Influence of a single hemodialysis on left ventricular energy loss and wall shear stress in patients with uremic cardiomyopathy assessed with vector flow mapping
Introduction
Uremic cardiomyopathy is a myocardium-involved complication during the end stage of renal failure, eventually leading to left ventricular hypertrophy, dilation, and systolic and diastolic dysfunction (1-4). Multiple factors, including insufficient hemodialysis (HD), may induce uremic cardiomyopathy.
Echocardiography is a vital tool to comprehensively assess the cardiac function of patients with uremia. In studies on uremic cardiomyopathy, focus has been centered on ventricular wall movement. For example, Doppler ultrasound or speckle-tracking imaging (STI) have been adopted to analyze the left ventricular function and the underlying mechanisms (5-7). Research on left ventricular fluid mechanics, however, is scant. Nevertheless, intracardiac circulation, energy, and fluid mechanics are associated with ventricular function. Several reliable methods have been developed to visualize intracardiac flow (8), including cardiac magnetic resonance (CMR), echocardiographic particle image velocimetry (echo-PIV), and vector flow mapping (VFM). Among these methods, VFM is a novel ultrasound technology, which can easily and non-invasively visualize cardiovascular blood flow vector (8). Combining color Doppler ultrasound imaging, STI, and continuity equation, VFM can display intracardiac laminar flow and turbulence, thus reflecting the cardiovascular hydromechanics through stream and vorticity lines. Energy loss (EL) and wall shear stress (WSS) are two indexes employed by VFM. The EL represents the consumption of blood flow energy caused by many fractions of blood in the turbulent flow. It predicts both the ventricular load in various heart diseases and the spatial dispersion of the blood flow signal in the ventricular cavity, and it is known to be an index of fluid transport efficiency. Left ventricular EL is a new clinical index of cardiac dysfunction in patients with diabetes (9) and subclinical cardiac dysfunction in patients with hypertrophic cardiomyopathy (10). The EL has provided a new direction for heart research (11,12). The WSS is the stress of the blood flow near the ventricular wall acting on the endothelial wall. Measurement of WSS allows quantification and visualization of the mechanical stresses in the vascular and ventricular walls, making it an ideal index to reflect the pathophysiology of cardiovascular disease on a macroscopic scale. Quantitative assessment of left ventricular (LV) intraluminal blood flow from the perspective of EL and WSS provides insight into LV function. In this study, we used VFM to measure left ventricular EL and WSS in different phases and segments during a single HD in uremia patients, thus analyzing the left ventricular loads before and after HD from the perspective of hydrodynamics. We present the following article in accordance with the STROBE reporting checklist (available at https://qims.amegroups.com/article/view/10.21037/qims-21-1083/rc).
Methods
Participants
A total of 40 patients with end-stage renal disease (ESRD) receiving conventional HD in the Hemodialysis Unit of the First Affiliated Hospital of Nanjing Medical University between January 2018 and January 2019 were prospectively recruited to this study, as previously described (9). The inclusion criteria were as follows: (I) ESRD (II) sinus rhythm, (III) HD for a minimum of 1 month and 2–3 times per week, and (IV) provision of informed consent. Patients with acute myocardial infarction, systolic heart failure, primary cardiomyopathy, obvious valve lesions (more severe than mild valve stenosis or insufficiency), pulmonary embolism, acute infection, pericardial disease, or atrial fibrillation were excluded from the study. The study was conducted in accordance with the Declaration of Helsinki (as revised in 2013). The study was approved by the Institutional Review Board of Jiangsu Province Hospital, and informed consent was provided by all individual participants.
Echocardiography
All patients were evaluated with echocardiography twice (before and within 24 h after HD). The data were self-controlled before and after a single HD (Figure 1).
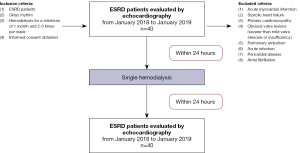
Color Doppler ultrasound was performed using the Hitachi Aloka Prosound F75 ultrasound machine (Hitachi Medical Corp., Tokyo, Japan) with a UST-52105 probe and a 1.0–5.0 MHz frequency. Echocardiography was performed by an experienced senior physician. Briefly, the patient was placed in the left lateral position and asked to breathe calmly. Left ventricular and left atrial volume, Doppler ultrasound images of blood flow and cardiac tissues, and two-dimensional (2D) gray scale images in the views across the left ventricular apical four-chamber, two-chamber, and longitudinal axis, and in the parasternal basal, middle, and apical segments of the left ventricle were obtained within three consecutive cardiac cycles at >50 frames per second. The left ventricular ejection fraction (LVEF) at the apical four-chamber and two-chamber longitudinal planes was measured using the biplane Simpson method. The blood flow at the tip of the mitral valve leaflets was sampled to measure the peak flow velocity at the mitral valve orifice in the early (E wave) and late diastolic phases (A wave). In addition, the early diastolic velocity at the septal (e’septal) or lateral mitral valve annulus (e’lateral), and their average values (e’ average) were calculated at the corresponding sampling locations. Then, E/A and E/e′ were calculated based on the above indexes.
