Schmorl’s nodes are associated with prevalent osteoporotic vertebral fracture and low bone mineral density: a population-based thoracic spine MRI study in older men and women
Introduction
In 1927, pathologist G. Schmorl (1) described a type of vertebral lesion which is now known as Schmorl’s node (SN). SN corresponds histologically to nucleus pulposus herniation into the vertebral spongy bone with thickened trabeculae around the formed node. A fair amount of research efforts has been conducted to characterize SN, yet their pathogenesis, clinical significance, and management remain still in debate (2,3). Although frequently incidental and asymptomatic, some SNs can cause back pain and negatively impact the patient’s quality of life (3-7). Histologically, endplate defects are associated with endplate pathology and are more innervated than disc pathology, and thus SN can be symptomatic. SNs that show enhanced magnetic resonance imaging (MRI) signal intensity after intravenous administration of a gadolinium-based contrast agent and those accompanied by bone marrow changes are more likely associated with back pain (7,8).
A number of theories addressing SN’s pathogenesis have been proposed, but no consensus currently exists (2). Overall, the physiopathology of SN formation is believed to be multifactorial. The vertebral endplate is a barrier with a two-layer structure (bony and cartilaginous) between the vertebra and the disc. The theory of weak spot presence within vertebral central endplate has been considered. Certain pre-existing conditions can facilitate herniation occurs due to axial forces. The possible endplate weakness can be due to notochord regression, ossification gaps, or vascular channels (2,3,9-11). A developmental defect can result in an indentation in the vertebral endplate into which nucleus pulposus herniation can readily occur if sufficient axial force is experienced or accumulated over time. Dar et al. (9) proposed an axial load model which suggests that the thoracolumbar spine bears much axial stress and is relatively mobile, it may accumulate micro-traumas that can, over time, lead to the formation of SN. Trauma and endplate micro-fractures are considered triggers for SN (12-14). Heritability of SN has also been demonstrated (15,16).
Recently, endplate fracture has been acknowledged as an important phenomenon of osteoporotic vertebral fracture (OVF) (17-19). In vertebral osteoporosis, the endplate becomes weakened due to the loss of support from trabecular bone, and due to thinning of the endplate itself (20). In their ex vivo specimen analysis, Pfirrmann and Resnick (21) noted endplate fracture existed more likely in the endplate with SN than in those endplates without SN. In our experience in reading medical images of older subjects, we commonly observed SN and endplate fracture co-exist (Figures 1,2). We empirically believe it is highly possible that osteoporosis leads to a weakened bony endplate, this weakened bony endplate together with possible developmental weak spots (if exist) lead to the genesis of SN, and then SN predisposes an osteoporotic endplate to fractures, i.e., there is a pathway that: osteoporosis → weakened endplate → SN development → endplate fracture of an osteoporotic vertebra. Or, endplate structural weakness predisposes to both Schmorl’s and OVF, and hence can lead to one, the other, or both. If this is true, then we would expect SNs are correlated with greater age, correlated with lower BMD status, correlated with OVF severity, and SNs would be much more prevalent in older women and in older men. SN is the most common endplate lesion (2,3). However, radiograph can only detect old SNs of great size, with the least prevalence of 3.8% to 8.1% was observed with plain radiography (2). This might have led to SN being under-researched in osteoporosis literature. On the other hand, cadaveric studies reported a SN prevalence of 40–76% (2). MRI analysis also reported much higher SN prevalence than those of radiography (4,15,22,23). As cross-sectional imaging is increasingly used in osteoporosis evaluation, it is highly the time to investigate the role of SN in OVF. During our 14-year follow-up of MrOS (Hong Kong) and MsOS (Hong Kong) studies, we sampled spine MRI for some of the follow-up participants (24,25). Using these MRI data, the primary aim of this study was to evaluate the possible correlation between SN and OVF in the thoracic spine, and we also evaluated the correlations of SN with a few other selected features of thoracic spine degeneration.
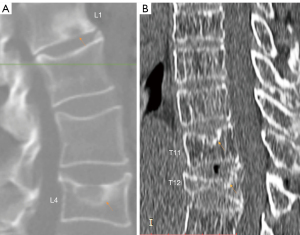
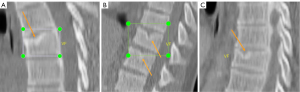
Methods
Osteoporotic fractures in men (MrOS) and in women (MsOS) Hong Kong represent the first large-scale prospective cohort study conducted on bone health in East Asians. At baseline, 2,000 Chinese men and 2,000 Chinese women ≥65 years were recruited from the local communities in Hong Kong from August 2001 to March 2003. The recruitment criteria were structured so that the study results from the cohort would be applicable to a broad population of similarly aged community-dwelling men and women. The project was designed primarily to examine the bone mineral density (BMD) of older Chinese adults prospectively for 4 years. All participants were community dwelling, able to walk without assistance, had no bilateral hip replacement, and had the potential to survive the duration of a primary study based on their general medical health. No participants had a known malignancy or previous lumbar spine surgery. During 2014–2017, a 14-year follow-up was carried out, and whole spine MRI performed on 271 men and 151 women. The study was conducted in accordance with the Declaration of Helsinki (as revised in 2013). The study protocol was approved by the Chinese University of Hong Kong Ethics Committee. Written informed consent was obtained from all subjects.
