Coronary computed tomography angiography assessment of relationship between right coronary artery-aorta angle and the development of coronary artery disease
Introduction
Between 2020 and 2021, an estimated 2.9% of the Australian population aged ≥18 years had coronary artery disease (CAD) (1). CAD is characterised by the formation of atherosclerotic plaques within the coronary arteries, and recent studies have determined that there is a relationship between left coronary artery (LCA) bifurcation angle and the development of CAD as assessed by coronary computed tomography angiography (CCTA) (2,3). Individuals with wide LCA bifurcation angles (>80°) are significantly more likely to develop CAD than those with narrow angles (2-4). To better understand the pathogenesis of CAD, Chaichana et al. used left coronary artery hemodynamics simulations to demonstrate that wide LCA bifurcation angles result in reduced wall shear stress, which is directly correlated with CAD development and atherosclerotic plaque formation (5).
Whilst invasive coronary angiography (ICA) has traditionally been used as the gold standard for coronary vessel assessment, CCTA has proven to be an accurate, less invasive tool for assessing LCA bifurcation angle [mainly left anterior descending (LAD)-left circumflex (LCx)] and diagnosing calcified plaques, demonstrating similar sensitivity, specificity and positive predictive values to those of ICA (6,7). Bifurcation angle assessment via CCTA also provides a reliable, accurate alternative to standard lumen assessment in patients with calcified plaques with improved specificity and positive predictive value (6). Although several studies have established and supported the relationship between LCA bifurcation angle and CAD (2-5), an association between right coronary artery-aorta (RCA-aorta) angle and the development of CAD is yet to be determined. This pilot study, to our knowledge, was the first to investigate such a relationship. Our primary aim was to measure and compare the RCA-aorta angles between normal and abnormal CCTA cases, hypothesising that a correlation exists between RCA-aorta angle and CAD development. We present the following article in accordance with the STROBE reporting checklist (available at https://qims.amegroups.com/article/view/10.21037/qims-22-655/rc).
Methods
Participants
This study included the CCTA datasets of 30 normal and 30 CAD cases, and patient demographics are summarised in Table 1. The 30 normal cases were randomly selected from a previous study serving as the control group, with CCTA scans performed on a 320-slice CT (3), whilst the 30 CAD cases were also randomly obtained from another previous study serving as the study group with CCTA scans performed on a first and second-generation dual source CT and 320-slice scanner, respectively (6). Normal CCTA cases were identified using radiology reports and did not undergo ICA, due to the high negative predictive value of CCTA, whereas ICA was performed in all CAD cases to ascertain the degree of coronary stenosis (denoted as either <50% or ≥50% stenosis). This study was conducted in accordance with the Declaration of Helsinki (as revised in 2013) and was approved by the Curtin University Human Research Ethics Committee. Individual consent for this retrospective analysis was waived.
Table 1
Characteristics | Normal group (n=30) | CAD group (n=30) |
---|---|---|
Age (years), mean ± SD | 61.7±9.1 | 67.5±10.3 |
M/F, n | 19/11 | 24/6 |
Coronary artery involvement, n (%) | ||
1-vessel disease | N/A | 5 (16.7) |
2-vessel disease | N/A | 18 (60.0) |
3-vessel disease | N/A | 7 (23.3) |
Diabetes | 42.9% | 52% |
Hypertension | 56.1% | 88% |
Smoking history | 50% | 74% |
Family history | 58.5% | 42% |
Distribution of calcified plaques at coronary arteries | ||
LAD (n=30), n (%) | ||
1–3 plaques | N/A | 26 (86.7) |
4–5 plaques | N/A | 4 (13.3) |
LCx (n=15), n (%) | ||
1–3 plaques | N/A | 15 (100.0) |
4–5 plaques | N/A | 0 |
RCA (n=11), n (%) | ||
1–3 plaques | N/A | 11 (100.0) |
4–5 plaques | N/A | 0 |
CAD, coronary artery disease; SD, standard deviation; M, male; F, female; N/A, not applicable; LAD, left anterior descending; LCx, left circumflex artery; RCA, right coronary artery.