Blood flow images at the apical long-axis, four-chamber, and two-chamber were obtained within three consecutive cardiac cycles at a frame frequency higher than 18 Hz by VFM. The image was required to cover the entire left ventricle. The VFM images were stored in the DICOM file for off-line analysis.
EL and WSS measured using VFM
The 2D dynamic color Doppler frames were analyzed using commercially available VFM analysis software (DAS-RS1, Hitachi Aloka Medical Ltd., Tokyo, Japan). A clear frame was frozen to manually trace the endocardial boundary, and the region of interest (ROI) was determined after the whole left ventricle had been defined. The 2D frame displayed quantitative images (e.g., velocity vector, stream line, vorticity, and vorticity line) and quantitative indexes (e.g., circulation, EL, and WSS). Combined with two sample lines equally dividing the left ventricle, electrocardiogram findings, valve opening, and time-flow curve, the following five phases during the cardiac cycle were determined: the early diastolic phase (T1), mid-diastolic phase (T2), late diastolic phase (T3), early systolic phase (T4), and late systolic phase (T5) (Figure 2). The summation of energy loss (EL-SUM) and average energy loss (EL-AVE) in the ROIs were calculated. The EL-AVE indicated the energy loss per 1 m2. In addition, EL-AVE in the basal (EL-base), middle (EL-mid), and apical (EL-apex) segments of the left ventricle were calculated (Figure 3). The WSS of the left ventricle at each frame was calculated using DAS-RS1 software (Figure 4). The WSS values at three segments in each phase were averaged as the overall left ventricular WSS.
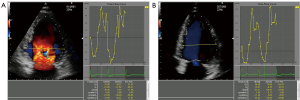
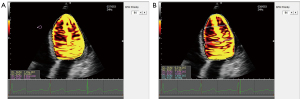
Statistical analysis
Statistical analysis was performed using the software SPSS 22.0 (IBM Corp., Armonk, NY, USA).
Continuous variables were expressed as mean ± standard deviation or median (interquartile range) and categorical data as the percentage or frequency. Continuous normally distributed data before and after HD were compared using the Student’s t-test, and the Mann-Whitney U test was used for abnormally distributed data. P value <0.05 was considered statistically significant.
Results
Baseline characteristics
A total of 40 uremia patients receiving conventional HD were recruited to this study, including 27 males and 13 females, with a mean age of 51.0±16.4 years and a HD course of 4.31±4.18 years. Their baseline characteristics are listed in Table 1, as reported in our previous study (13). The cause of ESRD was chronic glomerulonephritis, polycystic kidney, hypertension, and diabetes mellitus in 40%, 10%, 10%, and 7.5% of patients, respectively, and the remaining participants reported unknown causes. Systolic blood pressure, diastolic blood pressure, and body weight were significantly reduced after HD, compared with those measured before HD, although no significant difference was detected in heart rate (Table 2), as reported in our previous study (13).
Table 1
Variables | Value |
---|---|
Age (year), mean ± standard deviation | 51.0±16.4 |
Gender (male, %) | 67.5 |
Hemodialysis duration (year), mean ± standard deviation | 4.3±4.2 |
Smoking history (%) | 10 |
Body surface area (m2), mean ± standard deviation | 1.7±0.2 |
Cause of end-stage renal disease | |
Diabetes mellitus (%) | 7.5 |
Hypertension (%) | 10 |
Chronic glomerulonephritis (%) | 40 |
Polycystic kidney (%) | 10 |
Others or unknown causes (%) | 32.5 |
Medication | |
ACEI/ARB (%) | 18.3 |
Betaloc (%) | 21.7 |
Calcium channel blocker (%) | 40 |
Statins (%) | 1.7 |
ACEI/ARB, angiotensin-converting enzyme inhibitors/angiotensin receptor blockers.
