Predictive value of thrombolysis in myocardial infarction frame count for coronary microvascular dysfunction evaluated with an angiography-derived index of microcirculatory resistance in patients with coronary slow flow
Introduction
Coronary slow flow (CSF) is characterized by the delayed antegrade progression of the contrast injected from the proximal to the distal coronary artery during angiography (1). Patients with CSF usually report frequent chest pain (2). Additionally, CSF is not benign and is associated with various clinical adverse events, including acute myocardial infarction (AMI), malignant arrhythmia, and sudden cardiac death (3-5). According to the thrombolysis in myocardial infarction (TIMI) flow grade, a flow grade of TIMI 1–2 is considered to be CSF in clinical practice. Another method that quantifies the flow velocity by corrected TIMI frame counts (CTFC) is also used. A potential cause of CSF is impaired microvascular dilation and increased microvascular resistance, which characterizes coronary microvascular dysfunction (CMD) (1).
The index of microvascular resistance (IMR) is a reliable method to assess CMD (6). However, using a pressure wire to measure IMR has inevitable practical restrictions due to cost and technical and procedural complexities (7). A novel coronary angiography-derived IMR (caIMR) has been validated for its accuracy compared with wire-based IMR and is being increasingly used to evaluate CMD (8). The caIMR does not require a pressure wire or adenosine use and is convenient to perform. Therefore, we used this novel method to assess CMD.
Although CMD may explain the emergence of CSF (9), the precise association between CSF and CMD remains unclear. The aim of the present study was thus to clarify this relationship. We present the following article in accordance with the STARD reporting checklist (available at https://qims.amegroups.com/article/view/10.21037/qims-22-224/rc).
Methods
Study population
We adopted a retrospective and case-control design and consecutively enrolled patients with CSF from January 2017 to March 2018. The inclusion criteria of this study were as follows: (I) non-obstructive epicardial coronary arteries [i.e., angiographic stenosis <40% (10)]; (II) delayed distal vessels contrast opacification as evidenced by TIMI 1–2 flow [i.e., requiring more than 3 beats to opacity the distal vessel (11)], and (III) delayed distal vessels contrast in at least 1 epicardial vessel. The exclusion criteria were the following: coronary ectasia, coronary emboli, poor quality of angiographic images precluding IMR measurement (e.g., substantial foreshortening or overlap of the vessels, absence of 2 angiographic projections with the view of at least 30°, and insufficient contrast flush), heart failure, atrial fibrillation, valvular disease, and connecting tissue disorders. Concurrently, we randomly selected 39 control participants with normal coronary flow and normal or near-normal arteries from a database of catheter laboratories. According to the presence or absence of CSF, participants were divided into a CSF group and a non-CSF group.
The study protocol was approved by the independent institutional ethics committee of the First Affiliated Hospital of Nanjing Medical University and complied with the Declaration of Helsinki (as revised in 2013). Written informed consent was acquired from all patients. There was no registration requirement for this study.
Analysis of corrected TIMI frame count
Coronary angiography was performed following the standard Judkins technique. Angiograms were obtained in the right and left views, and in the cranial and caudal positions. Two well-trained cardiologists reviewed and analyzed the included images (MHL and HW). The TIMI frame count (TFC) was obtained by counting the difference between the first and last frames. The first frame is the frame where dye first fully enters the artery, which occurs when 3 conditions are met (12): (I) fully or near fully concentrated dye extends across the entire artery lumen; (II) dye touches both borders of the artery; and (III) dye has antegrade motion. The last frame is the frame when dye first enters the distal landmark branch. For the left anterior descending artery (LAD), the landmark branch is the distal bifurcation (e.g., the “mustache”, “pitchfork”, or “whale’s tail”). In the circumflex system, the distal branch is the bifurcation with the longest total distance. In the right coronary artery (RCA), the landmark branch is the first branch of the posterolateral artery. The standard coronary angiogram cine acquisition was performed at 30 frames per second. The coronary acquisition in this study was recorded at 15 frames per second. Therefore, the corrected TIMI frame counts (CTFC) were calculated as the number of frames multiplied by 2. Notably, the CTFC of the LAD had to be divided by 1.7 due to its increased length (12).
