Increased tissue water in patients with severe sepsis affects tissue oxygenation measured by near-infrared spectroscopy: a prospective, observational case-control study
Introduction
Severe sepsis is a life-threatening disease characterised by severe systemic inflammation, unstable haemodynamics, and multiple organ failure (1,2). Current treatment guidelines recommend fluid resuscitation to expand the intravascular volume and the use of vasopressors to achieve a target systemic arterial pressure level for the normalisation of unstable haemodynamics (3,4). However, excessive fluid administration, leading to tissue oedema and deterioration of tissue perfusion, remains a major concern (5). In addition, due to microcirculatory alterations in the presence of severe sepsis, loss of coherence between the macrocirculation and microcirculation occurs (6). Therefore, achieving the recommended systemic arterial pressure target may not guarantee an improvement in peripheral tissue perfusion and oxygenation (6-9). Sepsis with normal blood pressure may still show abnormal microcirculation (10,11). The primary function of the circulatory system is to deliver nutrients and oxygen to the organs and tissues and remove waste products. The ultimate goal of treating sepsis-related circulatory dysfunction is to restore microcirculation to provide adequate tissue perfusion and oxygenation to prevent eventual multiple organ failure (12,13). Therefore, monitoring tissue perfusion and oxygenation is essential for managing sepsis-related circulatory dysfunction.
Near-infrared spectroscopy (NIRS), a non-invasive and indirect method, has been developed and applied to measure tissue oxygenation (14). NIRS can detect the tissue content of haemoglobin (Hb) and myoglobin (Mb) in different oxygenation states (15). The estimation of tissue Hb oxygen saturation (StO2) is based on the differential absorption properties of oxy-Hb (HbO2) and deoxy-Hb (HbR) under different spectra of near-infrared wavelength light (16). In limb skeletal muscle at rest, light absorption is mainly from Mb, which contributes 50% to 70% of the total light absorption potential for NIRS in skeletal muscle (17). However, Hb rather than Mb can be altered by limb perfusion (18). In the presence of sepsis, the baseline thenar StO2 is low and changes in StO2 recovery after an ischaemic challenge are related to the survival of patients in the intensive care unit (ICU) (19). Low StO2 in early resuscitation is associated with poor outcomes and high mortality rates (20,21). However, sepsis-related tissue oedema caused by endothelial dysfunction and vascular leak has been determined to have a confounding effect on the assessment of StO2 with NIRS (22,23). To date, the impact of tissue water (H2O) on tissue HbO2 and HbR has not been comprehensively investigated.
In our previous study, we used NIRS to detect tissue Hb and H2O in the extremities of children with Kawasaki disease. We found that tissue H2O was significantly higher, but tissue Hb was lower in patients with Kawasaki disease than in healthy controls (24). In the present study, we applied NIRS to simultaneously detect and quantify regional tissue Hb and H2O content in adult patients with severe sepsis and healthy volunteers. In addition, we compared the differences in regional tissue oxygenation and H2O content between patients and healthy controls, as well as evaluated the relationships among systemic arterial pressure, regional tissue oxygenation, and H2O content in patients with severe sepsis. We present the following article in accordance with the STROBE reporting checklist (available at https://qims.amegroups.com/article/view/10.21037/qims-22-127/rc).
Methods
Near-infrared diffuse optical technique and wireless optical monitoring system
The fundamental principle of the near-infrared diffuse optical technique is based on the unique absorption and scattering properties of different tissue components corresponding to different wavelengths of light (15,25-29) For red and near-infrared wavelength light (range, 600–1,200 nm), HbO2, HbR, Mb and H2O are the major absorbers in human tissue. Under the assumption that the Mb concentration during the short term is constant, we only monitor the change of HbO2, HbR, and H2O in this study. Based on the apparent difference in the absorption spectra of HbO2, HbR, and H2O corresponding to different wavelengths, we used a tri-wavelength light (700, 910, and 950 nm)-emitting diode to assemble a wireless optical monitoring system comprising an optical probe, a wireless signal acquisition module, and a back-end host system (Figure 1). The optical probe was positioned on the volar surface of the right leg of the patients and healthy volunteers, at the level of the anterior tibial muscle, to estimate the relative tissue concentrations of HbO2 ([HbO2]), HbR ([HbR]), total Hb ([HbT]), and H2O ([H2O]) from the change in the optical density corresponding to different wavelengths. For the wavelength λ, the optical density attenuation of red or near-infrared light in human tissue can be simply expressed as
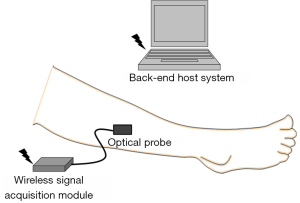
where d is the distance between the light source and detector, B(λ) is the differential path-length factor, and εHbO2(λ), εHbR(λ), and εH2O(λ) denote the molar extinction coefficient of [HbO2], [HbR], and [H2O]. Then, [HbO2], [HbR], and [H2O] can be estimated by the approach of least-squares approximation, as followings,
StO2 was calculated as
Study design and participants
This prospective, observational case-control study was approved by the Institutional Review Board of Chang Gung Medical Foundation (approval No. 103-5357B). The study was conducted in accordance with the Declaration of Helsinki (as revised in 2013) and was conducted in a 20-bed medical ICU of Chiayi Chang Gung Memorial Hospital from 27 November 2015 to 30 April 2019. Adult patients (age ≥18 years) who were transferred to the ICU from the emergency department and admitted within 72 h for new-onset severe sepsis were enrolled. Severe sepsis was defined as sepsis with sepsis-induced tissue hypoperfusion or organ dysfunction according to the 2012 Surviving Sepsis Campaign criteria (30). Patients with pregnancy, active pulmonary tuberculosis, or contact isolation were excluded from the study. Healthy volunteers who did not have any disorders were recruited among the students of the National Chiao Tung University as healthy controls. Informed consent was obtained from the healthy volunteers and patients. Legal guardians provided informed consent to participate if the patient had cognitive impairment. After signing the informed consent form, the patients and healthy volunteers received a non-invasive NIRS device for use in estimations at rest. The estimated data were recorded after 3 min to stabilise the NIRS signals (31). The NIRS data were collected for 3 consecutive days or until the patient was transferred to an ordinary ward, discharged against medical advice, or died. We recorded the demographic and clinical data of all patients, including age, sex, aetiology of severe sepsis, acute physiology and chronic health evaluation II score on admission, systemic haemodynamic parameters, laboratory results, and ICU outcome. Patients with missing or incomplete NIRS data were excluded from the final analysis.