Table 2
Variables | Before HD, mean ± standard deviation | After HD, mean ± standard deviation | P value |
---|---|---|---|
Systolic pressure (mmHg) | 139.40±14.81 | 126.09±23.95 | 0.002** |
Diastolic pressure (mmHg) | 82.60±10.62 | 77.03±9.53 | 0.002** |
Heart rate (beats/min) | 74.88±9.74 | 74.60±9.37 | 0.839 |
Body weight (kg) | 64.56±14.18 | 61.92±14.05 | <0.001*** |
**P<0.01, ***P<0.001 vs. clinical data before hemodialysis. HD, hemodialysis.
Ultrasound parameters of the left ventricle before and after HD
There was no significant difference in the left ventricular end-systolic volume (LVESV) before and after HD, but the left ventricular end-diastolic volume (LVEDV, 90.18±23.91 vs. 84.21±23.54 mL, P=0.036) and LVEF (64.63%±6.56% vs. 62.84%±6.56%, P=0.049) were significantly reduced after HD. The E/A was significantly reduced (0.90±0.27 vs. 0.79±0.23, P<0.001) after HD. The E/e′, which reflects the left ventricular end-diastolic filling pressure, was significantly reduced after HD (12.54±4.08 vs. 11.28±4.52, P=0.049) (Table 3), as reported in our previous study (13).
Table 3
Variables | Before HD, mean ± standard deviation | After HD, mean ± standard deviation | P value |
---|---|---|---|
LVEDV (mL) | 90.18±23.91 | 84.21±23.54 | 0.036* |
LVESV (mL) | 32.84±12.47 | 32.08±12.47 | 0.585 |
LVEF (%) | 64.63±6.56 | 62.84±6.56 | 0.049* |
E/A | 0.90±0.27 | 0.79±0.23 | <0.001*** |
E/e′ | 12.54±4.08 | 11.28±4.52 | 0.049 |
*P<0.05, ***P<0.001 vs. clinical data before hemodialysis. HD, hemodialysis; LVESV, left ventricular end-systolic volume; LVEDV, left ventricular end-diastolic volume; LVEF, left ventricular ejection fraction; E wave, the peak flow velocity at the mitral valve orifice in the early diastole; A wave, the peak flow velocity at the mitral valve orifice in the late diastole; E/e′, the average value of the E wave separately divided by the peak myocardial velocities at the septal mitral annulus and lateral mitral annulus.
EL of the left ventricle
The EL-AVE and EL-SUM of the left ventricle before and after hemodialysis at T1-T5 are listed in Tables 4,5. Briefly, EL-SUM and EL-AVE at T1, T2, and T4 after HD were significantly lower those before HD, although no significant differences were detected in those at T3 and T5. In addition, a significant reduction in EL-AVE at T1 was observed mainly in EL-base [50.70 (24.19 to 77.92) vs. 26.00 (11.50 to 47.68) N/(m2·s), P<0.001], EL-mid [15.52 (8.88 to 20.90) vs. 9.47 (6.41 to 14.21) N/(m2·s), P=0.001]; in EL-base [18.64 (10.33 to 29.80) vs. 10.25 (6.98 to 19.43) N/(m2·s), P<0.001], EL-mid [15.70 (9.93 to 23.08) vs. 9.99 (6.03 to 16.25) N/(m2·s), P<0.001], and EL-apex [9.78 (4.06 to 15.77) vs. 4.52 (3.14 to 10.36) N/(m2·s), P=0.001] at T2, and in EL-mid [14.34 (8.34 to 23.88) vs. 9.36 (6.48 to 17.05) N/(m2·s), P=0.013] and EL-apex [11.25 (6.37 to 21.88) vs. 6.60 (5.33 to 12.17) N/(m2·s), P=0.016] at T3. At T4, EL-AVE was significantly reduced in the EL-apex [10.28 (6.05 to 17.01) vs. 7.59 (3.73 to 13.20) N/(m2·s), P=0.025] (Figure 5).
Table 4
Variables | Before hemodialysis [N/(m2·s)], median (interquartile range) | After hemodialysis [N/(m2·s)], median (interquartile range) | P value |
---|---|---|---|
T1 | 29.43 (18.76–46.28) | 17.70 (10.76–95.60) | <0.001*** |
T2 | 17.07 (10.38–24.35) | 10.29 (5.86–16.30) | <0.001*** |
T3 | 28.77 (15.75–43.92) | 23.45 (14.87–35.50) | 0.368 |
T4 | 17.82 (12.79–24.77) | 14.90 (10.23–19.05) | 0.011* |
T5 | 12.96 (10.23–20.09) | 12.16 (9.65–28.63) | 0.265 |
*P<0.05, ***P<0.001 vs. clinical data before hemodialysis. HD, hemodialysis; T1, early-diastolic phase; T2, mid-diastolic phase; T3, late-diastolic phase; T4, early-systolic phase; T5, late-systolic phase.