Measurement of caIMR
The operator performing caIMR computation (Rainmed Medical Technology Co., Ltd, Suzhou, China) was blind to CTFC data. To assess caIMR, 2 angiographic images of the target vessel separated by at least 30° were selected to reconstruct a 3-dimensional (3D) model of the coronary artery. Invasive aortic blood pressure was reviewed from the data of the institutional catheter center and was input into the FlashAngio console. Then, caIMR was calculated by FlashAngio software with a proprietary fluid dynamic algorithm (8). according to the following formula: caIMR = Pdhyp × (L/K × Vdiastole), where Pdhyp is the mean invasive aortic pressure, L is the length of the selected vessel from the proximal inlet to the distal position, Vdiastole is the mean flow velocity (frames count of the selected segment of a vessel) at diastole, and K is a constant (K=2.1). Simultaneously, coronary angiography–derived fractional flow reserve (caFFR) was also recorded from the FlashAngio system. The calculation of caFFR has been reported in a previous study (13). Consistent with IMR, a caIMR >40 U was identified as a significant CMD (7).
Laboratory and echocardiographic data collection
Blood samples were collected to assess total cholesterol (TC), triglyceride (TG), low-density lipoprotein cholesterol (LDL-C), high-density lipoprotein cholesterol (HDL-C), lipid protein a (Lp-a), fasting blood glucose (FBG), white blood count (WBC), and neutrophils (NE) in the first morning of admission. Data of transthoracic echocardiography included E/e’ (a ratio of peak velocity of early diastole of left ventricular to peak velocity of early diastole of the root of the mitral annulus) and left ventricular ejection fraction (LVEF).
Sample size estimation
We used the tests for 1 receiver operating characteristic (ROC) curve. Presetting the power to 80%, the 2-sided α to 0.05, the standard area under the curve (AUC) to 0.7 (H0 hypothesis), and the new AUC =to 0.9 (H1 hypothesis) output a sample size of 42 arteries. Considering we would assess the ROC analysis stratified by the LAD, left circumflex artery (LCX), and RCA, the target sample size of RCA was reached until we screened participants in March 2018. Due to the consecutive enrollment, the final sample size of the study was 254. PASS software (version 15.0.5) was used in the estimation of the sample size.
Statistical analysis
The Kolmogorov-Smirnov test determined data distribution. Continuous data with a normal distribution are presented as mean ± deviation and were analyzed with an independent samples t-test. Nonnormally distributed continuous data are reported as median with interquartile range (IQR) and were compared by the nonparametric Man-Whitney U test. Categorical variables were calculated with counts and percentages and were assessed using the chi-square test or Fisher exact test. The correlation between caIMR and CTFC was analyzed by calculating the Pearson R correlation coefficients for variables with normal distribution or Spearman rank correlation coefficients for variables with a nonnormal distribution. A univariate analysis was performed to estimate the potential predictors for CMD, and a multivariate regression analysis was used to identify the independent predictors of CMD, in which variables with P<0.10 in the univariate analysis were included. ROC analysis was applied to assess the best cutoff values of CTFC for predicting CMD using MedCalc (version 18.2.1, MedCalc Software, Mariakerke, Belgium). The optimal threshold was calculated using the Youden index. Sensitivity and specificity with 95% CIs were determined for each cutoff value. A P value <0.05 was considered statistically significant. There were no missing data in this study. Statistical analyses were performed using SPSS 21.0 software (IBM Corp., Armonk, NY, USA).