Sample size calculation
The sample size estimate for the study was based on a comparison of repeated measures between patients and controls. Since this study was a pilot study and there was no previous applicable research to calculate the effect size, we considered a power of 0.95 at the 0.05 alpha level and an estimated effect size of 0.15 to calculate the sample size. Using G*Power, a sample size of 70 per group was required. However, that was inflated by 20% for any potentially missing data, thus yielding a priori sample size of 84 per group.
Statistical analyses
Continuous data were summarised as mean, standard deviation (SD), and 95% confidence interval (CI) or median and interquartile range (IQR), as appropriate. Categorical data were expressed using counts and percentages. A two-sample t-test was used to compare the differences between cases and controls for continuous variables once normality was demonstrated; otherwise, the nonparametric Mann-Whitney U test was performed. The chi-square or Fisher’s exact test was performed for categorical variables. Pearson correlation coefficients were used to investigate pairwise relationships between continuous variables. As the relative tissue concentrations of Hb and H2O and tissue oxygenation were repeatedly measured at designated intervals over time, generalised estimating equations (GEEs), which consider the correlation within individuals, were employed to evaluate the differences in NIRS parameters between groups. Statistical analyses were performed using SPSS version 22 (IBM Corp., Armonk, NY, USA). All tests were two-tailed with a significance level of 0.05.
Results
Demographic characteristics and clinical data of patients with severe sepsis
A total of 203 consecutive patients were assessed for eligibility (Figure 2). Six patients were excluded because of active pulmonary tuberculosis or requiring contact isolation, and 113 patients declined to participate in the study. The remaining 84 patients received the NIRS device for estimating regional tissue oxygenation and H2O content. In seven patients, the wireless signals of the device were intermittently received by the back-end host system, and the data were incompletely recorded. Therefore, the integrated data of the remaining 77 patients were analysed. Of these 77 patients, 30 were women and 47 were men (Table 1). The median [IQR] age of the patients was 75 [65–83] years. In addition, 45 patients (58%) had septic shock and received vasoactive agent therapy. In the three consecutive days, the total mean (SD) of the patient’s net intake and output before the different NIRS measurements was 514.86 (960.87) mL per day, and the total mean (SD) of the amount of fluid administered to patients before the different NIRS measurements was 1,376.16 (823.84) mL per day. Furthermore, 51 patients (66%) had pulmonary infection, and 61 patients (79%) were ICU survivors. The median (IQR) length of ICU stay was 7.00 (4.00–11.50) days. There were a total of 30 healthy controls recruited for the study (Figure 2). Among the healthy controls, 20 (67%) were men with a median [IQR] age of 24 [23–25] years, which was significantly lower than the age of patients with sepsis (P<0.001) (Table 1).
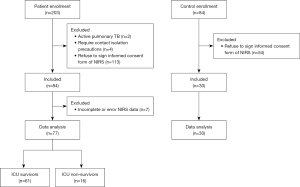
Table 1
Variables | Controls (n=30) | Patients (n=77) | P value |
---|---|---|---|
Age (years), median [IQR] | 24 [23–25] | 75 [65–83] | <0.001 |
Sex, male, n (%) | 20 (67%) | 47 (61%) | 0.589 |
Body height (cm), mean (SD) | 170.53 (8.11) | 160.19 (7.73) | <0.001 |
Body weight (kg), median (IQR) | 68.15 (56.58–78.70) | 58.00 (49.50–67.00) | 0.001 |
Body mass index (kg/m2), median (IQR) | 22.43 (20.06–26.96) | 22.49 (19.52–24.99) | 0.528 |
Septic shock with using vasoactive agents, n (%) | – | 45 (58%) | – |
Glasgow coma scale, median [IQR] | – | 8 [6–15] | – |
Acute Physiology and Chronic Health Evaluation Score II, mean (SD) | – | 18.91 (6.60) | – |
Mean arterial pressure (mmHg), median (IQR) | – | 87.50 (78.00–95.00) | – |
Systolic arterial pressure (mmHg), mean (SD) | – | 114.75 (19.58) | – |
Diastolic arterial pressure (mmHg), median (IQR) | – | 59.00 (53.50–70.50) | – |
White blood cells (1,000/μL), mean (SD) | – | 13.64 (8.56) | – |
Haemoglobin (g/dL), mean (SD) | – | 11.19 (2.65) | – |
Creatinine (mg/dL), median (IQR) | – | 1.59 (0.98–2.45) | – |
Arterial lactate (mg/dL) (n=75)*, median (IQR) | – | 19.40 (13.60–31.40) | – |
Partial pressure of oxygen (mmHg), median (IQR) | – | 105.80 (83.75–143.55) | – |
Arterial oxygen saturation (%), median (IQR) | – | 98.10 (96.95–99.35) | – |
Intake and output, mL/day** | |||
Day 1 (n=70)*, median (IQR) | 201.00 (−262.25 to 1,152.75) | ||
Day 2 (n=77), median (IQR) | 531 (−132 to 1,380) | ||
Day 3 (n=75)*, mean (SD) | 337.69 (1,222.80) | ||
Total mean, mean (SD) | 514.86 (960.87) | ||
Intravascular fluid administration, mL/day** | |||
Day 1 (n=70)*, median (IQR) | 973.00 (590.00–1,588.75) | ||
Day 2 (n=77), median (IQR) | 1,414.00 (902.00–1,984.50) | ||
Day 3 (n=75)*, median [IQR] | 988 [480–1,693] | ||
Total mean, mean (SD) | 1,376.16 (823.84) | ||
Diagnosis, n (%) | |||
Pulmonary infection | 51 (66%) | ||
Urinary tract infection | 36 (47%) | ||
Hepatic or biliary tract infection | 8 (10%) | ||
Spontaneous bacteria peritonitis | 1 (1%) | ||
Pelvic infection | 1 (1%) | ||
Cellulitis | 4 (5%) | ||
Other | 3 (4%) | ||
ICU survivor, n (%) | – | 61 (79%) | – |
ICU length of stay, days, median (IQR) | – | 7.00 (4.00–11.50) | – |
*, variable with missing or unrecorded data; **, data recorded on the day before the NIRS measurement. n, count; IQR, interquartile range; SD, standard deviation; ICU, intensive care unit; NIRS, near-infrared spectroscopy.
