26 (2.62) | 10.33 (3.47) | 2.76b | 0.03 |
Similarities | 6.29 (2.83) | 3.33 (1.17) | 3.048b | 0.002 |
Block design | 8.31 (2.11) | 6.58 (2.93) | 2.760b | 0.006 |
Object assembly | 6.57 (2.41) | 4.08 (2.02) | 3.957b | <0.001 |
The data are shown as the mean (standard deviation). a, two-sample t-tests; b, Mann–Whitney U test. PD-NC, Parkinson’s disease with normal cognition; PD-MCI, Parkinson’s disease with mild cognitive impairment.
FC of each ROI in BF
In the conventional frequency band, we only found a significant difference in FC in the right Ch4 among groups (GRF corrected, voxel P<0.005, cluster P<0.05) (Table 3, Figure 2). The PD-MCI group exhibited decreased FC values in the right middle cingulate and paracingulate gyri, right middle frontal gyrus, left inferior parietal gyrus, and left superior frontal gyrus, whereas an increased FC value was observed in the right calcarine fissure and surrounding cortex when compared with the HC group. The PD-MCI group exhibited increased FC value in the left calcarine fissure and surrounding cortex, wheras there was an decreased FC value in the right middle frontal gyrus when compared with the PD-NC group. However, there was no difference in the right Ch4-FC between patients with PD-NC and HC. Moreover, FC values between the PD-NC, PD-MCI, and HC groups were not significantly different in the left Ch4, left Ch1-3, and right Ch1-3.
Table 3
ROIs | Frequency bands | Contrast | Clusters No. | Number of voxel | Main brain regions | Peak MNI coordinate (x, y, z) | t value |
---|---|---|---|---|---|---|---|
Ch4_R | Conventional frequency band | PD-MCI vs. HC | 1 | 130 | Calcarine_R | 15, −96, −6 | 4.0614 |
2 | 132 | Cingulum_Mid_R | 3, −39, 36 | −4.415 | |||
3 | 172 | Frontal_Mid_R | 30, 30, 33 | −5.3615 | |||
4 | 59 | Parietal_Inf_L | −48, −51, 36 | −5.1066 | |||
5 | 63 | Frontal_Sup_L | −18, 36, 36 | −4.0553 | |||
6 | 99 | Frontal_Sup_L | −18, 9, 60 | −4.5736 | |||
PD-MCI vs. PD-NC | 1 | 144 | Calcarine_L | 0, −78, 6 | 4.4885 | ||
2 | 94 | Frontal_Mid_R | 30, 42, 36 | −4.5883 | |||
Slow 4 | PD-MCI vs. HC | 1 | 64 | Lingual_R | 9, −60, 0 | 4.935 | |
2 | 55 | Putamen_L | −21, −3, 12 | −3.827 | |||
3 | 208 | Frontal_Mid_R | 27, 42, 36 | −4.6999 | |||
4 | 58 | Precuneus_R | 3, −42, 45 | −3.867 | |||
5 | 124 | Frontal_Mid_L | −18, 24, 48 | −4.7368 | |||
PD-NC vs. HC | 1 | 77 | Temporal_Inf_R | 30, −24, −36 | −4.4447 | ||
PD-MCI vs. PD-NC | 1 | 137 | Frontal_Mid_R | 30, 42, 36 | −5.8167 | ||
2 | 57 | Cingulum_Mid_R | 3, −15, 39 | −4.4722 | |||
Slow 5 | PD-MCI vs. HC | 1 | 243 | Cerebellum_L | −9, −93, 21 | 4.9665 | |
PD-MCI vs. PD-NC | 1 | 195 | Calcarine_R | 9, −93, −3 | 4.3561 | ||
Ch1-3_L | Slow 4 | PD-MCI vs. PD-NC | 1 | 151 | Cingulum_Mid_R | 9, −42, 36 | −4.8412 |
All effects survived a voxel-wise statistical threshold (P<0.005) after GRF correction for multiple comparisons. *, main brain regions were located in white matter. PD-MCI, Parkinson’s disease with mild cognitive impairment; PD-NC, Parkinson’s disease with normal cognition; ROI, region of interest; MNI, Montreal Neurological Institute; HC, healthy control; R, right; L, left; Calcarine, calcarine fissure and surrounding cortex; Cingulum_Mid, middle cingulate and paracingulate gyri; Frontal_Mid, middle frontal gyrus; Parietal_Inf, inferior parietal, but supramarginal and angular gyri; Frontal_Sup, superior frontal gyrus; Lingual, lingual gyrus; Temporal_Inf, inferior temporal gyrus; GRF, Gaussian random field.