Coronary angle measurements
RCA-aorta angle measurements were obtained via multiplanar reformation (MPR) images, using variable, obliqued planes to ascertain the best observable RCA-aorta angle for each case. Axial measurements were also acquired, to determine whether this is a valid method of analysing RCA-aorta angle, since current literature is yet to explore this technique due to lack of studies documenting this aspect. Additionally, the LAD-LCx angles were measured using both 2D axial images and MPR images, since the accuracy of LAD-LCx angles measured on 2D axial images is similar to those measured on 3D views (4). Measurements were conducted by two independent assessors to determine measurement reliability.
Statistical analysis
Data were entered into SPSS V 27.0 for statistical analysis, with continuous variables expressed as mean ± standard deviation (SD). A one sample t-test was used to assess for a significant mean difference between MPR and axially-acquired RCA-aorta measurements, and a Bland-Altman plot was produced to assess overall agreeance for the two measurement techniques. An independent sample t-test was used to compare the differences in coronary angles between the normal and CAD group, and analysis of variance (ANOVA) was used to assess for significant differences between coronary angles in normal and CAD subgroups (individuals with <50% and ≥50% coronary stenosis). P values ≤0.05 indicated statistical significance. Agreement between the measurements of the two independent assessors was determined using an intraclass correlation coefficient.
Results
Despite the high prevalence of calcified plaques seen in the RCA, and LAD and LCx arteries, less than half of CAD cases were confirmed to have ≥50% stenosis on ICA (Table 2). There were significant differences in both the right and left coronary angle measurements, with a significantly larger mean RCA-aorta angle observed in the normal case group compared to the diseased group (Figure 1) (P=0.05). Figure 2 shows the distribution of RCA-aorta angle between normal and CAD groups. The mean RCA-aorta angle of the normal group was larger than that of the CAD group, with a greater number of cases exhibiting angles of 100° or higher in the normal group (11 cases in the normal group vs. 6 cases in the CAD group) as depicted by the histograms (Figure 2) and Table 3.
Table 2
Disease prevalence | Calcified plaques on CCTA | Degree of stenosis ≥50% on CCTA | Degree of stenosis ≥50% on ICA | ||||||||
---|---|---|---|---|---|---|---|---|---|---|---|
RCA | LAD | LCx | RCA | LAD | LCx | RCA | LAD | LCx | |||
No. of cases | 27 | 30 | 21 | 18 | 29 | 15 | 5 | 15 | 4 | ||
Percentage | 90% | 100% | 70% | 60% | 96.7% | 50% | 16.7% | 50% | 13.3% |
CCTA, coronary computed tomography angiography; ICA, invasive coronary angiography; RCA, right coronary artery; LAD, left anterior descending; LCx, left circumflex.
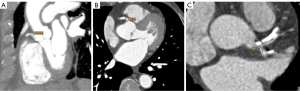
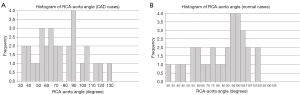
Table 3
Measurements | Normal group (n=30) | CAD group (n=30) | P value | |||
---|---|---|---|---|---|---|
RCA-aorta angle | LAD-LCx angle | RCA-aorta angle | LAD-LCx angle | |||
Mean (95% CI) | 87.47° (79.31° to 95.78°) | 59.2° (50.39° to 68.75°) | 76.82° (67.82° to 85.61°) | 79.5° (69.72° to 89.09°) | 0.05; 0.002* | |
Range | 33.2° to 123.3° | 26.1° to 130.0° | 38.2° to 120.3° | 37.9° to 136.6° | N/A | |
>100° for RCA angle, >80° for LCA angle, n (%) | 11 (36.7) | 5 (16.7) | 6 (20.0) | 16 (53.3) | N/A | |
≥50% vs. <50% stenosis, mean (95% CI) | N/A | N/A | 80.18° (55.46° to 102.50°) vs. 76.14° (66.46° to 86.24°) | 89.5° (72.83° to 103.42°) vs. 65.8° (54.99° to 75.38°) | 0.75; 0.01# |
*, indicates significant differences between normal and CAD groups for both angle measurements; #, indicates comparison for RCA-aorta and LAD-LCx angles between more than 50% and less than 50% stenosis, respectively. RCA, right coronary artery; LAD, left anterior descending; LCx, left circumflex; CAD, coronary artery disease; CI, confidence interval; N/A, not applicable; LCA, left coronary artery.