Comparisons of relative tissue concentrations of haemoglobin and water and tissue oxygenation between healthy controls and patients with severe sepsis
In patients with severe sepsis, the first-day measurement of anterior tibial muscle [H2O] was higher [mean (SD, 95% CI), 10.57 (3.37, 9.81–11.34) vs. 7.40 (1.89, 6.70–8.11); Table 2] and the [HbO2], [HbT], and StO2 were lower [0.20 (0.01, 0.20–0.20) vs. 0.22 (0.01, 0.22–0.23), 0.42 (0.02, 0.42–0.43) vs. 0.44 (0.02, 0.44–0.45), and 47.25% (1.97%, 46.80–47.70%) vs. 49.88% (1.26%, 49.41–50.35%), respectively; Table 2] than in healthy controls. In the next two consecutive days, the repeated measurements of [H2O] were higher, whereas [HbO2], [HbT], and StO2 remained lower in patients than in healthy controls (Figure 3). The GEE analysis also showed that these four parameters were significantly different between the two groups (all P≤0.001, Figure 3).
Table 2
Measurements | Days | Controls | Patients | |||||
---|---|---|---|---|---|---|---|---|
Mean (SD) | 95% CI | n | Mean (SD) | 95% CI | n | |||
[HbO2] (a.u.) | 1 | 0.22 (0.01) | 0.22–0.23 | 30 | 0.20 (0.01) | 0.20–0.20 | 77 | |
2 | 0.22 (0.01) | 0.22–0.23 | 30 | 0.20 (0.02) | 0.19–0.20 | 75 | ||
3 | 0.22 (0.01) | 0.22–0.23 | 30 | 0.19 (0.03) | 0.19–0.20 | 64 | ||
[HbR] (a.u.) | 1 | 0.22 (0.02) | 0.22–0.23 | 30 | 0.22 (0.02) | 0.22–0.23 | 77 | |
2 | 0.22 (0.02) | 0.22–0.23 | 30 | 0.23 (0.03) | 0.22–0.24 | 75 | ||
3 | 0.22 (0.02) | 0.22–0.23 | 30 | 0.23 (0.04) | 0.22–0.24 | 64 | ||
[HbT] (a.u.) | 1 | 0.44 (0.02) | 0.44–0.45 | 30 | 0.42 (0.02) | 0.42–0.43 | 77 | |
2 | 0.44 (0.02) | 0.44–0.45 | 30 | 0.42 (0.02) | 0.42–0.43 | 75 | ||
3 | 0.44 (0.02) | 0.43–0.45 | 30 | 0.42 (0.03) | 0.42–0.43 | 64 | ||
StO2 (%) | 1 | 49.88 (1.26) | 49.41–50.35 | 30 | 47.25 (1.97) | 46.80–47.70 | 77 | |
2 | 49.97 (1.35) | 49.47–50.47 | 30 | 46.40 (5.25) | 45.19–47.61 | 75 | ||
3 | 49.92 (1.28) | 49.45–50.40 | 30 | 45.66 (6.80) | 43.96–47.35 | 64 | ||
[H2O] (a.u.) | 1 | 7.40 (1.89) | 6.70–8.11 | 30 | 10.57 (3.37) | 9.81–11.34 | 77 | |
2 | 7.60 (1.95) | 6.87–8.33 | 30 | 10.52 (3.40) | 9.74–11.30 | 75 | ||
3 | 7.49 (1.92) | 6.77–8.21 | 30 | 10.79 (3.40) | 9.94–11.63 | 64 |
a.u., arbitrary unit; SD, standard deviation; CI, confidence interval; n, count; [HbO2], relative tissue concentration of oxy-haemoglobin; [HbR], relative tissue concentration of deoxy-haemoglobin; [HbT], relative tissue concentration of total haemoglobin; StO2, tissue haemoglobin oxygen saturation; [H2O], relative tissue concentration of H2O.
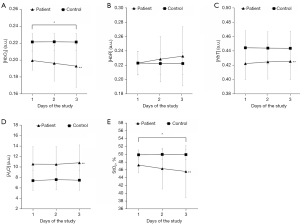
However, in terms of [HbR], the patients had the same value as the healthy controls [mean (SD, 95% CI), 0.22 (0.02, 0.22–0.23) vs. 0.22 (0.02, 0.22–0.23), in first-day measurements; Table 2], and the GEE analysis revealed that there was no difference among the 3-day repeated measurements between the groups (P=0.996, 0.248, and 0.121 on days 1, 2, and 3, respectively; see https://cdn.amegroups.cn/static/public/qims-22-127-1.docx). Furthermore, concerning the changes in the parameters over time, [HbO2] and StO2 gradually decreased in patients with severe sepsis (day 1 vs. day 3, P=0.041 and P=0.036, respectively; Figure 3). However, there were no changes in the time trends of tissue H2O content in either group or tissue oxygenation in healthy controls.
Relationship between tissue oxygenation and water content in severe sepsis
With respect to the relationship between tissue oxygenation and H2O content, negative correlations were found between [H2O] and [HbO2] (r=−0.51, −0.53, and −0.67 on days 1, 2, and 3, respectively, P<0.01 for all; Table 3) and StO2 (r=−0.42, −0.43, and −0.63 on days 1, 2, and 3, respectively, P<0.01, respectively; Table 3); however, [H2O] and [HbR] were positively correlated (r=0.28 on day 2 and 0.54 on day 3, P<0.05 and P<0.01, respectively; Table 3). The correlations between [H2O] and [HbO2], [HbR], and StO2 gradually became stronger during the 3-day repeated measurements (Figure 4A-4C). Furthermore, the correlation between [HbT] and StO2 was negative (r=−0.29 on day 1, P<0.05; r=−0.46 and −0.65 on days 2 and 3, respectively, P<0.01 for both; Table 3 and Figure 4D). Meanwhile, the relationship between [HbO2] and [HbR] changed over time: positive on day 1 (r=0.30, P<0.01; Table 3) but negative on days 2 and 3 (r=−0.65, P<0.01, and −0.81, P<0.01, respectively; Table 3 and Figure 4E).