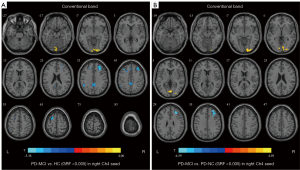
Regarding slow 4, for the right Ch4, compared with the HC group, the PD-MCI group showed significantly decreased FC values in the left putamen, right middle frontal gyrus, right precuneus, and left middle frontal gyrus, but an increased FC value in the right lingual gyrus, and the PD-NC group exhibited a decreased FC value in the right inferior temporal gyrus. The PD-MCI group exhibited lower FC values in the right middle frontal gyrus and the right middle cingulate and paracingulate gyri than the PD-NC group. Regarding the left Ch1-3, the FC values in the right middle cingulate and paracingulate gyri were lower in patients with PD-MCI compared with patients with PD-NC (Table 3, Figure 3).
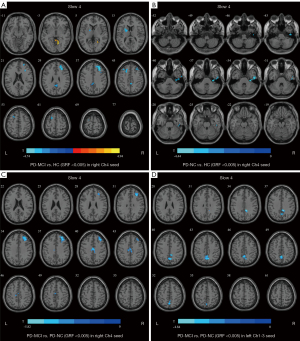
Regarding slow 5, for the right Ch4, the PD-MCI group showed a higher FC value in the right calcarine fissure and surrounding cortex compared with PD-NC in the left cerebellum when compared with HC (Table 3, Figure 4).
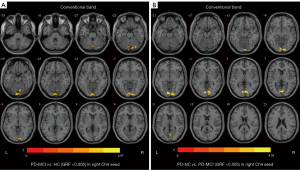
There was no difference in FC values among the three groups in the left Ch4 and right Ch1-3.
Correlation between FC and clinical variables
The correlations between clinical performance and FC values in significantly involved regions in PD-NC and PD-MCI group (Figure 5) were examined. Correlations between FC values and clinical performance were found in the right Ch4 rather than left Ch1-3 as per the seed-based analysis. HAMD scores of patients with PD-MCI were positively correlated with the FC values of the right middle cingulate and paracingulate gyri detected in the conventional frequency band (r=0.476, P=0.02). For global cognition, in patients with PD-MCI, MMSE scores were positively correlated with the FC values of the left calcarine fissure and surrounding cortex as detected in the conventional frequency band (r=0.435, P=0.03). Regarding executive function, the animal fluency test was positively correlated with the FC value in the right inferior temporal gyrus detected in patients with PD-NC in slow 4 (r=0.307, P=0.05). Regarding visuospatial functions, object assembly was negatively correlated with FC value in the right middle cingulate and paracingulate gyri in patients with PD-MCI in slow 4 (r=−0.406, P=0.05). Regarding memory function, immediate memory test scores were negatively correlated with FC values in the left putamen detected in patients with PD-MCI in the slow 4 (r=−0.431, P=0.04), and logical memory test scores were positively correlated with FC values in the right middle frontal gyrus detected in patients with PD-NC in the slow 4 (r=0.397, P=0.009). Regarding language function, the vocabulary test was negatively correlated with the FC value in the right calcarine fissure and surrounding cortex detected in patients with PD-MCI in the slow 4 (r=−0.432, P=0.04). However, these correlations did not persist once multiple comparisons were adjusted for. No significant correlations were observed in Ch1-3 seed-based FC in significantly altered regions with other cognitive tests scores.
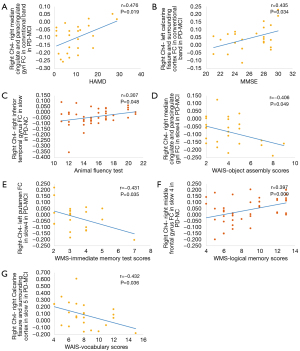
Discussion
Patients with PD with cognitive impairment presented evidence of abnormal FC in the BF. The findings of this study indicated there was reduced FC between Ch4 and frontal, parietal, occipital cortex in patients with PD-MCI compared with patients with PD-NC and HC. Furthermore, changes in connectivity within the Ch4-cortical network possibly correlated with depression (HAMD), global cognition (MMSE), and executive, visuospatial, memory, and language function. Longitudinal studies are needed to explore whether connectivity changes in the cholinergic BF nuclei are closely associated with cognitive decline in non-demented PD.