There was a significant mean difference between MPR and axially-acquired RCA-aorta measurements (27.64°±23.33°, P<0.001) (Figure 3), and the associated Bland-Altman plot suggests no agreement between the two measurement techniques (Figure 4). However, there was strong intraclass correlation between the two independent assessor measurements for both LAD-LCx (r=0.984–0.998, P<0.001) and MPR (r=0.971–0.993, P<0.001) and axially-acquired RCA-aorta angle measurements (r=0.994–0.998, P<0.001).
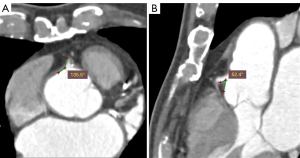
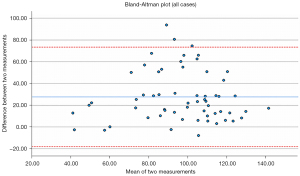
Conversely, a significantly smaller mean LAD-LCx angle was observed in the normal group compared to the CAD group (P=0.002), as presented in Table 3. Subgroup analysis between normal cases and CAD cases with <50% and ≥50% coronary stenosis yielded no significant difference regarding RCA-aorta angle (P=0.75). However, individuals with ≥50% stenosis had a significantly larger mean LAD-LCx angle (>80°) compared to those with <50% stenosis (P=0.01).
Discussion
This study provides further evidence supporting the relationship between wide LCA-LCx and CAD development, as suggested by several previous studies (2-6). Furthermore, our preliminary results show the correlation between RCA-aorta angle and CAD with a smaller angle observed in the CAD group compared to the normal group. Thus, measurement of RCA-aorta angle could be included in the diagnostic approach of CCTA in CAD to provide additional information such as prediction or risk stratification of CAD.
LAD-LCx angle measurement via axial and MPR images has been shown to be of similar accuracy to that measured on 3D views (4). However, there was a significant positive mean difference between MPR and axially-acquired RCA-aorta measurements (27.64°±23.33°, P<0.001). This suggests a consistent overestimation of RCA-aorta angle when assessed on axial views alone (Figure 3), since the accuracy of this method is probably largely dependent on RCA trajectory, unlike the LAD-LCx angle which can be accurately measured using axial and MPR images (2-4). Additionally, the associated Bland-Altman plot suggests no agreement between MPR and axially-acquired measurements (Figure 4). Hence, valid analysis of this angle requires 3D assessment, which cannot be achieved using only one anatomical plane. Although MPR can facilitate a 3D evaluation of the RCA-aorta angle, due to limited number of cases analysed in this study, further research is required to determine whether the results of this method of assessment is comparable to that of 3D volume rendering, in order to ascertain the most accurate, reliable method of measuring this coronary angle.
There was a discrepancy between the apparent degree of coronary stenosis on CCTA vs. ICA, indicating that CCTA yields a high false positive rate due to blooming artifacts associated with extensive calcification, which is within expectation. This supports the findings of various existing studies exploring the characteristics of blooming artifacts associated with severe coronary calcification. The appearance of severe coronary stenosis is frequently exacerbated on CCTA due to partial volume averaging, impeding the accuracy of consequent lumen assessment (8-10). The limitations of CCTA in diagnosing calcified plaques have been addressed and overcome to some extent via several methods, such as dual-energy CT (11), image subtraction algorithms (12-14), iterative reconstruction (15,16), high-definition CT (17-19), and deep learning (DL) approaches (20-24). Of these methods, using DL seems to be a promising strategy to improve diagnostic value of CCTA in assessing calcified plaques, however, incorporation of coronary angle measurements into routine coronary lumen assessment could be a simple and convenient approach, according to this and other reports (2-7,25,26).