Results
A total of 111 patients with CSF and 39 patients without CSF (non-CSF group) were enrolled in this study (Table 1). Functional assessment of microvascular resistance was analyzed in 254 arteries, including 165 arteries for the CSF and 89 arteries for the non-CSF groups (Figure 1). Compared with the non-CSF group, the CSF group had a greater proportion of male patients (65.8% vs. 23.1%; P<0.001), had less prevalent hypertension (47.7% vs. 67.7%; P=0.042), had a lower level of HDL-C (P=0.032), and had a lower E/e’ (P=0.015). Additionally, the CSF group had higher CTFC, caFFR, and caIMR in the LAD, LCX, and RCA (all P<0.001). After division of patients with CSF into TIMI grade 1 and TIMI grade 2, it was found that 9 patients identified as TIMI grade 1 had higher CTFC and caIMR (Figure S1). Figure 2 presents the correlation between CTFC and caIMR. A strong correlation was observed for all arteries (R=0.712; P<0.001). The correlations between CTFC and caIMR in the LAD or RCA were even stronger (R=0.897 in the LAD; R=0.863 in the RCA; all P<0.001).
Table 1
Characteristics | All (n=150) | CSF group (n=111) | Non-CSF group (n=39) | P value |
---|---|---|---|---|
Age (years) | 61.7±10.4 | 60.7±11.1 | 64.3±7.9 | 0.065 |
Male | 82 (54.7) | 73 (65.8) | 9 (23.1) | <0.001*** |
Hypertension | 79 (52.7) | 53 (47.7) | 26 (66.7) | 0.042* |
Diabetes mellitus | 19 (12.7) | 13 (11.7) | 6 (15.4) | 0.553 |
Hyperlipidemia | 34 (22.7) | 27 (24.3) | 7 (17.9) | 0.413 |
Stroke | 18 (12.0) | 12 (10.8) | 6 (15.4) | 0.450 |
WBC (×109/L) | 5.57 [4.54–6.73] | 5.39 [4.54–6.56] | 5.65 [4.50–7.19] | 0.564 |
NE (×109/L) | 3.15 [2.53–4.11] | 3.13 [2.51–4.02] | 3.27 [2.65–4.31] | 0.690 |
FBG (mmol/L) | 5.04 [4.58–5.57] | 5.01 [4.54–5.55] | 5.21 [4.69–5.65] | 0.309 |
TC (mmol/L) | 4.57±2.97 | 4.63±3.40 | 4.38±0.93 | 0.671 |
TG (mmol/L) | 1.45±0.84 | 1.43±0.86 | 1.48±0.79 | 0.777 |
HDL-C (mmol/L) | 1.10±0.24 | 1.08±0.23 | 1.18±0.25 | 0.032* |
LDL-C (mmol/L) | 2.74±0.80 | 2.75±0.84 | 2.71±0.84 | 0.825 |
Lp(a) (mg/L) | 129 [77–267] | 129 [86–281] | 157 [65–256] | 0.490 |
LVEF (%) | 61.8±8.8 | 62.1±8.3 | 61.1±10.5 | 0.569 |
E/e’ | 9.1±3.1 | 8.8±2.8 | 10.4±3.6 | 0.015* |
CTFC (frames) | ||||
LAD | 42.5±16.3 | 48.6±14.9 | 27.5±7.2 | <0.001*** |
LCX | 61.5±23.9 | 74.4±21.9 | 45.1±14.6 | <0.001*** |
RCA | 50.8±22.0 | 61.7±20.0 | 32.7±8.8 | <0.001*** |
caFFR | ||||
LAD | 0.96±0.03 | 0.96±0.03 | 0.95±0.02 | 0.031* |
LCX | 0.96±0.02 | 0.97±0.01 | 0.96±0.02 | <0.001*** |
RCA | 0.95±0.03 | 0.96±0.02 | 0.93±0.03 | 0.001** |
caIMR (U) | ||||
LAD | 47.9±20.5 | 55.9±18.1 | 22.1±9.7 | <0.001*** |
LCX | 42.3±15.8 | 50.9±12.7 | 31.2±12.0 | <0.001*** |
RCA | 37.6±16.9 | 45.2±16.6 | 25.0±6.9 | <0.001*** |
*, P<0.05; **, P<0.01; ***, P<0.001. The data are expressed as n (%) or mean ± SD or median (Q1–Q3). CSF, coronary slow flow; WBC, white blood cell; NE, neutrophil; FBG, fasting blood glucose; TC, total cholesterol; TG, triglyceride; HDL-C, high-density lipoprotein cholesterol; LDL-C, low-density lipoprotein cholesterol; Lp(a), lipoprotein (a); LVEF, left ventricular ejection fraction; E, peak velocity of early diastole of left ventricular; e’, peak velocity of early diastole of the root of mitral annulus; CTFC, corrected TIMI frame count; LAD, left anterior descending artery; LCX, left circumflex artery; RCA, right coronary artery; caFFR, coronary angiography-derived fractional flow reserve; caIMR, coronary angiography-derived index of microvascular resistance.