Table 3
Measurements | Days | [H2O] | [HbO2] | [HbR] | [HbT] |
---|---|---|---|---|---|
[HbO2] | 1 | −0.51** | – | – | – |
2 | −0.53** | – | – | – | |
3 | −0.67** | – | – | – | |
[HbR] | 1 | 0.04 | 0.30** | – | – |
2 | 0.28* | −0.65** | – | – | |
3 | 0.54** | −0.81** | – | – | |
[HbT] | 1 | −0.22 | 0.72** | 0.88** | – |
2 | −0.10 | 0.02 | 0.75** | – | |
3 | 0.21 | −0.33** | 0.82** | – | |
StO2 | 1 | −0.42** | 0.45** | −0.71** | −0.29* |
2 | −0.43** | 0.88** | −0.93** | −0.46** | |
3 | −0.63** | 0.93** | −0.97** | −0.65** |
Numbers in cells are Pearson’s correlation coefficients. **P<0.01; *P<0.05. The actual P values have been included in the https://cdn.amegroups.cn/static/public/qims-22-127-1.docx. [HbO2], relative tissue concentration of oxy-haemoglobin; [HbR], relative tissue concentration of deoxy-haemoglobin; [HbT], relative tissue concentration of total haemoglobin; StO2, tissue haemoglobin oxygen saturation; [H2O], relative tissue concentration of H2O.
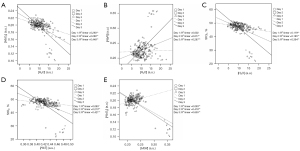
Association of regional tissue oxygenation and water content with systemic arterial pressure in severe sepsis
With regard to the associations of systemic haemodynamics with regional tissue oxygenation and H2O content in patients with severe sepsis, we analysed the correlations between real-time arterial blood pressures and NIRS parameters in patients who also received pulse contour cardiac output (PiCCO) monitoring. A total of 21 patients were monitored using the PiCCO. One of them was monitored starting from day 2, and monitoring was stopped for six patients on day 3 after participating in the study. We found that only [H2O] was significantly positively correlated with systemic arterial pressure in the first-day measurement, and the correlation coefficients with systolic arterial pressure and mean arterial pressure (MAP) were 0.51 (P<0.05, Table 4 and Figure 5) and 0.45 (P<0.05, Table 4 and Figure 5), respectively. However, no significant correlation was found between tissue oxygenation and systemic arterial pressure.
Table 4
Measurements | Days | [HbO2] | [HbR] | [HbT] | StO2 | [H2O] |
---|---|---|---|---|---|---|
Systolic arterial pressure | 1 | −0.15 | 0.23 | 0.11 | −0.30 | 0.51* |
2 | −0.39 | −0.19 | −0.29 | −0.06 | 0.36 | |
3 | −0.11 | 0.18 | 0.18 | −0.15 | 0.09 | |
Diastolic arterial pressure | 1 | 0.09 | 0.25 | 0.24 | −0.16 | 0.39 |
2 | −0.25 | −0.13 | −0.20 | <−0.01 | 0.20 | |
3 | −0.37 | 0.33 | 0.22 | −0.35 | 0.29 | |
Mean arterial pressure | 1 | −0.06 | 0.19 | 0.13 | −0.20 | 0.45* |
2 | −0.35 | −0.15 | −0.25 | −0.06 | 0.31 | |
3 | −0.26 | 0.30 | 0.25 | −0.29 | 0.22 |
Numbers in cells are Pearson’s correlation coefficients. *P<0.05. The actual P values have been included in the https://cdn.amegroups.cn/static/public/qims-22-127-1.docx. [HbO2], relative tissue concentration of oxy-haemoglobin; [HbR], relative tissue concentration of deoxy-haemoglobin; [HbT], relative tissue concentration of total haemoglobin; StO2, tissue haemoglobin oxygen saturation; [H2O], relative tissue concentration of H2O.
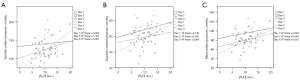
Discussion
Fluid administration in a fluid-responsive patient with sepsis can increase the left heart filling pressure and cardiac output, promote tissue perfusion and oxygenation, preserve organ function, and provide a survival benefit (32-34). However, the infused fluid is exchanged between the plasma and interstitium, and a large amount of fluid leaks from the capillaries and eventually accumulates in the interstitium (35). Increased vascular permeability in sepsis increases plasma volume loss, which becomes more pronounced when vasopressors are used to raise blood pressure (36,37). Therefore, the infusion may transiently affect haemodynamics but eventually cause tissue oedema and worsen tissue perfusion (38). Microcirculation improved by fluid administration was only observed in patients with severe sepsis diagnosed within 24 h (39). Liberal fluid administration can cause organ dysfunction and increase mortality risk (5,40,41). The current study used NIRS to detect tissue H2O and found that [H2O] was significantly higher in patients with severe sepsis than in healthy controls. Estimating [H2O] using NIRS may offer a real-time and non-invasive quantitative assessment of tissue oedema. This parameter is potentially valuable for guiding fluid therapy in patients with severe sepsis. Further studies are needed to ascertain the relationship between fluid administration and [H2O].
StO2 is a surrogate measure for assessing microcirculation (14,42). The value of StO2 varies depending on the measurement site (43,44). The normal gastrocnemius muscle StO2 is 65%±19% (45). Our study found that the normal anterior tibial muscle StO2 was 49.88%±1.26%. Muscle StO2 changes during limb perfusion (18). Lower venous oxygen saturation and StO2 have been identified in sepsis-related hypoperfusion (19,46). StO2 is dependent on [HbO2] and [HbR]. [HbO2], [HbT], and StO2 were significantly lower in patients with severe sepsis than in healthy controls. However, the [HbR] values were similar. In an early study, Davis and Barstow found that during exercise, changes in total tissue Hb and Mb measured by NIRS can reflect changes in microvascular hematocrit (17). In addition, NIRS-derived total Hb can indicate changes in muscular blood flow during exercise, measured using Doppler ultrasound (47). Therefore, [HbT] in the present study could be associated with tissue blood flow at rest. Besides, changes in HbR reflect microvascular oxygen extraction (48). Therefore, [HbR] may be related to tissue oxygen consumption (VO2) at rest. However, this does not mean that [HbR] can be a surrogate measure of VO2. Therefore, our findings suggest that patients with severe sepsis had reduced limb perfusion, resulting in lower [HbT]. Nevertheless, their peripheral skeletal muscle VO2 might remain unchanged, showing consistent [HbR] values in healthy controls at rest. [HbO2] and StO2 decreased with increasing oxygen extraction ratio (O2ER). To date, whether muscle VO2 increases in patients with sepsis remains controversial (49,50). VO2 is physiologically dependent on perfusive oxygen delivery (QO2) below critical QO2 (51). QO2 depends on blood flow, the product of heart rate and cardiac output, and O2 concentrations in arterial and venous blood (15). Therefore, patients with an obvious decrease in blood flow have a physiological dependence of VO2 on QO2. However, whether pathological dependence of VO2 on QO2 occurs in septic patients has not yet been elucidated (52). Our findings are consistent with the findings of Menegueti et al., who found that VO2 was not increased in patients with sepsis (53). Efforts to reduce metabolic demands from skeletal muscles using neuromuscular blockade do not alter VO2, QO2, and O2ER in septic patients (54). The above findings explain why maintaining supranormal QO2 may not benefit critically ill patients, as tissue oxygen demand may not be increased (55-57). Our study suggests that identifying the individual [HbT] and [HbR] using NIRS is crucial for non-invasively and indirectly understanding patients’ tissue perfusion and VO2. These data may help determine the peripheral tissue response to altered systemic haemodynamics using inotropic agents and fluid resuscitation in patients with severe sepsis.