The selection for MRI participants was random with a potential bias for more mobile subjects as they were more willing to participate. MRI was conducted using a Philips Achieva TX 3.0T scanner equipped with a dual transmitter (Philips Healthcare, Best, the Netherlands). The subjects were scanned in the supine position, with the body coil used as the radiofrequency signal transmitter and a dedicated spine coil used as the signal receiver. T2-weighted sagittal image was acquired with the following imaging parameters: 2-dimensional (2D) turbo spin echo sequence, repetition time (TR) =3,000 ms, echo time (TE) =120 ms, flip angle =90, slice thickness =3.5 mm, inter-slice gap =0.4 mm, resolution =1.0×0.8 mm2, and number of excitations (NEX) =1. All MRI was performed in Saturday morning. The study was designed so that men would be examined firstly. Due to a logistical disruption during the study, this Saturday morning MRI time became no longer available; thus, at the close of this study, more men were scanned than women. One women participant’s MRI demonstrated metastatic tumors in the spine, and one male participant’s MRI demonstrated low image quality, thus these two subjects were excluded. None of the subjects included in analysis were diagnosed as having pathological fractures or diseases other than degenerative or osteoporotic change. The MRI analysis for cervical spine degeneration, and OVF analysis for thoracic and lumbar spine had been reported previously (24,25).
On the thoracic spine MRI reading, the primary item assessed was SN, other spine degenerative changes assessed included Modic change, intervertebral disc posterior budging or protrusion or extrusion, ligamentum flavum ossification (LFO), and spinal canal stenosis. In addition, thoracic spine curvature change was also recorded. SN, Modic change, and spine curvature change were assessed as abnormality existed (=‘1’) or not existed (=‘0’). For posterior disc protrusion, LFO, and spinal canal stenosis, semi-quantitative gradings were applied (criteria shown in Table 1). SN and Modic change were scored from vertebra T1 to vertebra T12 with eighter ‘1’ or ‘0’, and for each subject the summed value was obtained. Posterior disc protrusion, LFO, and spinal canal stenosis were scored from level T1/T2 to level T12/L1, and for each subject the summed value was also obtained. As only T2 weighted MR images were acquired, sub-typing of Modic change was not evaluated. Out of conveniency, together with Modic change, intervertebral disc change, LFO, and spinal canal stenosis, SN was also termed as one of the spine degenerations. The assessment was conducted by two radiologists (YXJW, XRW) in consensus.
Table 1
Assessed items | Grading criteria |
---|---|
Schmorl’s node | 0: none; 1: Schmorl’s node exists |
Modic change | 0: none; 1: Modic change exists |
posterior disc protrusion | 0: normal appearance or minimal bulging; 1: disc bulging; 2: disc protrusion; 3: disc extrusion or sequestration |
Ligamentum flavum hypertrophy | 0: normal or mild subarachnoid space narrowing; 1: apparent posterior subarachnoid space but without spinal cord deformity; 2: canal stenosis with spinal cord compression |
spinal canal stenosis | 0: normal or mild subarachnoid space narrowing; 1: apparent anterior subarachnoid space but without spinal cord compression deformity; 2: canal stenosis with spinal cord compression |
Spine curvature | 0: normal; 1: altered curvature (kyphotic or straightened) |
MRI, magnetic resonance imaging.
For radiological OVF, we re-used the readings we reported earlier (25). The thoracic vertebrae (T1–T12) were evaluated with an extended version of semi-quantitative (SQ) criteria with the following criteria (25,26): (I) minimal grade refers to radiological osteoporotic vertebral deformities (OVDs) with <1/5 height loss, which would be theoretically equivalent to SQ grade 0.5; (II) mild grade is the same as Genant SQ mild grade (1/5–1/4 height loss); (III) SQ moderate grade is divided into two subgrades: ≥1/4–1/3 height loss and ≥1/3–2/3 height loss; (IV) SQ severe grade is divided into two subgrades: ≥2/5–2/3 height loss and with ≥2/3 height loss (collapsed grade). Nonfractural changes of the vertebrae shape were systematically differentiated from OVF (27,28). An OVF sum score (OVFss) was calculated for each subject. For each vertebra in women, a score of 0, 0.5, 1, 1.5, 2, 2.5, and 3 was assigned for no OVF or OVF of <1/5, ≥1/5–1/4, ≥1/4–1/3, ≥1/3–2/5, ≥2/5–2/3, and ≥2/3 vertebral height loss, respectively, and OVFss was calculated by summing up the scores of all thoracic vertebrae (T1 to T12). For men, those of minimal grade were not considered as OVF and assigned a ‘0’ score (25,29).
Among the study subjects, 260 men and 141 women had BMD measured at the 14-year follow-up (scanner: QDR-4500 W densitometer, Hologic Inc., Waltham, MA, USA). It is known that spine degeneration changes can affect the precision of spine BMD measurement. As higher degree of degenerative changes, which can lead to artificially higher BMD values, may co-exist with OVF, thus only femoral neck BMD data were utilised in this study.
All statistical analyses were performed using GraphPad Software (San Diego, CA, USA). Continuous variables were tested by Student’s t-test and categorical variables were analysed by chi-square test. Strength of correlation was evaluated by using the Pearson correlation coefficient (r). A P value of less than 0.05 was considered statistically significant, while a value of less than 0.1 was considered marginally significant.