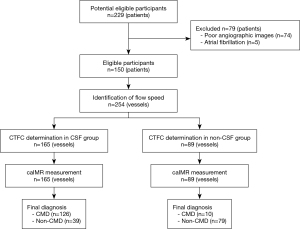
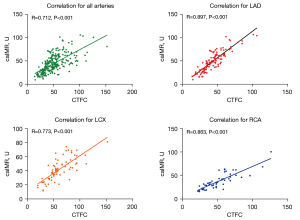
According to the value of caIMR, patients were assigned to 2 groups (CMD group: caIMR > 40 U; non-CMD group: caIMR ≤40 U). Figure 3 shows that the CTFC of all arteries was significantly higher in the CMD group than in the non-CMD group (61.33±20.85 vs. 36.26±12.60; P<0.001). The CTFC of each artery was still higher in the CMD group (all P<0.001). In the univariate analysis (Table 2), male sex [hazard ratio (HR) 2.63, 95% CI: 1.30–5.31], E/e’ (HR =0.88, 95% CI: 0.78–0.99), CTFC (HR =1.12, 95% CI: 1.09–1.16), and caFFR (HR =1.81, 95% CI: 1.50–2.17) were closely related to CMD. After adjustment for covariates, male sex (HR =2.72, 95% CI: 1.22–6.06), CTFC (HR =1.10, 95% CI: 1.07–1.14), and caFFR (HR =1.22, 95% CI: 1.00–1.50) were independent predictors for CMD.
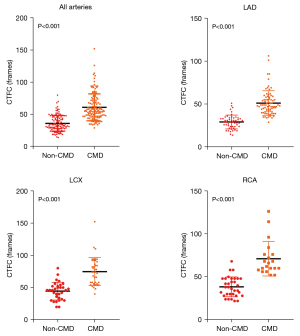
Table 2
Characteristics | Univariate analyses | Multivariate analyses | |||
---|---|---|---|---|---|
HR (95% CI) | P value | HR (95% CI) | P value | ||
Age (years) | 1.00 (0.96–1.03) | 0.765 | |||
Male | 2.63 (1.30–5.31) | 0.007** | 2.72 (1.22–6.06) | 0.015* | |
Hypertension | 0.60 (0.30–1.20) | 0.145 | |||
Diabetes mellitus | 1.06 (0.38–3.00) | 0.914 | |||
Hyperlipidemia | 0.73 (0.33–1.61) | 0.432 | |||
Stroke | 1.30 (0.44–3.88) | 0.638 | |||
WBC (×109/L) | 0.99 (0.80–1.23) | 0.928 | |||
NE (×109/L) | 0.98 (0.73–1.31) | 0.879 | |||
FBG (mmol/L) | 0.98 (0.74–1.31) | 0.906 | |||
TC (mmol/L) | 0.87 (0.67–1.13) | 0.298 | |||
TG (mmol/L) | 0.80 (0.53–1.19) | 0.264 | |||
HDL-C (mmol/L) | 0.28 (0.07–1.2) | 0.087 | |||
LDL-C (mmol/L) | 1.04 (0.67–1.60) | 0.877 | |||
Lp (a) (mg/L) | 1.00 (0.99–1.00) | 0.843 | |||
LVEF (%) | 1.03 (0.98–1.08) | 0.223 | |||
E/e’ | 0.88 (0.78–0.99) | 0.043* | |||
CTFC (frames) | 1.12 (1.09–1.16) | <0.001*** | 1.10 (1.07–1.14) | <0.001*** | |
caFFR, per 100 units | 1.81 (1.50–2.17) | <0.001*** | 1.22 (1.00–1.50) | 0.047* |
*, P<0.05; **, P<0.01; ***, P<0.001. CMD, coronary microvascular dysfunction; WBC, white blood cell; NE, neutrophil; FBG, fasting blood glucose; TC, total cholesterol; TG, triglyceride; HDL-C, high-density lipoprotein cholesterol; LDL-C, low-density lipoprotein cholesterol; Lp(a), lipoprotein (a); LVEF, left ventricular ejection fraction; E, peak velocity of early diastole of left ventricular; e’, peak velocity of early diastole of the root of mitral annulus; CTFC, corrected thrombolysis in myocardial infarction frame count; caFFR, coronary angiography-derived fractional flow reserve.