Similar to our findings in patients with non-demented PD, previous studies have shown volumetric and FC changes between Ch4 and several related cortical and subcortical regions in patients with PDD (10,32). This is in line with previous postmortem histological studies, and some neuroimaging findings (7,33). Severely reduced cortical cholinergic activity and loss of Ch4 cholinergic neurons in PD and PDD have been reported by many studies (7,34). Overall, these changes indicate widespread α‐synuclein‐immunopositive Lewy neurites and Lewy bodies deposition in Ch4 in patients with PDD (7). Some positron emission tomography (PET) studies identified that acetylcholinesterase activity was significantly decreased in the cerebral cortex in patients with PDD and cortical hypometabolism in patients with PD with Ch4 atrophy (35,36). Interestingly, cortical cholinergic deficit was more severe in patients with PDD than patients with Alzheimer’s disease (AD) (7). Furthermore, atrophy of Ch4 observed in patients with PD without cognitive impairment precedes and predicts future dementia, and increased microstructural deficit of NBM in patients with PD predicts cognitive impairment (9,37). Consistent with these changes, reduced FC between Ch4 and anatomical cortical areas was detected in patients with PD-MCI in this study. The volume of NBM was not significantly different between PD-MCI and PD-NC, and, thus, the possibility that reduced FC in Ch4-corticocal network caused by NBM atrophy was not considered in this study. Similar results have not yet been reported in patients with PD-MCI. Therefore, the potential for neuromodulatory treatments targeting the Ch4, such as repetitive transcranial magnetic stimulation (rTMS) and deep brain stimulation (DBS), is under active investigation. Previous studies have shown impairment of structural and FC in different cholinergic BF nuclei in patients with PD (10). In contrast, we hardly found any significant regions in left Ch1-3 seed-based FC except for reduced FC in the right middle cingulate and paracingulate gyri in patients with PD-MCI compared to PD-NC. A postmortem study found cholinergic septal neurons were largely spared, but a nearly 50% cholinergic neurons loss in Ch4 was observed in patients with PD (7). Similarly, lower volume of Ch4 is followed by changes in Ch1/Ch2, reflecting a posterior-anterior pattern of BF atrophy in a longitudinal structural MRI study (37). All of these results may indicate that MCI in the participants with PD appeared firstly as abnormal FC between Ch4 and cortex, but abnormal FC in Ch1-3 may have arisen when cognitive impairment was aggravated. In addition, no significant FC was observed between Ch1‐3 and the other regions of cortex except for the right middle cingulate and paracingulate gyri adjacent to the hippocampus, which may be explained by indirect FC.
Consistent with previous results, FC values were decreased in the occipital, parietal, and temporal areas and the limbic system, and possibly correlated with global cognition (MMSE), and executive and memory function based on right Ch4-seed analysis. In de novo patients with PD, degeneration of BF and concomitant cholinergic cortical denervation are associated with impaired memory, attention, and executive dysfunction (8,38,39). Executive tasks require planning and working memory, which requires a frontoparietal network that includes the prefrontal associative cortex (DLPFC) and posterior parietal cortex (40,41). These regions receive afferents from Ch4, possibly reflecting functional deficits of BF cholinergic circuits in patients with PD-MCI. The cognitive deficits that related closely with functional alterations of the BF cholinergic system were consistent with those brought out by acetylcholinesterase inhibitors medication (42). However, in this study, patients with PD taking anticholinergic medication were excluded, suggesting our findings were due to the degeneration of NBM. However, as the correlations did not persist following Bonferroni correction, the finding should be interpreted with caution. One explanation for this could be the small sample size, and longitudinal studies with larger sample sizes are needed to verify these results.
Unexpected results in this study were that the right cingulate areas were positively correlated with HAMD scores in patients with PD-MCI in the right Ch4 seed-based analysis. Previous structural and functional studies confirmed that patients with damaged cingulate cortex and reduced cortical cholinergic activity are susceptible to depression with PD and primary depression (43-45). Given these findings, there may be an analogous pathophysiological mechanism between depression with PD and primary depression, as the cingulate cortex is largely involved in the pathophysiology of primary depression as well as depression with PD. In addition, there were possibly negative associations between the middle cingulate cortex and visuospatial function, the left putamen and memory function, as well as the occipital cortex and language function, which is inconsistent with previous studies (10,38). Although the interpretation of negative correlations in fMRI in these regions is challenging, a possible interpretation of negative correlations is a loss of inhibition. Taylor et al. (46) suggested a loss of inhibition in the visual system in dementia with Lewy bodies, indicating possibly a negative association between cortical excitability in the visual system and cognitive function. Moreover, opposite findings were reported between the left and right calcarine fissure and the surrounding cortex in patients with PD-MCI. Increased FC in the right calcarine fissure and surrounding cortex were possibly correlated with reduced cognitive performance in patients with PD-MCI in slow 5, but reduced FC in the left calcarine fissure and surrounding cortex was possibly correlated with reduced cognitive performance in conventional band. An explanation for these discrepant findings may be that the frequency is specific with slow 5 being more sensitive for detecting abnormality in the dorsolateral prefrontal cortex, and also a stronger connectivity in the fronto-occipital pathways has been found in neurodegenerative disease (47), and thus increased FC in the occipital cortex could be observed in patients with PD-MCI in slow 5.