Most studies available in the existing literature focus on the correlation between LAD-LCx and CAD (2-7,25,26). There is no current research investigating possible relationships between RCA-aorta angle and CAD. This could be due to the fact that the RCA path is relatively straight, unlike the left coronary artery and its bifurcation angle, which results in hemodynamic changes such as low wall shear stress and flow turbulence due to wide angulation. By contrast, smaller RCA-aorta angles may result in unstable hemodynamics in the proximal RCA, reducing wall shear stress, which could damage the intimal coronary wall and lead to atherosclerosis (27). This pilot study has supported our hypothesis, and its results lay foundation for further research in this direction. Specifically, research investigating hemodynamic changes using computational fluid dynamics between RCA-aorta angle and CAD development is necessary to elaborate on this relationship.
Additionally, our findings indicate that there is likely a correlation between narrow RCA-aorta angle and the development of CAD, suggesting that RCA-aorta angle assessment may be incorporated into diagnostic evaluation for patients with calcified plaques to enhance the diagnostic accuracy of CCTA. However, subgroup analysis did not yield any significant differences between the RCA-aorta angles of normal cases, or those with <50% and ≥50% coronary stenosis. Degree of coronary stenosis is commonly used in the current literature to characterise CAD severity (25,26,28-30). However, this alone is not necessarily indicative of ischemic severity, which is an inherent limitation for CCTA, as the degree of coronary stenosis does not always translate to the hemodynamic significance of coronary lesions. Recent studies suggest the diagnostic accuracy of CT-derived fractional flow reserve (FFRCT) may more accurately correspond to ischemic severity, and hence guide patient treatments more effectively (31,32). Consequently, future studies should aim to correlate coronary angles with changes in physiology, characterising CAD severity with tools such as FFR, in order to better understand these relationships.
This study has several limitations. This was a pilot study involving a small sample size and further studies with larger sample sizes are necessary to validate our results. Secondly, although our findings suggest a relationship between narrow RCA-aorta angle and CAD, this study’s CAD group consisted only of patients with calcified plaques. Further, only a very small number of cases had significant RCA stenosis indicating another limitation of the diseased group. Subsequent studies should include patients with different plaque types, and of different risk groups (low, intermediate and high risk) in order for more robust, comprehensive conclusions to be formed. Cardiac CT radiomics is an emerging area enabling quantitative analysis of plaque features for cardiac risk prediction and prevention (33,34), thus this could be a future research direction with inclusion of RCA-aorta angle as another variable in the CT radiomics analysis.
In conclusion, this preliminary study suggests there may be a correlation between RCA-aorta angle and the development of CAD. Measurements of coronary angles, including both RCA-aorta and LAD-LCx could be incorporated into routine diagnostic CCTA to improve its diagnostic value. Further studies involving large sample sizes, in particular, testing different approaches of measuring RCA-aorta angles are warranted to validate our findings.
Acknowledgments
Funding: None.
Footnote
Reporting Checklist: The authors have completed the STROBE reporting checklist. Available at https://qims.amegroups.com/article/view/10.21037/qims-22-655/rc
Conflicts of Interest: Both authors have completed the ICMJE uniform disclosure form (available at https://qims.amegroups.com/article/view/10.21037/qims-22-655/coif). ZS serves as an unpaid associate editor of Quantitative Imaging in Medicine and Surgery. The other author has no conflicts of interest to declare.
Ethical Statement:
Open Access Statement: This is an Open Access article distributed in accordance with the Creative Commons Attribution-NonCommercial-NoDerivs 4.0 International License (CC BY-NC-ND 4.0), which permits the non-commercial replication and distribution of the article with the strict proviso that no changes or edits are made and the original work is properly cited (including links to both the formal publication through the relevant DOI and the license). See: https://creativecommons.org/licenses/by-nc-nd/4.0/.