ROC analysis indicated that CTFC could predict CMD, with an AUC =of 0.873 for all arteries, an AUC =of 0.948 for the LAD, an AUC =of 0.906 for the LCX, and an AUC =of 0.968 for the RCA (Figure 4). Additionally, as shown in Table 3, the best cutoff value of CTFC of all arteries for predicting CMD was 38 frames, with a sensitivity of 92.8% and a specificity of 63.8% (P<0.001). Moreover, the best cutoff value of CTFC for the LAD, LCX, and RCA in identifying CMD was 35 frames, 52 frames, and 50 frames, respectively (all P<0.001). Table S1 shows the cross-tabulation of the CTFC of all arteries by caIMR.
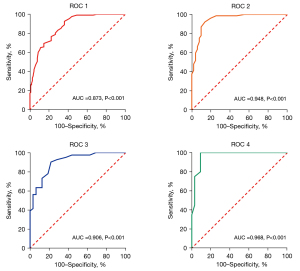
Table 3
Target vessels | Positive threshold | AUC | Sensitivity (%) | Specificity (%) | P value |
---|---|---|---|---|---|
All arteries | >38 | 0.873 | 92.8 | 63.8 | <0.001*** |
LAD | >35 | 0.948 | 92.2 | 86.3 | <0.001*** |
LCX | >52 | 0.906 | 90.2 | 78.1 | <0.001*** |
RCA | >50 | 0.968 | 100 | 90.9 | <0.001*** |
***, P<0.001. CTFC, corrected thrombolysis in myocardial infarction frame count; CMD, coronary microvascular dysfunction; AUC, area under the curve; LAD, left anterior descending artery; LCX, left circumflex artery; RCA, right coronary artery.
Discussion
The present study evaluated the correlation between CSF and CMD defined with caIMR. First, we found that caIMR was significantly higher in patients with CSF than in those without CSF. Additionally, CTFC correlated well with caIMR. Finally, CTFC had good predictive power in identifying CMD.
CSF during coronary angiography is quite uncommon in patients with suspected coronary artery disease. Reports show that the prevalence of CSF varies from 1% to 7% (3,14-16). Some studies defined CSF as CTFC of more than 27 frames of all arteries, while several studies identified CSF using TIMI grade (14-16). In our study, when defining CSF as TIMI grade I or II, the prevalence of CSF was 5.2%, which was within the range of the previous report (3,14-16).
Most studies indicated male sex, a lower level of HDL-C, higher BMI, and impaired glucose metabolism to be independent predictors of CSF, with the traditional risk factors of CAD (e.g., diabetes, hyperlipidemia, stroke) not playing a role in patients with CSF (3,15). We also found that patients with CSF were more likely to be male and have a lower level of HDL-C. Sanati et al. (3) reported an increased prevalence of hypertension in patients with CSF, but the majority of studies indicate there to be no difference between CSF and controls in terms of hypertension (14,15). Nevertheless, we found a decreased prevalence of hypertension in those with CSF. In this case, the P value was quite close to 0.05; we believed that the small sample size of the control group contributed to the significant difference in hypertension between the two groups.