Consistent with our finding of reduced FC between the right Ch4, rather than the left, and the cortex in patients with PD-MCI, Teipel et al. (48) also found right NBM dominance both in the extent of atrophy and in the effects on fiber tracts in patients with MCI and AD, which may indicate different stages of NBM degeneration in both hemispheres. The prealpha neurons in entorhinal regions receive cholinergic projection from the BF (49). Numbers of prealpha neurons were significantly lower in the right entorhinal region compared with the left entorhinal region in postmortem brains from individuals ranging from 18 to 86 years of age (50), which may suggest that cholinergic neurons in the right rather than the left entorhinal region were more prone to degenerate (48). However, data on both hemispheres of neuron numbers in BF cholinergic nuclei in patients with PD-MCI are still lacking. More studies are needed to address this question in the future.
We explored FC values associated with BF in the different frequency bands, mainly in conventional, slow 4, and slow 5. This is an effective method to seek out abnormal functional changes in patients with PD-MCI. We found that right Ch4 presented reduced FC values with associative frontal, occipital, and parietal cortex in patients with PD-MCI in the conventional band, which is consistent with those in slow 4 to some extent, but different from those in slow 5. We also found reduced abnormal FC in the left putamen in slow 4 but not in the conventional band nor in slow 5. Previous studies have highlighted that neural activity in the brain is found in various frequency bands (51,52). Thus, merely discovering neural activity in the conventional band possibly omits significant findings from spontaneous blood-oxygen-level-dependent (BOLD) fluctuations at specific frequencies.
Previous studies suggested slow 4 exhibited less power and was more likely to be localized within the subcortical structure (including basal ganglia) (52,53). Our results indicated that slow 4 might be sensitive to abnormal putamen activity, which is consistent with related findings in patients with depressed PD (54). The FC in patients with PD-MCI was significantly decreased in the frontal and parietal cortex in slow 4, which was almost consistent with those found in the conventional band. These analogous patterns might indicate slow 4 was more sensitive for detecting abnormal FC changes in patients with PD-MCI. Moreover, slow 5 might be more sensitive for detecting abnormality in the dorsolateral prefrontal cortex and limbic system (12,55). Similarly, patients with PD-MCI in our study mainly exhibited FC changes in the calcarine fissure and surrounding cortex.
There were several limitations to the current study, which should be considered. First, this is a cross-sectional study with a relatively small sample size. To overcome this issue, the patients with PD enrolled in this study were clinically confirmed and matched with HC for age, sex, and education. In addition, the voxel threshold was set as P<0.005. In the future, more multicenter prospective and multimodal studies with stricter statistical thresholds are needed to verify these results. Second, there were some patients enrolled in our study who had been treated by medications but their MRI scans were performed without antiparkinsonian drugs. To overcome this issue, we matched the levodopa equivalent daily dose of each PD group. Furthermore, rs-fMRI were used to detect changes of FC, but the histological changes of the cholinergic system are poorly understood, and we cannot determine these changes due to the degeneration of cholinergic neurons in the BF.
Conclusions
Our study showed that connectivity changes in the cholinergic BF nuclei were possibly associated with the cognitive impairments in nondemented PD from the perspective of resting-state FC in different frequency bands, and the study identified an imbalanced association between different cholinergic BF nuclei and cortical regions, which may provide new insights into functional changes within cholinergic BF nuclei in cognitive impairment associated with PD.
Acknowledgments
We would like to thank all the patients for their cooperation in this study.
Funding: This work was supported by the National Natural Science Foundation of China (No. 82071419); the High-Level Hospital Construction Project (No. DFJH201907); Supporting Research Funds for Outstanding Young Medical Talents in Guangdong Province (No. KJ012019442); the Medical Scientific Research Foundation of Guangdong Province, China (Nos. A2019141 and A2018036).