Results
Thoracic spine MR images were analysed in 270 men (mean: 82.9±3.7 years; range, 77–99 years) and 150 women (mean: 81.5±4.3 years; range, 74–100 years), with men being slightly older than women (P<0.001). 25.9% of the men and 55.5% of the women had SN (Figure 3). The prevalence of spine changes was all more prevalent among women than among men (Table 2), while the male-female difference was the greatest for SN. For the severity of those positive cases, women appeared to be overall slightly more severe except for Modic change. For the cases with the highest degeneration scores, women appeared to be more heavily affected (Table 2).
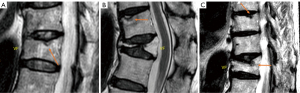
Table 2
Degeneration changes | Men | Women | P values | |||||||
---|---|---|---|---|---|---|---|---|---|---|
Prevalence | M score | Top 8 score | Prevalence | M score | Top 5 score | P# | P§ | |||
Schmorl’s node | 25.9% (70/270) | 2.529 | 5.88 | 55.5% (86/155)* | 2.674 | 7.2 | <0.0001 | >0.05 | ||
Modic change | 29.6% (80/270) | 2.200 | 5.25 | 39.4% (61/155)* | 2.164 | 5.0 | <0.5 | >0.05 | ||
Disc protrusion | 31.5% (85/270) | 2.659 | 7.63 | 37.4% (58/155) | 3.052 | 8.4 | >0.05 | >0.05 | ||
Ligamentum flavum hypertrophy | 41.5% (112/270) | 2.277 | 7.13 | 60.6% (94/155)* | 2.840* | 7.4 | <0.001 | 0.02 | ||
Spinal canal narrowing | 34.8% (94/270) | 2.415 | 6.75 | 45.2% (70/155)* | 2.621 | 7.0 | <0.5 | >0.05 | ||
Spine curvature change | 11.5% (30/270) | – | – | 14.2% (22/155) | – | – | >0.05 | – |
*, male female difference achieved statistical difference for prevalence; #, male female difference on prevalence; §, male female difference on M score. M score: mean score of the positive cases. Top 8 score: the mean score of the 8 cases (out of 270 male subjects) with the highest degeneration change score. Top 5 score: the mean score of the 5 cases (out of 155 female subjects) with the highest degeneration change score. MRI, magnetic resonance imaging.
The vertebral level distributions of OVF and SN are shown in Figure 4. The vertebral level distributions of Modic change, posterior disc protrusion, LFO and spinal canal stenosis are shown in Figure 5. Overall, the mid-thoracic region had a high prevalence of OVF and other degeneration changes, though for LFO the T10 level had the highest prevalence. For both OVF and SN, another peak appeared at T12 level.
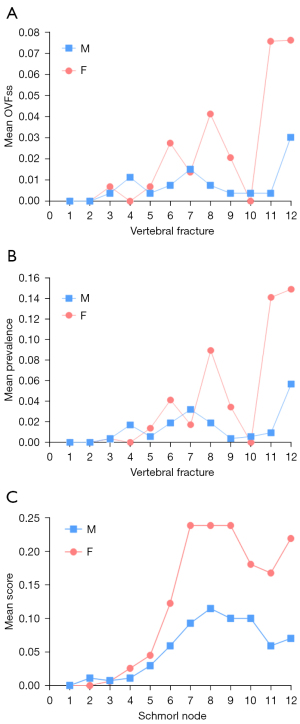
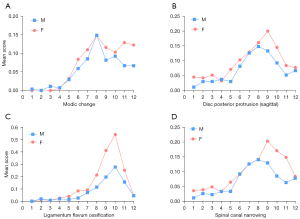
The correlation between age and five degeneration changes is shown in Table 3. The summed score of SNs was positively correlated with older age, both for men and for women. The same was true for canal narrowing, Modic change, and LFO. The correlation between age and disc protrusion summed score was not statistically significant. Among the five degeneration changes assessed, the correlation between SN and age was the strongest.
Table 3
Gender | Age vs. | ||||
---|---|---|---|---|---|
Schmorl’s node | Modic change | Disc protrusion | LFO | Canal narrowing | |
Men | |||||
Pearson r | 0.281* | 0.183* | 0.071 | 0.123* | 0.116# |
P value | <0.0001 | 0.0026 | 0.244 | 0.043 | 0.057 |
Women | |||||
Pearson r | 0.182* | 0.162* | 0.120 | 0.270* | 0.205* |
P value | 0.024 | 0.044 | 0.136 | <0.001 | 0.011 |
Men and women | |||||
Pearson r | 0.182* | 0.159* | 0.079 | 0.147* | 0.135* |
P value | 0.0002 | 0.001 | 0.108 | 0.002 | 0.006 |
*, correlation achieved statistical difference; #, correlation achieved marginal statistical difference. Men and women, results of the man and women grouped together; LFO, ligamentum flavum ossification.
Subjects with SN were more likely to have OVF, with odds ratio (OR) for men of 4.32 [95% confidence interval (CI): 1.70–11.00, P=0.002] and OR for women of 3.28 (95% CI: 1.23–8.74, P=0.018). If only OVFs with >1/3 vertebral height loss were counted, the OR for men increased to 5.69 (95% CI: 1.61–20.09, P<0.01). SN was statistically significantly correlated with lower femoral neck BMD derived T-score, while other four spine degeneration changes were not statistically significantly correlated with the T-score (Table 4). SN was also statistically significantly correlated OVFss. The five spine degeneration features were all mutually statistically significantly correlated with each other (Table 4).