As an estimation of tissue perfusion and oxygenation, StO2 is correlated with QO2, responds to the ischaemic challenge, and may be associated with survival outcomes in patients with severe sepsis (19-21,58,59). Nevertheless, the clinical significance of StO2 remains controversial. Baseline StO2 cannot be used for the early detection of severe sepsis, and the routine implementation of resuscitation protocols incorporating StO2 >80% as a target does not provide a survival benefit (60,61). An important reason for these controversial findings is that StO2 is confounded by several factors, including tissue oedema (23). Compared with baseline StO2, the measurement of the change in StO2 reperfusion slope during a vascular occlusion test (VOT) may eliminate the personal confounding factor of tissue oedema and is significantly related to microcirculation in patients with septic shock and the outcome of septic patients (46,62). However, VOT is not convenient for continuous real-time monitoring of microcirculation. In the present study, we found that StO2 was significantly negatively correlated with [H2O], which resulted from the negative correlation between [H2O] and [HbO2]. In addition, the relationship between [HbO2], [HbR], and StO2 and [H2O] became more relevant during the study period, suggesting that the impact of tissue H2O on tissue oxygenation may become increasingly apparent during ICU admission. Our study determined that regional tissue H2O interacts with tissue HbO2 and StO2. It remains unclear whether the oedematous interstitium physiologically leads to reduced regional tissue perfusion, thereby altering tissue oxygenation. More research is warranted to clarify the detailed mechanism by which tissue H2O affects tissue perfusion and oxygenation, and whether fluid administration for normalising unstable haemodynamics in septic shock causes tissue oedema, leading to tissue perfusion deterioration.
In sepsis-induced hypoperfusion, additional fluid administration after the initial resuscitation should be guided by frequent reassessments of the patient’s haemodynamic status (3). Most measurements are obtained by monitoring systemic haemodynamics, and systemic arterial pressure is one of the most commonly measured parameters. The present study found that the regional tissue oxygenation parameters, including [HbO2], [HbR], and StO2, were not related to the systemic arterial pressure in the early stage of ICU admission. Our findings seem to be consistent with the concept of loss of haemodynamic coherence between the macrocirculation and microcirculation in sepsis (6). Systemic arterial pressure poorly reflects regional tissue perfusion (63). In addition, microcirculation is profoundly disturbed in severe sepsis, and the organ tissue blood supply may not reflect tissue oxygenation (64). Meanwhile, as mentioned above, fluid volume expansion to increase systemic arterial pressure also increases intravascular hydrostatic pressure, which may exacerbate fluid accumulation and result in tissue oedema (65). The oedematous interstitium reduces capillary perfusion, which in turn worsens tissue oxygenation (66). This may explain why systemic arterial pressure was positively correlated with tissue H2O but was not related to tissue oxygenation in our study. Targeting an MAP level >65 mmHg is a general goal in the initial resuscitation of patients with septic shock (3). Jozwiak et al. recently found that the impact of a unique MAP target on peripheral oxygenation may differ widely among patients with septic shock (9). In this study, patients with severe sepsis were enrolled after ICU admission. Most of them had received initial fluid resuscitation for normalising systemic haemodynamics at the ER. Attempts to increase MAP levels early in ICU admission may not guarantee improved tissue oxygenation but may result in increased tissue H2O. These findings suggest that care should be taken when administering fluid to normalise systemic blood pressure during early ICU admission, particularly if initial fluid resuscitation has already been performed. Initial fluid resuscitation may improve sepsis-induced hypoperfusion and improve patient survival (4). However, continued fluid administration following initial resuscitation may be harmful and should be guided by careful assessment of intravascular volume status and organ perfusion (67). Tissue H2O can be measured using NIRS and is potentially helpful in appreciating the effectiveness of fluid therapy on regional tissue perfusion and oxygenation.
This study has some limitations. First, the estimated sample size required 70 people per group, but the healthy control group comprised only 30 people, reducing the statistical power. In addition, healthy controls had a lower mean age than those with severe sepsis. Therefore, differences in NIRS parameters may be attributed to differences in age. However, Rosenberry et al. recently found that age is related to NIRS-derived post-occlusion StO2 recovery kinetics, but not baseline forearm StO2 (68). Therefore, severe sepsis remained a significant factor contributing to the difference in NIRS parameters between the two groups. Another limitation is the lack of a multivariate analysis investigating the causal relationship between [H2O] and tissue oxygenation parameters. Correlation analysis offers only crude assessments of the linear relationships between [H2O] and tissue oxygenation parameters. The same limitation exists in the findings on the relationship between systemic arterial pressure and [H2O]. However, the results provide evidence for the interaction of tissue H2O with tissue Hb and StO2 used to assess tissue perfusion and oxygenation. Compared with baseline StO2, dynamic NIRS measurements are more relevant to sepsis-related microvascular dysfunction (19,69). However, we did not investigate the dynamic changes in [HbO2], [HbR], and [H2O] during VOT and their relationship to the StO2 recovery slope. This crucial issue will be investigated and examined in future studies.
Conclusions
A NIRS device transmitting tri-wavelength light can be used to concurrently assess regional tissue oxygenation and H2O content. The regional tissue H2O content was significantly increased in patients with severe sepsis. In the early phase of severe sepsis, elevated systemic arterial pressure may be related to increased regional tissue H2O, but not to tissue oxygenation. Therefore, the measurement of tissue H2O is crucial and should be considered when estimating microcirculation and tissue oxygenation in patients with severe sepsis. Further studies are needed to elucidate the physiological effect of tissue H2O on tissue oxygenation and whether it can be accurately measured.
Acknowledgments
Funding: This work was supported by the Chang Gung Medical Foundation of Taiwan (Nos. CORPG6E0111, CORPG6E0112, and CORPG6E0113 to Chin-Kuo Lin).