Footnote
Reporting Checklist: The authors have completed the STROBE reporting checklist. Available at https://qims.amegroups.com/article/view/10.21037/qims-22-582/rc
Conflicts of Interest: All authors have completed the ICMJE uniform disclosure form (available at https://qims.amegroups.com/article/view/10.21037/qims-22-582/coif). The authors have no conflicts of interest to declare.
Ethical Statement: The authors are accountable for all aspects of the work in ensuring that questions related to the accuracy or integrity of any part of the work are appropriately investigated and resolved. All participants in this study signed informed consent prior to the study, which was in accordance with the ethical standards of the Declaration of Helsinki (as revised in 2013) and approved by the Ethics Committee of Guangdong Provincial People’s Hospital (No. GDREC2018338H [R1]).
Open Access Statement: This is an Open Access article distributed in accordance with the Creative Commons Attribution-NonCommercial-NoDerivs 4.0 International License (CC BY-NC-ND 4.0), which permits the non-commercial replication and distribution of the article with the strict proviso that no changes or edits are made and the original work is properly cited (including links to both the formal publication through the relevant DOI and the license). See: https://creativecommons.org/licenses/by-nc-nd/4.0/.
References
- Postuma RB, Berg D, Stern M, Poewe W, Olanow CW, Oertel W, Obeso J, Marek K, Litvan I, Lang AE, Halliday G, Goetz CG, Gasser T, Dubois B, Chan P, Bloem BR, Adler CH, Deuschl G. MDS clinical diagnostic criteria for Parkinson's disease. Mov Disord 2015;30:1591-601. [Crossref] [PubMed]
- Aarsland D, Andersen K, Larsen JP, Lolk A, Kragh-Sørensen P. Prevalence and characteristics of dementia in Parkinson disease: an 8-year prospective study. Arch Neurol 2003;60:387-92. [Crossref] [PubMed]
- Aarsland D, Creese B, Politis M, Chaudhuri KR, Ffytche DH, Weintraub D, Ballard C. Cognitive decline in Parkinson disease. Nat Rev Neurol 2017;13:217-31. [Crossref] [PubMed]
- Kehagia AA, Barker RA, Robbins TW. Cognitive impairment in Parkinson's disease: the dual syndrome hypothesis. Neurodegener Dis 2013;11:79-92. [Crossref] [PubMed]
- Mesulam MM, Mufson EJ, Levey AI, Wainer BH. Cholinergic innervation of cortex by the basal forebrain: cytochemistry and cortical connections of the septal area, diagonal band nuclei, nucleus basalis (substantia innominata), and hypothalamus in the rhesus monkey. J Comp Neurol 1983;214:170-97. [Crossref] [PubMed]
- Mesulam MM, Geula C. Nucleus basalis (Ch4) and cortical cholinergic innervation in the human brain: observations based on the distribution of acetylcholinesterase and choline acetyltransferase. J Comp Neurol 1988;275:216-40. [Crossref] [PubMed]
- Hall H, Reyes S, Landeck N, Bye C, Leanza G, Double K, Thompson L, Halliday G, Kirik D. Hippocampal Lewy pathology and cholinergic dysfunction are associated with dementia in Parkinson's disease. Brain 2014;137:2493-508. [Crossref] [PubMed]
- Ray NJ, Bradburn S, Murgatroyd C, Toseeb U, Mir P, Kountouriotis GK, Teipel SJ, Grothe MJ. In vivo cholinergic basal forebrain atrophy predicts cognitive decline in de novo Parkinson's disease. Brain 2018;141:165-76. [Crossref] [PubMed]
- Schulz J, Pagano G, Fernández Bonfante JA, Wilson H, Politis M. Nucleus basalis of Meynert degeneration precedes and predicts cognitive impairment in Parkinson's disease. Brain 2018;141:1501-16. [Crossref] [PubMed]