Theoretically, the main pathogenetic mechanism of CSF is the inability of coronary arteries to regulate the blood flow to supply the increased oxygen demand (17). As epicardial arteries do not resist blood flow, small vessels of <400 µm are regarded as resistant vessels that influence the myocardial blood flow (4). One histopathological study presented evidence of extensive structural abnormalities of coronary small vessels in patients with CSF (18), while another study demonstrated TIMI flow grade to be an independent predictor for decreased myocardial blood flow (19). IMR is a valuable way to assess the microvascular resistance generated by distal or small coronary vessels. In recent years, angiography-derived IMR has been validated for its accuracy compared with wire-based IMR (7,8). The present study is the first study to use wire-free IMR to evaluate the microvascular resistance in patients with CSF. We found that caIMR was significantly higher in the CSF group than in the non-CSF group, which was consistent with the above theory.
In clinical practice, the most commonly used method for identifying blood flow velocity is TIMI grade classification, which is a semiquantitative index to assess CSF, with the more quantitative and objective measure of coronary blood flow for the assessment of CSF being CTFC (14). In our study, we identified CSF by using TIMI grade and determined the CTFC. When comparing CTFC with caIMR, we found strong correlations between CTFC and caIMR. The key to correctly determining the CTFC is distinguishing the landmark of distal bifurcation (12). However, the identification of the landmark branch of LCX is somewhat vague. Therefore, the correlation in LCX was slightly weaker than that in LAD and RCA in the present study.
IMR serves as a specific approach for evaluating the microvascular function of interrogated vessels, which has been established as a surrogate parameter of CMD with an IMR >40 (20). Kotronias et al. (7) demonstrated IMR >40 to be an independent predictor of all-cause of mortality, resuscitated cardiac arrest, and new heart failure in patients with acute myocardial infarction (AMI). In our study, male sex, CTFC, and caFFR were independent factors for predicting CMD in patients with CSF. Although CMD would not be affected by epicardial arteries functionally evaluated by FFR, this study showed that caFFR may be a predictor for CMD. Moreover, despite all recruited coronary arteries being normal or near-normal, arteries with CMD might have had a lower degree of stenosis. Thus, after adjustments for sex, caFFR, and CTFC, the HR =of caFFR for predicting CMD was significantly decreased, with a boundary P value of 0.047.
The method to define CSF using CTFC was conducted by calculating the mean CTFC and 2-fold standard deviation in 78 normal arteries (12), but the threshold of CTFC in determining CMD is unclear. In this study’s ROC analysis, CTFC >38 frames (AUC =0.873; P<0.001), >35 frames (AUC =0.948; P<0.001), >52 frames (AUC =0.906; P<0.001), and >50 frames (AUC =0.968; P<0.001) were found to be the best cutoff values for predicting CMD for all arteries, the LAD, LCX, and RCA, respectively. This is the first study to determine the threshold of CTFC to define CMD and produce a superb AUC, which indicates that the accuracy of diagnosing CMD via CTFC is extremely high.
There were several limitations of the current study. First, this study’s retrospective design might have generated a potential selection bias. Second, some of the participants were excluded due to the quality of their coronary angiogram. Third, caIMR is evaluated using blood pressures and flows at rest, which might have biased results from invasive IMR using adenosine at hyperemia. Moreover, we failed to provide information on coronary flow reserve, which may more accurately reflect the function of epicardial and capillary coronary arteries. Lastly, even though caIMR is a novel noninvasive method for assessing coronary microcirculatory resistance, it cannot fully supersede wire-based IMR, indicating that further evaluation of the relationship between CTFC and IMR is needed.
Conclusions
CTFC correlated well with caIMR and had strong predictive power for identifying CMD.
Acknowledgments
We would like to thank Suzhou RainMed Medical Technology Co., Ltd. for supporting the computation of the caIMR.