Footnote
Reporting Checklist: The authors have completed the STROBE reporting checklist. Available at https://qims.amegroups.com/article/view/10.21037/qims-22-127/rc
Conflicts of Interest: All authors have completed the ICMJE uniform disclosure form (available at https://qims.amegroups.com/article/view/10.21037/qims-22-127/coif). CKL received grants from the Chang Gung Medical Foundation of Taiwan (Nos. CORPG6E0111, CORPG6E0112, and CORPG6E0113) for the study. The other authors have no conflicts of interest to declare.
Ethical Statement: The authors are accountable for all aspects of the work in ensuring that questions related to the accuracy or integrity of any part of the work are appropriately investigated and resolved. The study was conducted in accordance with the Declaration of Helsinki (as revised in 2013). The study was approved by the Institutional Review Board of Chang Gung Medical Foundation (No. 103-5357B). Informed consent was obtained from the healthy volunteers and patients. Legal guardians provided informed consent to participate if the patient had cognitive impairment.
Open Access Statement: This is an Open Access article distributed in accordance with the Creative Commons Attribution-NonCommercial-NoDerivs 4.0 International License (CC BY-NC-ND 4.0), which permits the non-commercial replication and distribution of the article with the strict proviso that no changes or edits are made and the original work is properly cited (including links to both the formal publication through the relevant DOI and the license). See: https://creativecommons.org/licenses/by-nc-nd/4.0/.
References
- Singer M, Deutschman CS, Seymour CW, Shankar-Hari M, Annane D, Bauer M, Bellomo R, Bernard GR, Chiche JD, Coopersmith CM, Hotchkiss RS, Levy MM, Marshall JC, Martin GS, Opal SM, Rubenfeld GD, van der Poll T, Vincent JL, Angus DC. The Third International Consensus Definitions for Sepsis and Septic Shock (Sepsis-3). JAMA 2016;315:801-10. [Crossref] [PubMed]
- Astiz ME, Rackow EC, Falk JL, Kaufman BS, Weil MH. Oxygen delivery and consumption in patients with hyperdynamic septic shock. Crit Care Med 1987;15:26-8. [Crossref] [PubMed]
- Rhodes A, Evans LE, Alhazzani W, Levy MM, Antonelli M, Ferrer R, et al. Surviving Sepsis Campaign: International Guidelines for Management of Sepsis and Septic Shock: 2016. Intensive Care Med 2017;43:304-77. [Crossref] [PubMed]
- Evans L, Rhodes A, Alhazzani W, Antonelli M, Coopersmith CM, French C, et al. Surviving Sepsis Campaign: International Guidelines for Management of Sepsis and Septic Shock 2021. Crit Care Med 2021;49:e1063-143. [Crossref] [PubMed]
- Jaffee W, Hodgins S, McGee WT. Tissue Edema, Fluid Balance, and Patient Outcomes in Severe Sepsis: An Organ Systems Review. J Intensive Care Med 2018;33:502-9. [Crossref] [PubMed]
- Ince C. Hemodynamic coherence and the rationale for monitoring the microcirculation. Crit Care 2015;19:S8. [Crossref] [PubMed]
- De Backer D, Creteur J, Preiser JC, Dubois MJ, Vincent JL. Microvascular blood flow is altered in patients with sepsis. Am J Respir Crit Care Med 2002;166:98-104. [Crossref] [PubMed]
- Boerma EC, van der Voort PH, Spronk PE, Ince C. Relationship between sublingual and intestinal microcirculatory perfusion in patients with abdominal sepsis. Crit Care Med 2007;35:1055-60. [Crossref] [PubMed]
- Jozwiak M, Chambaz M, Sentenac P, Monnet X, Teboul JL. Assessment of tissue oxygenation to personalize mean arterial pressure target in patients with septic shock. Microvasc Res 2020;132:104068. [Crossref] [PubMed]
- Lam C, Tyml K, Martin C, Sibbald W. Microvascular perfusion is impaired in a rat model of normotensive sepsis. J Clin Invest 1994;94:2077-83. [Crossref] [PubMed]
- Farquhar I, Martin CM, Lam C, Potter R, Ellis CG, Sibbald WJ. Decreased capillary density in vivo in bowel mucosa of rats with normotensive sepsis. J Surg Res 1996;61:190-6. [Crossref] [PubMed]
- Bakker J, Ince C. Monitoring coherence between the macro and microcirculation in septic shock. Curr Opin Crit Care 2020;26:267-72. [Crossref] [PubMed]
- Valeanu L, Bubenek-Turconi SI, Ginghina C, Balan C. Hemodynamic Monitoring in Sepsis-A Conceptual Framework of Macro- and Microcirculatory Alterations. Diagnostics (Basel) 2021;11:1559. [Crossref] [PubMed]
- Charlton M, Sims M, Coats T, Thompson JP. The microcirculation and its measurement in sepsis. J Intensive Care Soc 2017;18:221-7. [Crossref] [PubMed]
- Barstow TJ. Understanding near infrared spectroscopy and its application to skeletal muscle research. J Appl Physiol (1985) 2019;126:1360-76. [PubMed]
- Jöbsis FF. Noninvasive, infrared monitoring of cerebral and myocardial oxygen sufficiency and circulatory parameters. Science 1977;198:1264-7. [Crossref] [PubMed]
- Davis ML, Barstow TJ. Estimated contribution of hemoglobin and myoglobin to near infrared spectroscopy. Respir Physiol Neurobiol 2013;186:180-7. [Crossref] [PubMed]
- Mancini DM, Bolinger L, Li H, Kendrick K, Chance B, Wilson JR. Validation of near-infrared spectroscopy in humans. J Appl Physiol (1985) 1994;77:2740-7. [PubMed]
- Creteur J, Carollo T, Soldati G, Buchele G, De Backer D, Vincent JL. The prognostic value of muscle StO2 in septic patients. Intensive Care Med 2007;33:1549-56. [Crossref] [PubMed]
- Leone M, Blidi S, Antonini F, Meyssignac B, Bordon S, Garcin F, Charvet A, Blasco V, Albanèse J, Martin C. Oxygen tissue saturation is lower in nonsurvivors than in survivors after early resuscitation of septic shock. Anesthesiology 2009;111:366-71. [Crossref] [PubMed]