Table 4
Assessed item | Schmorl’s node | Modic change | Disc protrusion | LFO | Canal narrowing |
---|---|---|---|---|---|
T score | −0.121 (0.015)* | P>0.1 | P>0.1 | P>0.1 | P>0.1 |
OVFss | 0.126 (0.011)* | P>0.1 | P>0.1 | 0.131 (0.0078)* | 0.093 (0.061)# |
Schmorl’s node | – | 0.304 (<0.0001)* | 0.344 (<0.0001)* | 0.226 (<0.0001)* | 0.366 (<0.0001)* |
Schmorl’s node | – | 0.283 (<0.0001)*§ | 0.336 (<0.0001)*§ | 0.204 (<0.0001)*§ | 0.350 (<0.0001)*§ |
Modic change | 0.304 (<0.0001)* | – | 0.480 (<0.0001)* | 0.299 (<0.0001)* | 0.469 (<0.0001) |
Modic change | 0.283 (<0.0001)*§ | – | 0.475(<0.0001)*§ | 0.282 (<0.0001)*§ | 0.457 (<0.0001)*§ |
Disc protrusion | 0.344 (<0.0001)* | 0.480 (<0.0001)* | – | 0.495 (<0.0001)* | 0.936 (<0.0001)* |
Disc protrusion | 0.336 (<0.0001)*§ | 0.475 (<0.0001)*§ | – | 0.490 (<0.0001)*§ | 0.937(<0.0001)*§ |
LFO | 0.226 (<0.0001)* | 0.299 (<0.0001) | 0.495 (<0.0001)* | – | 0.544 (<0.0001)* |
LFO | 0.204 (<0.0001)*§ | 0.282 (<0.0001)*§ | 0.490 (<0.0001)*§ | – | 0.535 (<0.0001)*§ |
Canal narrowing | 0.366 (<0.0001)* | 0.469 (<0.0001)* | 0.936 (<0.0001)* | 0.544 (<0.0001)* | – |
Canal narrowing | 0.350 (<0.0001)*§ | 0.457(<0.0001)*§ | 0.937(<0.0001)*§ | 0.535 (<0.0001)*§ | – |
Number in the cells are Pearson r value and statistically significant P value in the bracket, or only nonsignificant P. *, statistically significant; #, P<0.1, marginally significant; §, values were correlations after the adjustment for age. BMD, bone mineral density; LFO, ligamentum flavum ossification; OVF, osteoporotic vertebral fracture; OVFss, OVF sum score; T-score, femoral neck T-score.
Discussion
In this population-based study of older Chinese, using thoracic spine MR imaging we demonstrated that: (I) OVFss was statistically significantly correlated with SN. Subjects with SN were more likely to have OVF, with OR for men 4.32 (P=0.002) and for women 3.28 (P=0.018); (II) while all the five spine degeneration features were more prevalent in older women, the male-female difference was the greatest for SN; (III) compared with other four spine degeneration features, SN was most correlated with age; (IV) while the five spine degeneration features all tended to have a high prevalence in the mid-thoracic level, the vertebral level distribution of SN more resembled the vertebral level distribution of thoracic spine OVF, being high both at T8 and T12 levels (Figure 4) (29,30); (V) only SN was statistically significantly correlated with BMD derived femoral neck T-score, but not the other four spine degeneration features; (VI) all the five spine degeneration features were statistically significantly correlated with each other after the adjustment for age. Understandably, disc protrusion was very strongly correlated with spinal canal narrowing. Overall, these results suggest that, among the five spine changes evaluated in this study, SN was most correlated with OVF. This cross-section study results support the existence of this pathway that: osteoporosis → weakened endplate → SN development ↔ endplate fracture of osteoporotic vertebrae.
The correlations between SN and femoral neck T-score and OVFss in this study had a Pearson r of −0.121 (P=0.015) and 0.126 (P=0.011), respectively. Though these were not strong correlations, both P values reached the level of close to 0.01. The weak Pearson r value may also be due to there are multiple pathways for the genesis of OVF. Some OVFs may develop without pre-existing endplate SN. A common observation in osteoporosis studies is that many correlations have small correlation coefficient values. For example, with 200 MsOS (Hong Kong) female participants analysed in one recent study, we found that the correlation between OVFss (calculate from T4-L5) and femoral neck T-score had Pearson r of 0.33 (95% CI: 0.20–0.45, P<0.0001), and the correlation between OVFss and lumbar spine T-score had Pearson r of 0.15 (95% CI: 0.01–0.28, P=0.036, this was the reason we did not use lumbar spine T-score in this study). Another example is that Wong et al. (31) described strong risk factors can explained 30% variance of hip BMD, while for spine BMD, the associations were lower, only 20% variance can be explained by strong risk factors.
It has been noted that Caucasians have a higher prevalence of SN compared to other ethnicities like Africans (2). Dar et al. (32) studied 240 skeleton vertebrae (T4-L5) from a normal adult population, they noted that SN was more common in European-Americans (60.3%) than in African-Americans (36.7%). Moustarhfir et al. (23) studied 320 patients referred for spine MR imaging, and noted that SNs were significantly less frequent in patients with an African origin (OR =0.28; 95% CI: 0.17–0.48) compared to French Caucasian patients. It has been well recognised that populations of African ancestry have lower fragility fracture prevalence. In an analysis of National Health and Nutrition Examination Survey (NHANES) 2005–2008 data, Dawson-Hughes et al. (33) noted that spinal osteoporosis prevalence was 5.3% among non-Hispanic blacks and 10.9% among non-Hispanic whites. Tentative data also showed MRI detected SN prevalence is also lower among Asian populations compared with Caucasians (2). We have recently demonstrated that radiographic and clinical OVF prevalence among Hong Kong Chinese are approximately half of those of Caucasians (30,34). Therefore, the ethnic difference pattern of SN appears to mirror that of OVF.