References
- Australian Institute of Health and Welfare. Australian Government. Australia’s health 2020. Available online: https://www.aihw.gov.au/reports/australias-health/coronary-heart-disease
- Sun Z, Cao Y. Multislice CT angiography assessment of left coronary artery: correlation between bifurcation angle and dimensions and development of coronary artery disease. Eur J Radiol 2011;79:e90-5. [Crossref] [PubMed]
- Temov K, Sun Z. Coronary computed tomography angiography investigation of the association between left main coronary artery bifurcation angle and risk factors of coronary artery disease. Int J Cardiovasc Imaging 2016;32:129-37. [Crossref] [PubMed]
- Juan YH, Tsay PK, Shen WC, Yeh CS, Wen MS, Wan YL. Comparison of the Left Main Coronary Bifurcating Angle among Patients with Normal, Non-significantly and Significantly Stenosed Left Coronary Arteries. Sci Rep 2017;7:1515. [Crossref] [PubMed]
- Chaichana T, Sun Z, Jewkes J. Computation of hemodynamics in the left coronary artery with variable angulations. J Biomech 2011;44:1869-78. [Crossref] [PubMed]
- Sun Z, Xu L, Fan Z. Coronary CT angiography in calcified coronary plaques: Comparison of diagnostic accuracy between bifurcation angle measurement and coronary lumen assessment for diagnosing significant coronary stenosis. Int J Cardiol 2016;203:78-86. [Crossref] [PubMed]
- Geerlings-Batt J, Sun Z. Evaluation of the Relationship between Left Coronary Artery Bifurcation Angle and Coronary Artery Disease: A Systematic Review. J Clin Med 2022;11:5143. [Crossref] [PubMed]
- Hoffmann U, Ferencik M, Cury RC, Pena AJ. Coronary CT angiography. J Nucl Med 2006;47:797-806. [PubMed]
- Kruk M, Noll D, Achenbach S, Mintz GS, Pręgowski J, Kaczmarska E, Kryczka K, Pracoń R, Dzielińska Z, Sleszycka J, Witkowski A, Demkow M, Rużyłło W, Kępka C. Impact of coronary artery calcium characteristics on accuracy of CT angiography. JACC Cardiovasc Imaging 2014;7:49-58. [Crossref] [PubMed]
- Yan RT, Miller JM, Rochitte CE, Dewey M, Niinuma H, Clouse ME, Vavere AL, Brinker J, Lima JA, Arbab-Zadeh A. Predictors of inaccurate coronary arterial stenosis assessment by CT angiography. JACC Cardiovasc Imaging 2013;6:963-72. [Crossref] [PubMed]
- Yunaga H, Ohta Y, Kishimoto J, Kitao S, Ishibashi M, Fujii S. Effect of energy difference in the evaluation of calcification size and luminal diameter in calcified coronary artery plaque using spectral CT. Jpn J Radiol 2020;38:1142-9. [Crossref] [PubMed]
- Sun Z, Ng CKC. High calcium scores in coronary CT angiography: effects of image post-processing on visualization and measurement of coronary lumen diameter. J Med Imaging Health Inform 2015;5:110-6. [Crossref]
- Li P, Xu L, Yang L, Wang R, Hsieh J, Sun Z, Fan Z, Leipsic JA. Blooming Artifact Reduction in Coronary Artery Calcification by A New De-blooming Algorithm: Initial Study. Sci Rep 2018;8:6945. [Crossref] [PubMed]
- Sun Z, Ng CKC, Xu L, Fan Z, Lei J, Coronary CT. Angiography in Heavily Calcified Coronary Arteries: Improvement of Coronary Lumen Visualization and Coronary Stenosis Assessment With Image Postprocessing Methods. Medicine (Baltimore) 2015;94:e2148. [Crossref] [PubMed]