- Colin G, Nardi O, Polito A, Aboab J, Maxime V, Clair B, Friedman D, Orlikowski D, Sharshar T, Annane D. Masseter tissue oxygen saturation predicts normal central venous oxygen saturation during early goal-directed therapy and predicts mortality in patients with severe sepsis. Crit Care Med 2012;40:435-40. [Crossref] [PubMed]
- Lee WL, Slutsky AS. Sepsis and endothelial permeability. N Engl J Med 2010;363:689-91. [Crossref] [PubMed]
- Poeze M. Tissue-oxygenation assessment using near-infrared spectroscopy during severe sepsis: confounding effects of tissue edema on StO2 values. Intensive Care Med 2006;32:788-9. [Crossref] [PubMed]
- Kuo HC, Lo CC, Lin PX, Kao CC, Huang YH, Lin BS. Wireless optical monitoring system identifies limb induration characteristics in patients with Kawasaki disease. J Allergy Clin Immunol 2018;142:710-1. [Crossref] [PubMed]
- Wang L, Jacques SL, Zheng L. MCML--Monte Carlo modeling of light transport in multi-layered tissues. Comput Methods Programs Biomed 1995;47:131-46. [Crossref] [PubMed]
- Bashkatov AN, Genina EA, Kochubey VI, Tuchin VV. Optical properties of human skin, subcutaneous and mucous tissues in the wavelength range from 400 to 2000 nm. Journal of Physics D: Applied Physics 2005;38:2543. [Crossref]
- Molavi B, Shadgan B, Macnab AJ, Dumont GA. Noninvasive Optical Monitoring of Bladder Filling to Capacity Using a Wireless Near Infrared Spectroscopy Device. IEEE Trans Biomed Circuits Syst 2014;8:325-33. [Crossref] [PubMed]
- Cope M, Delpy DT, Reynolds EO, Wray S, Wyatt J, van der Zee P. Methods of quantitating cerebral near infrared spectroscopy data. Adv Exp Med Biol 1988;222:183-9. [Crossref] [PubMed]
- Delpy DT, Cope M, van der Zee P, Arridge S, Wray S, Wyatt J. Estimation of optical pathlength through tissue from direct time of flight measurement. Phys Med Biol 1988;33:1433-42. [Crossref] [PubMed]
- Dellinger RP, Levy MM, Rhodes A, Annane D, Gerlach H, Opal SM, et al. Surviving sepsis campaign: international guidelines for management of severe sepsis and septic shock: 2012. Crit Care Med 2013;41:580-637. [Crossref] [PubMed]
- Sadaka F, Aggu-Sher R, Krause K, O'Brien J, Armbrecht ES, Taylor RW. The effect of red blood cell transfusion on tissue oxygenation and microcirculation in severe septic patients. Ann Intensive Care 2011;1:46. [Crossref] [PubMed]
- Packman MI, Rackow EC. Optimum left heart filling pressure during fluid resuscitation of patients with hypovolemic and septic shock. Crit Care Med 1983;11:165-9. [Crossref] [PubMed]
- Kaufman BS, Rackow EC, Falk JL. The relationship between oxygen delivery and consumption during fluid resuscitation of hypovolemic and septic shock. Chest 1984;85:336-40. [Crossref] [PubMed]
- Molnár Z, Mikor A, Leiner T, Szakmány T. Fluid resuscitation with colloids of different molecular weight in septic shock. Intensive Care Med 2004;30:1356-60. [Crossref] [PubMed]
- Hahn RG, Lyons G. The half-life of infusion fluids: An educational review. Eur J Anaesthesiol 2016;33:475-82. [Crossref] [PubMed]
- Groeneveld AB, Teule GJ, Bronsveld W, van den Bos GC, Thijs LG. Increased systemic microvascular albumin flux in septic shock. Intensive Care Med 1987;13:140-2. [Crossref] [PubMed]
- Dubniks M, Persson J, Grände PO. Effect of blood pressure on plasma volume loss in the rat under increased permeability. Intensive Care Med 2007;33:2192-8. [Crossref] [PubMed]
- Armistead CW Jr, Vincent JL, Preiser JC, De Backer D. Thuc Le Minh. Hypertonic saline solution-hetastarch for fluid resuscitation in experimental septic shock. Anesth Analg 1989;69:714-20. [Crossref] [PubMed]
- Ospina-Tascon G, Neves AP, Occhipinti G, Donadello K, Büchele G, Simion D, Chierego ML, Silva TO, Fonseca A, Vincent JL, De Backer D. Effects of fluids on microvascular perfusion in patients with severe sepsis. Intensive Care Med 2010;36:949-55. [Crossref] [PubMed]
- Maitland K, Kiguli S, Opoka RO, Engoru C, Olupot-Olupot P, Akech SO, Nyeko R, Mtove G, Reyburn H, Lang T, Brent B, Evans JA, Tibenderana JK, Crawley J, Russell EC, Levin M, Babiker AG, Gibb DMFEAST Trial Group. Mortality after fluid bolus in African children with severe infection. N Engl J Med 2011;364:2483-95. [Crossref] [PubMed]
- Sirvent JM, Ferri C, Baró A, Murcia C, Lorencio C. Fluid balance in sepsis and septic shock as a determining factor of mortality. Am J Emerg Med 2015;33:186-9. [Crossref] [PubMed]
- Mesquida J, Gruartmoner G, Espinal C. Skeletal muscle oxygen saturation (StO2) measured by near-infrared spectroscopy in the critically ill patients. Biomed Res Int 2013;2013:502194. [Crossref] [PubMed]
- Beilman GJ, Groehler KE, Lazaron V, Ortner JP. Near-infrared spectroscopy measurement of regional tissue oxyhemoglobin saturation during hemorrhagic shock. Shock 1999;12:196-200. [Crossref] [PubMed]
- Nagashima Y, Yada Y, Hattori M, Sakai A. Development of a new instrument to measure oxygen saturation and total hemoglobin volume in local skin by near-infrared spectroscopy and its clinical application. Int J Biometeorol 2000;44:11-9. [Crossref] [PubMed]
- Comerota AJ, Throm RC, Kelly P, Jaff M. Tissue (muscle) oxygen saturation (StO2): a new measure of symptomatic lower-extremity arterial disease. J Vasc Surg 2003;38:724-9. [Crossref] [PubMed]