Our study also contributes to the literature on epidemiology of SN. In a number of earlier several reports it was noted that, except for the early childhood period, SN prevalence among age groups remains stable (22,32,35,36). Dar et al. (32) studied 240 skeleton vertebrae (T4-L5) from a normal adult population, they noted that SN was gender dependent, more common in men than in women, but SNs were age independent. Because in their study of cadaveric thoracolumbar spines, they found no relationship between age and SNs, Hilton et al. (35) proposed a strictly developmental pathogenesis for SNs. Recently, Chen et al. (37) reported that aging was related to greater odds of endplate lesions; however, the prevalence of focal endplate defects remained stable. Sonne-Holm et al. (36) analysed lateral spine radiographs in an adult Caucasian population cohort. They did not observe any significant correlation between SNs and gender, age, and did not find any significant correlations between SNs and overall degeneration of the lumbar spine. On the other hand, some authors have reported the association of SN with age. Wang et al. (38) reported that greater age was associated with the presence of SN among a male cadaver collection. A male predominance of SN had been frequently reported (35,39-41). Üstündağ (41) studied SN in a post-medieval skeletal sample in Austria. It was noted that males were more affected than females, and there was no relationship found between SN and aging. However, a few studies did not observe an association of SN with gender (23,36,42). The correlation between osteoporosis and SNs also remains controversial (43,44). In a study on the cadavers of pre-Hispanic inhabitants González-Reimers et al. (43) did not find a relationship between osteoporosis and SN. On the other hand, other evidence suggests osteoporosis is a cause of SN. Mäkitie et al. (45) reported a high prevalence (61%) of SN in their case-control study of 18 patients diagnosed with WNT mutation-induced osteoporosis. Based on a CT study, Güngör et al. (46) suggested that low BMD may be a predisposing factor for the development of SN in patients younger than 40 years. We suspect the discordances of the above-listed reports were largely caused by different study subject sampling strategies. Results based on anthropological studies using skeleton samples suffer from ancient people did not live as long as we do in modern society and we in modern society suffer more from degenerative changes and less from traumas. In one our systematic review on lumbar spondylolisthesis (47), we noted that, among young and middle-aged subjects lumbar spondylolisthesis prevalence is low and mostly due to congenital or traumatic causes and also the prevalence is higher among men; on the other hand, in older subjects lumbar spondylolisthesis prevalence increases rapidly with aging and the degenerative cause dominates and also the prevalence is higher among post-menopausal women than age-matched men. The same may be true for SN. In young and middle-aged subjects SN is mostly due to congenital or traumatic causes (12-14) and the prevalence is higher among men and also SN is not correlated to osteoporosis. On the other hand, in older subjects SN is more associated with osteoporotic endplate weakening and their SN prevalence increases with greater age, and the prevalence is higher among women. This study also shows SN was correlated with other four types of spine degeneration changes, actually the five types of spine degeneration changes in this study were all correlated with each other. This corroborates with other reports that SNs are associated with degenerative changes of the spine (2,3,15,42,48).
There are many limitations to this study. The pathophysiology of SN formation is known to be multifactorial (2,3). In an ex vivo specimen study, Hansson and Roos (44) studied 109 lumbar vertebrae from 36 subjects. They reported that the bone mineral areal content was related to the number, shape, and localization of the SN detected in the X-rayed and sectioned vertebral bodies. One type of node, irregular in shape and localization, occurred only in the vertebrae with low bone mineral areal content values and thus in vertebrae with relatively low compressive strength. A second type of SN, regular in shape and localization, did not reflect any general weakness of the vertebral bodies. We did not try to sub-classify SN shape as this was not readily feasible with our in vivo MR images. We only acquired T2-weighted MR images, which also limited our ability to sub-classify Modic change. Due to the spatial resolution limitation of MR image and we did not simultaneously acquire computed tomography (CT) image, in some scenarios the differentiation between SN, Modic change, and endplate fracture may not always be distinct. Modic signal change could be occasionally from acute fractures of the endplate due to formation of an acute SN as this will cause edema in the region of bone around the endplate. For structural classification, ideally CT is used to evaluate SN and muti-contrast MR images are used to evaluation Modic changes. However, we expect this imperfection would not affect the overall conclusion of this study. Another issue is that, as we noted recently, fracture-shaped vertebral deformities, particularly those of milder grade, can also be seen among subjects of normal bone strength (49). However, statistically, fracture shaped vertebral deformities described in this study were more likely to be osteoporotic among older women, and fracture shaped vertebral deformities with ≥1/5 vertebral height loss were shown to be associated with increased further vertebral fracture risk statistically among older men (25,50). Considering that a portion of compressive vertebral deformities with ≥1/5 vertebral height loss could still be non-osteoporotic among men (49), compared with counting OVFs ≥1/5 vertebral height loss, in this study if only OVFs with ≥1/3 vertebral height loss were counted, then odds ratio increased from 4.32 to 5.69. Another limitation is that this study is a cross-sectional study and focused on thoracic spine. We could only observe correlation, but we could not study SN and OVF causation. We indeed acquired lumbar spine MRI for a portion of study participants both at baseline and at 14-year follow-up, thus we hope to conduct another longitudinal quantitative comparison to corroborate our results in this study. Finally, a few analyses may not be statistically well powered. This study was not initially planned to study SN, thus our study constitutes an ad hoc analysis. However, our study demonstrated statistically significant correlations between SN and lower femoral neck BMD derived T-score, and also between SN and OVFss.