- Gargouri F, Gallea C, Mongin M, Pyatigorskaya N, Valabregue R, Ewenczyk C, Sarazin M, Yahia-Cherif L, Vidailhet M, Lehéricy S. Multimodal magnetic resonance imaging investigation of basal forebrain damage and cognitive deficits in Parkinson's disease. Mov Disord 2019;34:516-25. [Crossref] [PubMed]
- Therriault J, Ng KP, Pascoal TA, Mathotaarachchi S, Kang MS, Struyfs H, Shin M, Benedet AL, Walpola IC, Nair V, Gauthier S, Rosa-Neto P. Anosognosia predicts default mode network hypometabolism and clinical progression to dementia. Neurology 2018;90:e932-9. [Crossref] [PubMed]
- He Z, Cui Q, Zheng J, Duan X, Pang Y, Gao Q, Han S, Long Z, Wang Y, Li J, Wang X, Zhao J, Chen H. Frequency-specific alterations in functional connectivity in treatment-resistant and -sensitive major depressive disorder. J Psychiatr Res 2016;82:30-9. [Crossref] [PubMed]
- Wang X, Zhang Y, Long Z, Zheng J, Zhang Y, Han S, Wang Y, Duan X, Yang M, Zhao J, Chen H. Frequency-specific alteration of functional connectivity density in antipsychotic-naive adolescents with early-onset schizophrenia. J Psychiatr Res 2017;95:68-75. [Crossref] [PubMed]
- Li J, Cheng L, Chen S, Zhang J, Liu D, Liang Z, Li H. Functional Connectivity Changes in Multiple-Frequency Bands in Acute Basal Ganglia Ischemic Stroke Patients: A Machine Learning Approach. Neural Plast 2022;2022:1560748. [Crossref] [PubMed]
- Wang J, Zuo X, Dai Z, Xia M, Zhao Z, Zhao X, Jia J, Han Y, He Y. Disrupted functional brain connectome in individuals at risk for Alzheimer's disease. Biol Psychiatry 2013;73:472-81. [Crossref] [PubMed]
- Hu H, Chen J, Huang H, Zhou C, Zhang S, Liu X, Wang L, Chen P, Nie K, Chen L, Wang S, Huang B, Huang R. Common and specific altered amplitude of low-frequency fluctuations in Parkinson's disease patients with and without freezing of gait in different frequency bands. Brain Imaging Behav 2020;14:857-68. [Crossref] [PubMed]
- Rong S, Zhang P, He C, Li Y, Li X, Li R, Nie K, Huang S, Wang L, Wang L, Zhang Y. Abnormal Neural Activity in Different Frequency Bands in Parkinson's Disease With Mild Cognitive Impairment. Front Aging Neurosci 2021;13:709998. [Crossref] [PubMed]
- Hughes AJ, Daniel SE, Kilford L, Lees AJ. Accuracy of clinical diagnosis of idiopathic Parkinson's disease: a clinico-pathological study of 100 cases. J Neurol Neurosurg Psychiatry 1992;55:181-4. [Crossref] [PubMed]
- Emre M, Aarsland D, Brown R, Burn DJ, Duyckaerts C, Mizuno Y, et al. Clinical diagnostic criteria for dementia associated with Parkinson's disease. Mov Disord 2007;22:1689-707; quiz 1837. [Crossref] [PubMed]
- Chen KL, Xu Y, Chu AQ, Ding D, Liang XN, Nasreddine ZS, Dong Q, Hong Z, Zhao QH, Guo QH. Validation of the Chinese Version of Montreal Cognitive Assessment Basic for Screening Mild Cognitive Impairment. J Am Geriatr Soc 2016;64:e285-90. [Crossref] [PubMed]
- Goetz CG, Tilley BC, Shaftman SR, Stebbins GT, Fahn S, Martinez-Martin P, et al. Movement Disorder Society-sponsored revision of the Unified Parkinson's Disease Rating Scale (MDS-UPDRS): scale presentation and clinimetric testing results. Mov Disord 2008;23:2129-70. [Crossref] [PubMed]
- Hamilton M. A rating scale for depression. J Neurol Neurosurg Psychiatry 1960;23:56-62. [Crossref] [PubMed]
- Hamilton M. The assessment of anxiety states by rating. Br J Med Psychol 1959;32:50-5. [Crossref] [PubMed]
- Nasreddine ZS, Phillips NA, Bédirian V, Charbonneau S, Whitehead V, Collin I, Cummings JL, Chertkow H. The Montreal Cognitive Assessment, MoCA: a brief screening tool for mild cognitive impairment. J Am Geriatr Soc 2005;53:695-9. [Crossref] [PubMed]