- van Osch JA, Mouden M, van Dalen JA, Timmer JR, Reiffers S, Knollema S, Greuter MJ, Ottervanger JP, Jager PL. Influence of iterative image reconstruction on CT-based calcium score measurements. Int J Cardiovasc Imaging 2014;30:961-7. [Crossref] [PubMed]
- Funabashi N, Irie R, Aiba M, Morimoto R, Kabashima T, Fujii S, Uehara M, Ozawa K, Takaoka H, Kobayashi Y. Adaptive-Iterative-Dose-Reduction 3D with multisector-reconstruction method in 320-slice CT may maintain accurate-measurement of the Agatston-calcium-score of severe-calcification even at higher pulsating-beats and low tube-current in vitro. Int J Cardiol 2013;168:601-3. [Crossref] [PubMed]
- Pontone G, Bertella E, Mushtaq S, Loguercio M, Cortinovis S, Baggiano A, Conte E, Annoni A, Formenti A, Beltrama V, Guaricci AI, Andreini D. Coronary artery disease: diagnostic accuracy of CT coronary angiography--a comparison of high and standard spatial resolution scanning. Radiology 2014;271:688-94. [Crossref] [PubMed]
- Latina J, Shabani M, Kapoor K, Whelton SP, Trost JC, Sesso J, Demehri S, Mahesh M, Lima JAC, Arbab-Zadeh A. Ultra-High-Resolution Coronary CT Angiography for Assessment of Patients with Severe Coronary Artery Calcification: Initial Experience. Radiol Cardiothorac Imaging 2021;3:e210053. [Crossref] [PubMed]
- Schuijf JD, Lima JAC, Boedeker KL, Takagi H, Tanaka R, Yoshioka K, Arbab-Zadeh A. CT imaging with ultra-high-resolution: Opportunities for cardiovascular imaging in clinical practice. J Cardiovasc Comput Tomogr 2022;16:388-96. [Crossref] [PubMed]
- Tatsugami F, Higaki T, Nakamura Y, Yu Z, Zhou J, Lu Y, Fujioka C, Kitagawa T, Kihara Y, Iida M, Awai K. Deep learning-based image restoration algorithm for coronary CT angiography. Eur Radiol 2019;29:5322-9. [Crossref] [PubMed]
- Benz DC, Benetos G, Rampidis G, von Felten E, Bakula A, Sustar A, Kudura K, Messerli M, Fuchs TA, Gebhard C, Pazhenkottil AP, Kaufmann PA, Buechel RR. Validation of deep-learning image reconstruction for coronary computed tomography angiography: Impact on noise, image quality and diagnostic accuracy. J Cardiovasc Comput Tomogr 2020;14:444-51. [Crossref] [PubMed]
- Sun Z, Ng CKC. Artificial Intelligence (Enhanced Super-Resolution Generative Adversarial Network) for Calcium Deblooming in Coronary Computed Tomography Angiography: A Feasibility Study. Diagnostics (Basel) 2022;12:991. [Crossref] [PubMed]
- Inage H, Tomizawa N, Otsuka Y, Aoshima C, Kawaguchi Y, Takamura K, Matsumori R, Kamo Y, Nozaki Y, Takahashi D, Kudo A, Hiki M, Kogure Y, Fujimoto S, Minamino T, Aoki S. Use of a deep-learning-based lumen extraction method to detect significant stenosis on coronary computed tomography angiography in patients with severe coronary calcification. Egypt Heart J 2022;74:43. [Crossref] [PubMed]
- Sun Z, Ng CKC. Finetuned Super-Resolution Generative Adversarial Network (Artificial Intelligence) Model for Calcium Deblooming in Coronary Computed Tomography Angiography. J Pers Med 2022;12:1354. [Crossref] [PubMed]
- Cui Y, Zeng W, Yu J, Lu J, Hu Y, Diao N, Liang B, Han P, Shi H. Quantification of left coronary bifurcation angles and plaques by coronary computed tomography angiography for prediction of significant coronary stenosis: A preliminary study with dual-source CT. PLoS One 2017;12:e0174352. [Crossref] [PubMed]