In conclusion, our population-based MR imaging among older subjects shows SN in thoracic spine correlated with thoracic OVF prevalence, lower BMD, and other spinal degenerative changes. Our analysis suggests a number of features of SN parallel those of OVF. Our results support this pathway exists that: osteoporosis → weakened endplate → SN development ↔ osteoporotic endplate fracture. In addition, this study provides further insights into the epidemiology of SN.
Acknowledgments
Funding: None.
Footnote
Conflicts of Interest: All authors have completed the ICMJE uniform disclosure form (available at https://qims.amegroups.com/article/view/10.21037/qims-22-1410/coif). YXJW serves as the Editor-in-Chief of Quantitative Imaging in Medicine and Surgery. JFG serves as an unpaid editorial board member of Quantitative Imaging in Medicine and Surgery. The other authors have no conflicts of interest to declare.
Ethical Statement: The authors are accountable for all aspects of the work in ensuring that questions related to the accuracy or integrity of any part of the work are appropriately investigated and resolved. The study was conducted in accordance with the Declaration of Helsinki (as revised in 2013). The study protocol was approved by the Chinese University of Hong Kong Ethics Committee. Written informed consent was obtained from all subjects.
Open Access Statement: This is an Open Access article distributed in accordance with the Creative Commons Attribution-NonCommercial-NoDerivs 4.0 International License (CC BY-NC-ND 4.0), which permits the non-commercial replication and distribution of the article with the strict proviso that no changes or edits are made and the original work is properly cited (including links to both the formal publication through the relevant DOI and the license). See: https://creativecommons.org/licenses/by-nc-nd/4.0/.
References
- Schmorl G. Uber die an den wirbelbandscheiben vorkommenden ausdehnungs–und zerreisungsvorgange und die dadurch an ihnen und der wirbelspongiosa hervorgerufenen veranderungen. Verh Dtsch Path Ges 1927;22:250.
- Azzouzi H, Ichchou L. Schmorl's nodes: demystification road of endplate defects-a critical review. Spine Deform 2022;10:489-99. [Crossref] [PubMed]
- Kyere KA, Than KD, Wang AC, Rahman SU, Valdivia-Valdivia JM, La Marca F, Park P. Schmorl's nodes. Eur Spine J 2012;21:2115-21. [Crossref] [PubMed]
- Hamanishi C, Kawabata T, Yosii T, Tanaka S. Schmorl's nodes on magnetic resonance imaging. Their incidence and clinical relevance. Spine (Phila Pa 1976) 1994;19:450-3. [Crossref] [PubMed]
- Takahashi K, Miyazaki T, Ohnari H, Takino T, Tomita K. Schmorl's nodes and low-back pain. Analysis of magnetic resonance imaging findings in symptomatic and asymptomatic individuals. Eur Spine J 1995;4:56-9. [Crossref] [PubMed]
- Wang Y, Videman T, Battié MC. ISSLS prize winner: Lumbar vertebral endplate lesions: associations with disc degeneration and back pain history. Spine (Phila Pa 1976) 2012;37:1490-6. [Crossref] [PubMed]
- Wáng YXJ, Wu AM, Ruiz Santiago F, Nogueira-Barbosa MH. Informed appropriate imaging for low back pain management: A narrative review. J Orthop Translat 2018;15:21-34. [Crossref] [PubMed]
- Stäbler A, Bellan M, Weiss M, Gärtner C, Brossmann J, Reiser MF. MR imaging of enhancing intraosseous disk herniation (Schmorl's nodes). AJR Am J Roentgenol 1997;168:933-8. [Crossref] [PubMed]
- Dar G, Masharawi Y, Peleg S, Steinberg N, May H, Medlej B, Peled N, Hershkovitz I. Schmorl's nodes distribution in the human spine and its possible etiology. Eur Spine J 2010;19:670-5. [Crossref] [PubMed]
- Resnick D, Niwayama G. Intravertebral disk herniations: cartilaginous (Schmorl's) nodes. Radiology 1978;126:57-65. [Crossref] [PubMed]
- Chandraraj S, Briggs CA, Opeskin K. Disc herniations in the young and end-plate vascularity. Clin Anat 1998;11:171-6. [Crossref] [PubMed]
- Möller A, Maly P, Besjakov J, Hasserius R, Ohlin A, Karlsson MK. A vertebral fracture in childhood is not a risk factor for disc degeneration but for Schmorl's nodes: a mean 40-year observational study. Spine (Phila Pa 1976) 2007;32:2487-92. [Crossref] [PubMed]
- Fahey V, Opeskin K, Silberstein M, Anderson R, Briggs C. The pathogenesis of Schmorl's nodes in relation to acute trauma. An autopsy study. Spine (Phila Pa 1976) 1998;23:2272-5. [Crossref] [PubMed]