- Litvan I, Goldman JG, Tröster AI, Schmand BA, Weintraub D, Petersen RC, Mollenhauer B, Adler CH, Marder K, Williams-Gray CH, Aarsland D, Kulisevsky J, Rodriguez-Oroz MC, Burn DJ, Barker RA, Emre M. Diagnostic criteria for mild cognitive impairment in Parkinson's disease: Movement Disorder Society Task Force guidelines. Mov Disord 2012;27:349-56. [Crossref] [PubMed]
- Li X, Jiao J, Shimizu S, Jibiki I, Watanabe K, Kubota T. Correlations between atrophy of the entorhinal cortex and cognitive function in patients with Alzheimer's disease and mild cognitive impairment. Psychiatry Clin Neurosci 2012;66:587-93. [Crossref] [PubMed]
- Eickhoff SB, Stephan KE, Mohlberg H, Grefkes C, Fink GR, Amunts K, Zilles K. A new SPM toolbox for combining probabilistic cytoarchitectonic maps and functional imaging data. Neuroimage 2005;25:1325-35. [Crossref] [PubMed]
- Zaborszky L, Hoemke L, Mohlberg H, Schleicher A, Amunts K, Zilles K. Stereotaxic probabilistic maps of the magnocellular cell groups in human basal forebrain. Neuroimage 2008;42:1127-41. [Crossref] [PubMed]
- Tzourio-Mazoyer N, Landeau B, Papathanassiou D, Crivello F, Etard O, Delcroix N, Mazoyer B, Joliot M. Automated anatomical labeling of activations in SPM using a macroscopic anatomical parcellation of the MNI MRI single-subject brain. Neuroimage 2002;15:273-89. [Crossref] [PubMed]
- Rong S, Li Y, Li B, Nie K, Zhang P, Cai T, Mei M, Wang L, Zhang Y. Meynert nucleus-related cortical thinning in Parkinson's disease with mild cognitive impairment. Quant Imaging Med Surg 2021;11:1554-66. [Crossref] [PubMed]
- Power JD, Barnes KA, Snyder AZ, Schlaggar BL, Petersen SE. Spurious but systematic correlations in functional connectivity MRI networks arise from subject motion. Neuroimage 2012;59:2142-54. [Crossref] [PubMed]
- Schumacher J, Thomas AJ, Peraza LR, Firbank M, O'Brien JT, Taylor JP. Functional connectivity of the nucleus basalis of Meynert in Lewy body dementia and Alzheimer's disease. Int Psychogeriatr 2021;33:89-94. [Crossref] [PubMed]
- Oswal A, Gratwicke J, Akram H, Jahanshahi M, Zaborszky L, Brown P, Hariz M, Zrinzo L, Foltynie T, Litvak V. Cortical connectivity of the nucleus basalis of Meynert in Parkinson's disease and Lewy body dementias. Brain 2021;144:781-8. [Crossref] [PubMed]
- Alexandris AS, Walker L, Liu AKL, McAleese KE, Johnson M, Pearce RKB, Gentleman SM, Attems J. Cholinergic deficits and galaninergic hyperinnervation of the nucleus basalis of Meynert in Alzheimer's disease and Lewy body disorders. Neuropathol Appl Neurobiol 2020;46:264-78. [Crossref] [PubMed]
- Shimada H, Hirano S, Shinotoh H, Aotsuka A, Sato K, Tanaka N, Ota T, Asahina M, Fukushi K, Kuwabara S, Hattori T, Suhara T, Irie T. Mapping of brain acetylcholinesterase alterations in Lewy body disease by PET. Neurology 2009;73:273-8. [Crossref] [PubMed]
- Gang M, Baba T, Hosokai Y, Nishio Y, Kikuchi A, Hirayama K, Hasegawa T, Aoki M, Takeda A, Mori E, Suzuki K. Clinical and Cerebral Metabolic Changes in Parkinson's Disease With Basal Forebrain Atrophy. Mov Disord 2020;35:825-32. [Crossref] [PubMed]
- Pereira JB, Hall S, Jalakas M, Grothe MJ, Strandberg O, Stomrud E, Westman E, van Westen D, Hansson O. Longitudinal degeneration of the basal forebrain predicts subsequent dementia in Parkinson's disease. Neurobiol Dis 2020;139:104831. [Crossref] [PubMed]
- Grothe MJ, Labrador-Espinosa MA, Jesús S, Macías-García D, Adarmes-Gómez A, Carrillo F, et al. In vivo cholinergic basal forebrain degeneration and cognition in Parkinson's disease: Imaging results from the COPPADIS study. Parkinsonism Relat Disord 2021;88:68-75. [Crossref] [PubMed]
- Bohnen NI, Kaufer DI, Hendrickson R, Ivanco LS, Lopresti BJ, Constantine GM, Mathis ChA, Davis JG, Moore RY, Dekosky ST. Cognitive correlates of cortical cholinergic denervation in Parkinson's disease and parkinsonian dementia. J Neurol 2006;253:242-7. [Crossref] [PubMed]