Funding: This paper was funded by the Science Foundation of Gusu School (No. GSKY20220102), the Natural Science Foundation of Jiangsu Provincial (No. BK2012648 to HW), and the General Scientific Research Project of Jiangsu Provincial Health Commission (No. M2020083). The funding body played no role in the design, writing, or decision to publish this paper.
Footnote
Reporting Checklist: The authors have completed the STARD reporting checklist. Available at https://qims.amegroups.com/article/view/10.21037/qims-22-224/rc
Conflicts of Interest: All authors have completed the ICMJE uniform disclosure form (available at https://qims.amegroups.com/article/view/10.21037/qims-22-224/coif). The authors report that this research was funded by the Science Foundation of Gusu School (No. GSKY20220102), the Natural Science Foundation of Jiangsu Provincial (No. BK2012648 to HW), and the General Scientific Research Project of Jiangsu Provincial Health Commission (No. M2020083), with further support being provided by Suzhou RainMed Medical Technology Co., Ltd. The authors have no other conflicts of interest to declare.
Ethical Statement: The authors are accountable for all aspects of the work in ensuring that questions related to the accuracy or integrity of any part of the work are appropriately investigated and resolved. The study protocol was approved by the independent institutional ethics committee of the First Affiliated Hospital of Nanjing Medical University and complied with the Declaration of Helsinki (as revised in 2013). Written informed consent was acquired from all patients.
Open Access Statement: This is an Open Access article distributed in accordance with the Creative Commons Attribution-NonCommercial-NoDerivs 4.0 International License (CC BY-NC-ND 4.0), which permits the non-commercial replication and distribution of the article with the strict proviso that no changes or edits are made and the original work is properly cited (including links to both the formal publication through the relevant DOI and the license). See: https://creativecommons.org/licenses/by-nc-nd/4.0/.
References
- Turhan H, Yetkin E. The relation between insulin resistance and slow coronary flow: the development of microvascular dysfunction in insulin resistant state may be a plausible explanation. Int J Cardiol 2006;111:474-5. [Crossref] [PubMed]
- Li JJ, Wu YJ, Qin XW. Should slow coronary flow be considered as a coronary syndrome? Med Hypotheses 2006;66:953-6. [Crossref] [PubMed]
- Sanati H, Kiani R, Shakerian F, Firouzi A, Zahedme HR. =A, Peighambari M, Shokrian L, Ashrafi P. Coronary Slow Flow Phenomenon Clinical Findings and Predictors. Res Cardiovasc Med 2016;5:e30296. [Crossref] [PubMed]
- Wozakowska-Kapłon B, Niedziela J, Krzyzak P, Stec S. Clinical manifestations of slow coronary flow from acute coronary syndrome to serious arrhythmias. Cardiol J 2009;16:462-8. [PubMed]
- Saya S, Hennebry TA, Lozano P, Lazzara R, Schechter E. Coronary slow flow phenomenon and risk for sudden cardiac death due to ventricular arrhythmias: a case report and review of literature. Clin Cardiol 2008;31:352-5. [Crossref] [PubMed]
- Fearon WF, Balsam LB, Farouque HM, Caffarelli AD, Robbins RC, Fitzgerald PJ, Yock PG, Yeung AC. Novel index for invasively assessing the coronary microcirculation. Circulation 2003;107:3129-32. [Crossref] [PubMed]