- Swärd L, Hellström M, Jacobsson B, Nyman R, Peterson L. Disc degeneration and associated abnormalities of the spine in elite gymnasts. A magnetic resonance imaging study. Spine (Phila Pa 1976) 1991;16:437-43. [Crossref] [PubMed]
- Williams FM, Manek NJ, Sambrook PN, Spector TD, Macgregor AJ. Schmorl's nodes: common, highly heritable, and related to lumbar disc disease. Arthritis Rheum 2007;57:855-60. [Crossref] [PubMed]
- Rajasekaran S, Kanna RM, Reddy RR, Natesan S, Raveendran M, Cheung KMC, Chan D, Kao PYP, Yee A, Shetty AP. How Reliable Are the Reported Genetic Associations in Disc Degeneration?: The Influence of Phenotypes, Age, Population Size, and Inclusion Sequence in 809 Patients. Spine (Phila Pa 1976) 2016;41:1649-60. [Crossref] [PubMed]
- Jiang G, Eastell R, Barrington NA, Ferrar L. Comparison of methods for the visual identification of prevalent vertebral fracture in osteoporosis. Osteoporos Int 2004;15:887-96. [Crossref] [PubMed]
- Wáng YXJ, Che-Nordin N, Deng M, Leung JCS, Kwok AWL, He LC, Griffith JF, Kwok TCY, Leung PC. Osteoporotic vertebral deformity with endplate/cortex fracture is associated with higher further vertebral fracture risk: the Ms. OS (Hong Kong) study results. Osteoporos Int 2019;30:897-905. [Crossref] [PubMed]
- Lentle BC, Berger C, Probyn L, Brown JP, Langsetmo L, Fine B, Lian K, Shergill AK, Trollip J, Jackson S, Leslie WD, Prior JC, Kaiser SM, Hanley DA, Adachi JD, Towheed T, Davison KS, Cheung AM, Goltzman D. Comparative Analysis of the Radiology of Osteoporotic Vertebral Fractures in Women and Men: Cross-Sectional and Longitudinal Observations from the Canadian Multicentre Osteoporosis Study (CaMos). J Bone Miner Res 2018;33:569-79. [Crossref] [PubMed]
- Wang YX, Griffith JF. Menopause causes vertebral endplate degeneration and decrease in nutrient diffusion to the intervertebral discs. Med Hypotheses 2011;77:18-20. [Crossref] [PubMed]
- Pfirrmann CW, Resnick D. Schmorl nodes of the thoracic and lumbar spine: radiographic-pathologic study of prevalence, characterization, and correlation with degenerative changes of 1,650 spinal levels in 100 cadavers. Radiology 2001;219:368-74. [Crossref] [PubMed]
- Mok FP, Samartzis D, Karppinen J, Luk KD, Fong DY, Cheung KM. ISSLS prize winner: prevalence, determinants, and association of Schmorl nodes of the lumbar spine with disc degeneration: a population-based study of 2449 individuals. Spine (Phila Pa 1976) 2010;35:1944-52. [Crossref] [PubMed]
- Moustarhfir M, Bresson B, Koch P, Perozziello A, Barreau G, Schouman-Claeys E, Henry-Feugeas MC, Ou P, Dallaudière B. MR imaging of Schmorl's nodes: Imaging characteristics and epidemio-clinical relationships. Diagn Interv Imaging 2016;97:411-7. [Crossref] [PubMed]
- Wang XR, Kwok TCY, Griffith JF, Man Yu BW, Leung JCS, Wáng YXJ. Prevalence of cervical spine degenerative changes in elderly population and its weak association with aging, neck pain, and osteoporosis. Ann Transl Med 2019;7:486. [Crossref] [PubMed]
- Wáng YXJ, Che-Nordin N, Leung JCS, Man Yu BW, Griffith JF, Kwok TCY. Elderly men have much lower vertebral fracture risk than elderly women even at advanced age: the MrOS and MsOS (Hong Kong) year 14 follow-up radiology results. Arch Osteoporos 2020;15:176. [Crossref] [PubMed]
- Wáng YXJ, Diacinti D, Yu W, Cheng XG, Nogueira-Barbosa MH, Che-Nordin N, Guglielmi G, Ruiz Santiago F. Semi-quantitative grading and extended semi-quantitative grading for osteoporotic vertebral deformity: a radiographic image database for education and calibration. Ann Transl Med 2020;8:398. [Crossref] [PubMed]
- Wáng YXJ, Santiago FR, Deng M, Nogueira-Barbosa MH. Identifying osteoporotic vertebral endplate and cortex fractures. Quant Imaging Med Surg 2017;7:555-91. [Crossref] [PubMed]
- Wáng YXJ. An update of our understanding of radiographic diagnostics for prevalent osteoporotic vertebral fracture in elderly women. Quant Imaging Med Surg 2022;12:3495-514. [Crossref] [PubMed]
- Wáng YXJ, Lentle BC. Radiographic osteoporotic vertebral fractures in elderly men: a brief review focusing on differences between the sexes. Quant Imaging Med Surg 2020;10:1863-76. [Crossref] [PubMed]
- Wáng YXJ, Diacinti D, Leung JCS, Iannacone A, Kripa E, Kwok TCY, Diacinti D. Much lower prevalence and severity of radiographic osteoporotic vertebral fracture in elderly Hong Kong Chinese women than in age-matched Rome Caucasian women: a cross-sectional study. Arch Osteoporos 2021;16:174. [Crossref] [PubMed]