- Neto AS, Pereira VG, Manetta JA, Espósito DC, Schultz MJ. Association between static and dynamic thenar near-infrared spectroscopy and mortality in patients with sepsis: a systematic review and meta-analysis. J Trauma Acute Care Surg 2014;76:226-33. [Crossref] [PubMed]
- Alvares TS, Oliveira GV, Soares R, Murias JM. Near-infrared spectroscopy-derived total haemoglobin as an indicator of changes in muscle blood flow during exercise-induced hyperaemia. J Sports Sci 2020;38:751-8. [Crossref] [PubMed]
- Hammer SM, Alexander AM, Didier KD, Smith JR, Caldwell JT, Sutterfield SL, Ade CJ, Barstow TJ. The noninvasive simultaneous measurement of tissue oxygenation and microvascular hemodynamics during incremental handgrip exercise. J Appl Physiol (1985) 2018;124:604-14. [PubMed]
- Girardis M, Rinaldi L, Busani S, Flore I, Mauro S, Pasetto A. Muscle perfusion and oxygen consumption by near-infrared spectroscopy in septic-shock and non-septic-shock patients. Intensive Care Med 2003;29:1173-6. [Crossref] [PubMed]
- De Blasi RA, Palmisani S, Alampi D, Mercieri M, Romano R, Collini S, Pinto G. Microvascular dysfunction and skeletal muscle oxygenation assessed by phase-modulation near-infrared spectroscopy in patients with septic shock. Intensive Care Med 2005;31:1661-8. [Crossref] [PubMed]
- Baigorri F, Russell JA. Oxygen delivery in critical illness. Crit Care Clin 1996;12:971-94. [Crossref] [PubMed]
- Russell JA, Phang PT. The oxygen delivery/consumption controversy. Approaches to management of the critically ill. Am J Respir Crit Care Med 1994;149:533-7. [Crossref] [PubMed]
- Menegueti MG, de Araújo TR, Laus AM, Martins-Filho OA, Basile-Filho A, Auxiliadora-Martins M. Resting Energy Expenditure and Oxygen Consumption in Critically Ill Patients With vs Without Sepsis. Am J Crit Care 2019;28:136-41. [Crossref] [PubMed]
- Freebairn RC, Derrick J, Gomersall CD, Young RJ, Joynt GM. Oxygen delivery, oxygen consumption, and gastric intramucosal pH are not improved by a computer-controlled, closed-loop, vecuronium infusion in severe sepsis and septic shock. Crit Care Med 1997;25:72-7. [Crossref] [PubMed]
- Gutierrez G, Palizas F, Doglio G, Wainsztein N, Gallesio A, Pacin J, Dubin A, Schiavi E, Jorge M, Pusajo J. Gastric intramucosal pH as a therapeutic index of tissue oxygenation in critically ill patients. Lancet 1992;339:195-9. [Crossref] [PubMed]
- Yu M, Levy MM, Smith P, Takiguchi SA, Miyasaki A, Myers SA. Effect of maximizing oxygen delivery on morbidity and mortality rates in critically ill patients: a prospective, randomized, controlled study. Crit Care Med 1993;21:830-8. [Crossref] [PubMed]
- Russell JA. Adding fuel to the fire--the supranormal oxygen delivery trials controversy. Crit Care Med 1998;26:981-3. [Crossref] [PubMed]
- Mesquida J, Gruartmoner G, Martínez ML, Masip J, Sabatier C, Espinal C, Artigas A, Baigorri F. Thenar oxygen saturation and invasive oxygen delivery measurements in critically ill patients in early septic shock. Shock 2011;35:456-9. [Crossref] [PubMed]
- Vorwerk C, Coats TJ. The prognostic value of tissue oxygen saturation in emergency department patients with severe sepsis or septic shock. Emerg Med J 2012;29:699-703. [Crossref] [PubMed]
- Goulet H, André S, Sahakian GD, Freund Y, Khelifi G, Claessens YE, Riou B, Ray P. Accuracy of oxygen tissue saturation values in assessing severity in patients with sepsis admitted to emergency departments. Eur J Emerg Med 2014;21:266-71. [Crossref] [PubMed]
- Nardi O, Zavala E, Martin C, Nanas S, Scheeren T, Polito A, Borrat X, Annane D. Targeting skeletal muscle tissue oxygenation (StO2) in adults with severe sepsis and septic shock: a randomised controlled trial (OTO-StS Study). BMJ Open 2018;8:e017581. [Crossref] [PubMed]
- Payen D, Luengo C, Heyer L, Resche-Rigon M, Kerever S, Damoisel C, Losser MR. Is thenar tissue hemoglobin oxygen saturation in septic shock related to macrohemodynamic variables and outcome? Crit Care 2009;13:S6. [Crossref] [PubMed]
- Pinsky MR, Payen D. Functional hemodynamic monitoring. Crit Care 2005;9:566-72. [Crossref] [PubMed]
- Østergaard L, Granfeldt A, Secher N, Tietze A, Iversen NK, Jensen MS, Andersen KK, Nagenthiraja K, Gutiérrez-Lizardi P, Mouridsen K, Jespersen SN, Tønnesen EK. Microcirculatory dysfunction and tissue oxygenation in critical illness. Acta Anaesthesiol Scand 2015;59:1246-59. [Crossref] [PubMed]
- Obonyo NG, Fanning JP, Ng AS, Pimenta LP, Shekar K, Platts DG, Maitland K, Fraser JF. Effects of volume resuscitation on the microcirculation in animal models of lipopolysaccharide sepsis: a systematic review. Intensive Care Med Exp 2016;4:38. [Crossref] [PubMed]
- Jerome SN, Akimitsu T, Korthuis RJ. Leukocyte adhesion, edema, and development of postischemic capillary no-reflow. Am J Physiol 1994;267:H1329-36. [PubMed]
- Malbrain MLNG, Langer T, Annane D, Gattinoni L, Elbers P, Hahn RG, De Laet I, Minini A, Wong A, Ince C, Muckart D, Mythen M, Caironi P, Van Regenmortel N. Intravenous fluid therapy in the perioperative and critical care setting: Executive summary of the International Fluid Academy (IFA). Ann Intensive Care 2020;10:64. [Crossref] [PubMed]
- Rosenberry R, Munson M, Chung S, Samuel TJ, Patik J, Tucker WJ, Haykowsky MJ, Nelson MD. Age-related microvascular dysfunction: novel insight from near-infrared spectroscopy. Exp Physiol 2018;103:190-200. [Crossref] [PubMed]
- Skarda DE, Mulier KE, Myers DE, Taylor JH, Beilman GJ. Dynamic near-infrared spectroscopy measurements in patients with severe sepsis. Shock 2007;27:348-53. [Crossref] [PubMed]