- Kotronias RA, Terentes-Printzios D, Shanmuganathan M, Marin F, Scarsini R, Bradley-Watson J, Langrish JP, Lucking AJ, Choudhury R, Kharbanda RK, Garcia-Garcia HM, Channon KM, Banning AP, De Maria GL. Long-Term Clinical Outcomes in Patients With an Acute ST-Segment-Elevation Myocardial Infarction Stratified by Angiography-Derived Index of Microcirculatory Resistance. Front Cardiovasc Med 2021;8:717114. [Crossref] [PubMed]
- Ai H, Feng Y, Gong Y, Zheng B, Jin Q, Zhang HP, Sun F, Li J, Chen Y, Huo Y, Huo Y. Coronary Angiography-Derived Index of Microvascular Resistance. Front Physiol 2020;11:605356. [Crossref] [PubMed]
- Esmaeili Nadimi A, Pour Amiri F, Sheikh Fathollahi M, Hassanshahi G, Ahmadi Z, Sayadi AR. Opium addiction as an independent risk factor for coronary microvascular dysfunction: A case-control study of 250 consecutive patients with slow-flow angina. Int J Cardiol 2016;219:301-7. [Crossref] [PubMed]
- Beltrame JF. Defining the coronary slow flow phenomenon. Circ J 2012;76:818-20. [Crossref] [PubMed]
- Gibson CM, Ryan KA, Kelley M, Rizzo MJ, Mesley R, Murphy S, Swanson J, Marble SJ, Dodge JT, Giugliano RP, Cannon CP, Antman EM. Methodologic drift in the assessment of TIMI grade 3 flow and its implications with respect to the reporting of angiographic trial results. The TIMI Study Group. Am Heart J 1999;137:1179-84. [Crossref] [PubMed]
- Gibson CM, Cannon CP, Daley WL, Dodge JT Jr, Alexander B Jr, Marble SJ, McCabe CH, Raymond L, Fortin T, Poole WK, Braunwald E. TIMI frame count: a quantitative method of assessing coronary artery flow. Circulation 1996;93:879-88. [Crossref] [PubMed]
- Li J, Gong Y, Wang W, Yang Q, Liu B, Lu Y, Xu Y, Huo Y, Yi T, Liu J, Li Y, Xu S, Zhao L, Ali ZA, Huo Y. Accuracy of computational pressure-fluid dynamics applied to coronary angiography to derive fractional flow reserve: FLASH FFR. Cardiovasc Res 2020;116:1349-56. [Crossref] [PubMed]
- Wang X, Nie SP. The coronary slow flow phenomenon: characteristics, mechanisms and implications. Cardiovasc Diagn Ther 2011;1:37-43. [PubMed]
- Hawkins BM, Stavrakis S, Rousan TA, Abu-Fadel M, Schechter E. Coronary slow flow--prevalence and clinical correlations. Circ J 2012;76:936-42. [Crossref] [PubMed]
- Diver DJ, Bier JD, Ferreira PE, Sharaf BL, McCabe C, Thompson B, Chaitman B, Williams DO, Braunwald E. Clinical and arteriographic characterization of patients with unstable angina without critical coronary arterial narrowing (from the TIMI-IIIA Trial). Am J Cardiol 1994;74:531-7. [Crossref] [PubMed]
- Chalikias G, Tziakas D. Slow Coronary Flow: Pathophysiology, Clinical Implications, and Therapeutic Management. Angiology 2021;72:808-18. [Crossref] [PubMed]
- Mangieri E, Macchiarelli G, Ciavolella M, Barillà F, Avella A, Martinotti A, Dell'Italia LJ, Scibilia G, Motta P, Campa PP. Slow coronary flow: clinical and histopathological features in patients with otherwise normal epicardial coronary arteries. Cathet Cardiovasc Diagn 1996;37:375-81. [Crossref] [PubMed]
- Ma H, Dai X, Yang X, Zhao X, Wang R, Zhang J. Clinical and imaging predictors of impaired myocardial perfusion in symptomatic patients after percutaneous coronary intervention: insights from dynamic CT myocardial perfusion imaging. Quant Imaging Med Surg 2021;11:3327-37. [Crossref] [PubMed]
- Scarsini R, Shanmuganathan M, De Maria GL, Borlotti A, Kotronias RA, Burrage MK, Terentes-Printzios D, Langrish J, Lucking A, Fahrni G, Cuculi F, Ribichini F, Choudhury RP, Kharbanda R, Ferreira VM, Channon KM, Banning AP. OxAMI Study Investigators. Coronary Microvascular Dysfunction Assessed by Pressure Wire and CMR After STEMI Predicts Long-Term Outcomes. JACC Cardiovasc Imaging 2021;14:1948-59. [Crossref] [PubMed]