- Owen A. Working memory in dorsolateral frontal cortex. Trends Cogn Sci 1998;2:239. [Crossref] [PubMed]
- Rowe JB, Toni I, Josephs O, Frackowiak RS, Passingham RE. The prefrontal cortex: response selection or maintenance within working memory? Science 2000;288:1656-60. [Crossref] [PubMed]
- Cooper JA, Sagar HJ, Doherty SM, Jordan N, Tidswell P, Sullivan EV. Different effects of dopaminergic and anticholinergic therapies on cognitive and motor function in Parkinson's disease. A follow-up study of untreated patients. Brain 1992;115:1701-25. [Crossref] [PubMed]
- Jonkman LE, Fathy YY, Berendse HW, Schoonheim MM, van de Berg WDJ. Structural network topology and microstructural alterations of the anterior insula associate with cognitive and affective impairment in Parkinson's disease. Sci Rep 2021;11:16021. [Crossref] [PubMed]
- Nakano T, Kajiyama Y, Revankar GS, Hashimoto R, Watanabe Y, Kishima H, Ikeda M, Mihara M, Mochizuki H, Hattori N. Neural networks associated with quality of life in patients with Parkinson's disease. Parkinsonism Relat Disord 2021;89:6-12. [Crossref] [PubMed]
- Sarter M, Albin RL, Kucinski A, Lustig C. Where attention falls: Increased risk of falls from the converging impact of cortical cholinergic and midbrain dopamine loss on striatal function. Exp Neurol 2014;257:120-9. [Crossref] [PubMed]
- Taylor JP, Firbank M, O'Brien JT. Visual cortical excitability in dementia with Lewy bodies. Br J Psychiatry 2016;208:497-8. [Crossref] [PubMed]
- Brcina N, Hohenfeld C, Heidbreder A, Mirzazade S, Krahe J, Wojtala J, Binkofski F, Schulz JB, Schiefer J, Reetz K, Dogan I. Increased neural motor activation and functional reorganization in patients with idiopathic rapid eye movement sleep behavior disorder. Parkinsonism Relat Disord 2021;92:76-82. [Crossref] [PubMed]
- Teipel SJ, Meindl T, Grinberg L, Grothe M, Cantero JL, Reiser MF, Möller HJ, Heinsen H, Hampel H. The cholinergic system in mild cognitive impairment and Alzheimer's disease: an in vivo MRI and DTI study. Hum Brain Mapp 2011;32:1349-62. [Crossref] [PubMed]
- Graham AJ, Ray MA, Perry EK, Jaros E, Perry RH, Volsen SG, Bose S, Evans N, Lindstrom J, Court JA. Differential nicotinic acetylcholine receptor subunit expression in the human hippocampus. J Chem Neuroanat 2003;25:97-113. [Crossref] [PubMed]
- Heinsen H, Henn R, Eisenmenger W, Götz M, Bohl J, Bethke B, Lockemann U, Püschel K. Quantitative investigations on the human entorhinal area: left-right asymmetry and age-related changes. Anat Embryol (Berl) 1994;190:181-94. [Crossref] [PubMed]
- Buzsáki G, Draguhn A. Neuronal oscillations in cortical networks. Science 2004;304:1926-9. [Crossref] [PubMed]
- Xue S, Wang X, Wang W, Liu J, Qiu J. Frequency-dependent alterations in regional homogeneity in major depression. Behav Brain Res 2016;306:13-9. [Crossref] [PubMed]
- Baria AT, Baliki MN, Parrish T, Apkarian AV. Anatomical and functional assemblies of brain BOLD oscillations. J Neurosci 2011;31:7910-9. [Crossref] [PubMed]
- Qian L, Zhang Y, Zheng L, Fu X, Liu W, Shang Y, Zhang Y, Xu Y, Liu Y, Zhu H, Gao JH. Frequency specific brain networks in Parkinson's disease and comorbid depression. Brain Imaging Behav 2017;11:224-39. [Crossref] [PubMed]
- Xue SW, Li D, Weng XC, Northoff G, Li DW. Different neural manifestations of two slow frequency bands in resting functional magnetic resonance imaging: a systemic survey at regional, interregional, and network levels. Brain Connect 2014;4:242-55. [Crossref] [PubMed]