- Wong SY, Kwok T, Woo J, Lynn H, Griffith JF, Leung J, Tang YY, Leung PC. Bone mineral density and the risk of peripheral arterial disease in men and women: results from Mr. and Ms Os, Hong Kong. Osteoporos Int 2005;16:1933-8. [Crossref] [PubMed]
- Dar G, Peleg S, Masharawi Y, Steinberg N, May H, Hershkovitz I. Demographical aspects of Schmorl nodes: a skeletal study. Spine (Phila Pa 1976) 2009;34:E312-5. [Crossref] [PubMed]
- Dawson-Hughes B, Looker AC, Tosteson AN, Johansson H, Kanis JA, Melton LJ 3rd. The potential impact of the National Osteoporosis Foundation guidance on treatment eligibility in the USA: an update in NHANES 2005-2008. Osteoporos Int 2012;23:811-20. [Crossref] [PubMed]
- Wáng YXJ, Deng M, Griffith JF, Kwok AWL, Leung JCS, Lam PMS, Yu BWM, Leung PC, Kwok TCY. 'Healthier Chinese spine': an update of osteoporotic fractures in men (MrOS) and in women (MsOS) Hong Kong spine radiograph studies. Quant Imaging Med Surg 2022;12:2090-105. [Crossref] [PubMed]
- Hilton RC, Ball J, Benn RT. Vertebral end-plate lesions (Schmorl's nodes) in the dorsolumbar spine. Ann Rheum Dis 1976;35:127-32. [Crossref] [PubMed]
- Sonne-Holm S, Jacobsen S, Rovsing H, Monrad H. The epidemiology of Schmorl's nodes and their correlation to radiographic degeneration in 4,151 subjects. Eur Spine J 2013;22:1907-12. [Crossref] [PubMed]
- Chen L, Battié MC, Yuan Y, Yang G, Chen Z, Wang Y. Lumbar vertebral endplate defects on magnetic resonance images: prevalence, distribution patterns, and associations with back pain. Spine J 2020;20:352-60. [Crossref] [PubMed]
- Wang Y, Videman T, Battié MC. Lumbar vertebral endplate lesions: prevalence, classification, and association with age. Spine (Phila Pa 1976) 2012;37:1432-9. [Crossref] [PubMed]
- Yin R, Lord EL, Cohen JR, Buser Z, Lao L, Zhong G, Wang JC. Distribution of Schmorl nodes in the lumbar spine and their relationship with lumbar disk degeneration and range of motion. Spine (Phila Pa 1976) 2015;40:E49-53. [Crossref] [PubMed]
- Brayda-Bruno M, Albano D, Cannella G, Galbusera F, Zerbi A. Endplate lesions in the lumbar spine: a novel MRI-based classification scheme and epidemiology in low back pain patients. Eur Spine J 2018;27:2854-61. [Crossref] [PubMed]
- Üstündağ H. Schmorl’s Nodes in a Post-Medieval Skeletal Sample from Klostermarienberg, Austria. Int J Osteoarchaeol 2009;19:695-710. [Crossref]
- Zehra U, Cheung JPY, Bow C, Lu W, Samartzis D. Multidimensional vertebral endplate defects are associated with disc degeneration, modic changes, facet joint abnormalities, and pain. J Orthop Res 2019;37:1080-9. [Crossref] [PubMed]
- González-Reimers E, Mas-Pascual M, Arnay-De-La-Rosa M, Velasco-Vázquez J, Santolaria-Fernández F. Schmorl nodes: lack of relationship between degenerative changes and osteopenia. Radiology 2002;222:293-4. [Crossref] [PubMed]
- Hansson T, Roos B. The amount of bone mineral and Schmorl's nodes in lumbar vertebrae. Spine (Phila Pa 1976) 1983;8:266-71. [Crossref] [PubMed]
- Mäkitie RE, Niinimäki T, Nieminen MT, Schalin-Jäntti C, Niinimäki J, Mäkitie O. Impaired WNT signaling and the spine-Heterozygous WNT1 mutation causes severe age-related spinal pathology. Bone 2017;101:3-9. [Crossref] [PubMed]
- Güngör Ö, Gezer NS, Özdamarlar U, Balcı A. The effect of bone mineral density on development of Schmorl's nodes in young patients. Acta Orthop Traumatol Turc 2020;54:287-92. [Crossref] [PubMed]
- Wang YXJ, Káplár Z, Deng M, Leung JCS. Lumbar degenerative spondylolisthesis epidemiology: A systematic review with a focus on gender-specific and age-specific prevalence. J Orthop Translat 2016;11:39-52. [Crossref] [PubMed]
- Fields AJ, Liebenberg EC, Lotz JC. Innervation of pathologies in the lumbar vertebral end plate and intervertebral disc. Spine J 2014;14:513-21. [Crossref] [PubMed]
- Ma JB, Wáng YXJ. Chest radiograph prevalence of vertebral deformity among young and middle-aged population of mixed city dwellers and rural residents. J Thorac Dis 2022;14:4685-98. [Crossref] [PubMed]
- Wáng YXJ, Lu ZH, Leung JCS, Fang ZY, Kwok TCY. Osteoporotic-like vertebral fracture with less than 20% height loss is associated with increased further vertebral fracture risk in older women: the MrOS and MsOS (Hong Kong) year-18 follow-up radiograph results. Quant Imaging Med Surg 2022; [Crossref]