- Moon SH, Byun JH, Kim JW, Kim SH, Kim KN, Jung JJ, Kang DH, Yang JH, Choi JY, Jang IS, Park HO, Lee CE, Ahn JH. Clinical usefulness of the angle between left main coronary artery and left anterior descending coronary artery for the evaluation of obstructive coronary artery disease. PLoS One 2018;13:e0202249. [Crossref] [PubMed]
- Mishani S, Belhoul-Fakir H, Lagat C, Jansen S, Evans B, Lawrence-Brown M. Stress distribution in the walls of major arteries: implications for atherogenesis. Quant Imaging Med Surg 2021;11:3494-505. [Crossref] [PubMed]
- Rudziński PN, Kruk M, Kępka C, Schoepf UJ, Otani K, Leonard TJ, Dębski M, Dzielińska Z, Pręgowski J, Witkowski A, Rużyłło W, Demkow M. Assessing the value of coronary artery computed tomography as the first-line anatomical test for stable patients with indications for invasive angiography due to suspected coronary artery disease. Initial cost analysis in the CAT-CAD randomized trial. J Cardiovasc Comput Tomogr 2020;14:75-9. [Crossref] [PubMed]
- Rudziński PN, Kruk M, Kępka C, Schoepf UJ, Duguay T, Dzielińska Z, Pręgowski J, Witkowski A, Rużyłło W, Demkow M. The value of Coronary Artery computed Tomography as the first-line anatomical test for stable patients with indications for invasive angiography due to suspected Coronary Artery Disease: CAT-CAD randomized trial. J Cardiovasc Comput Tomogr 2018;12:472-9. [Crossref] [PubMed]
- Rudziński PN, Kruk M, Demkow M, Oleksiak A, Schoepf JU, Mach M, Dzielińska Z, Pręgowski J, Witkowski A, Rużyłło W, Kępka C. Efficacy and safety of coronary computed tomography angiography in patients with a high clinical likelihood of obstructive coronary artery disease. Kardiol Pol 2022;80:56-63. [Crossref] [PubMed]
- Koo BK, Erglis A, Doh JH, Daniels DV, Jegere S, Kim HS, Dunning A, DeFrance T, Lansky A, Leipsic J, Min JK. Diagnosis of ischemia-causing coronary stenoses by noninvasive fractional flow reserve computed from coronary computed tomographic angiograms. Results from the prospective multicenter DISCOVER-FLOW (Diagnosis of Ischemia-Causing Stenoses Obtained Via Noninvasive Fractional Flow Reserve) study. J Am Coll Cardiol 2011;58:1989-97. [Crossref] [PubMed]
- Ko BS, Cameron JD, Munnur RK, Wong DTL, Fujisawa Y, Sakaguchi T, Hirohata K, Hislop-Jambrich J, Fujimoto S, Takamura K, Crossett M, Leung M, Kuganesan A, Malaiapan Y, Nasis A, Troupis J, Meredith IT, Seneviratne SK. Noninvasive CT-Derived FFR Based on Structural and Fluid Analysis: A Comparison With Invasive FFR for Detection of Functionally Significant Stenosis. JACC Cardiovasc Imaging 2017;10:663-73. [Crossref] [PubMed]
- Shang J, Guo Y, Ma Y, Hou Y. Cardiac computed tomography radiomics: a narrative review of current status and future directions. Quant Imaging Med Surg 2022;12:3436-53. [Crossref] [PubMed]
- Yuan M, Wu H, Li R, Yu M, Dai X, Zhang J. The value of quantified plaque analysis by dual-source coronary CT angiography to detect vulnerable plaques: a comparison study with intravascular ultrasound. Quant Imaging Med Surg 2020;10:668-77. [Crossref] [PubMed]