Table 5
Variables | Before hemodialysis (e−2 J), median (interquartile range) | After hemodialysis (e−2 J), median (interquartile range) | P value |
---|---|---|---|
T1 | 12 [6–17] | 5 [3–11] | <0.001*** |
T2 | 7 [3–10] | 4 [2–6] | <0.001*** |
T3 | 11 [7–18] | 8 [6–13] | 0.094 |
T4 | 8 [5–11] | 5 [4–8] | 0.002** |
T5 | 6 [3–8] | 5 [3–8] | 0.931 |
**P<0.01, ***P<0.001 vs. clinical data before hemodialysis. HD, hemodialysis; T1, early-diastolic phase; T2, mid-diastolic phase; T3, late-diastolic phase; T4, early-systolic phase; T5, late-systolic phase.
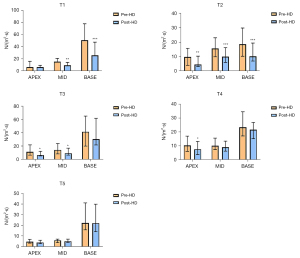
WSS of the left ventricle
Compared with before HD, WSS at T2 [0.51 (0.32 to 0.69) vs. 0.38 (0.30 to 0.46) Pa, P=0.001] and T4 [0.60 (0.45 to 0.81) vs. 0.57 (0.42 to 0.68) Pa, P=0.029] were significantly reduced after HD, and the values before and after HD at T1, T3, and T5 were comparable (Table 6).
Table 6
Variables | Before hemodialysis (Pa) | After hemodialysis (Pa) | P value |
---|---|---|---|
T1 | 0.45 (0.38–0.57) | 0.44 (0.36–0.56) | 0.227 |
T2 | 0.51 (0.32–0.69) | 0.38 (0.30–0.46) | 0.001* |
T3 | 0.54±0.22 | 0.53±0.20 | 0.676 |
T4 | 0.60 (0.45–0.81) | 0.57 (0.42–0.68) | 0.029* |
T5 | 0.49 (0.36–0.67) | 0.47 (0.38–0.60) | 0.439 |
Data were expressed as mean ± standard deviation or median (interquartile range). *P<0.05 vs. clinical data before hemodialysis. WSS, wall shear stress; HD, hemodialysis; T1, early-diastolic phase; T2, mid-diastolic phase; T3, late-diastolic phase; T4, early-systolic phase; T5, late-systolic phase.
Discussion
Hemodynamic abnormalities are a vital cause of uremic cardiomyopathy. Using information extracted from Doppler signals, VFM can dynamically visualize the cardiac blood flow. To date, the influence of HD on hydromechanics of the left ventricle has not been reported. In the present study, we compared the left ventricular EL and WSS before and after a single HD in patients with uremia. The results showed that EL changed evidently during the early- and mid-diastolic phases and the early systolic phase, but almost returned to normal during the late diastolic and systolic phases. Moreover, WSS was reduced during the mid-diastolic and early systolic phases but remained unchanged during the early and late diastolic phases and the late systolic phase.
Ventricular EL is an additional energy loss caused by the friction of the blood flow in the left ventricle. A previous study reported left ventricular EL changes in several pathophysiological conditions (14). For example, EL during the diastolic and systolic phases is significantly higher in patients with ESRD than in controls and significantly lower during the diastolic phase in patients receiving peritoneal dialysis than that in those who have been prepared for HD. Moreover, the left ventricular EL during the diastolic and partial systolic phases is higher in patients with diabetes mellitus than in controls (15). Ischemic cardiomyopathy, left ventricular desynchronization, and mitral valve replacement surgery can deform the left ventricular vortex and increase left ventricle EL (16-18).
Our findings show that left ventricular EL-AVE and EL-SUM were reduced during the early diastolic phase in patients with ESRD after HD. The reason may be that the left ventricular preload is significantly reduced after HD, and the decreased blood volume in the left ventricle further reduces the friction in blood flow vector, thus leading to EL. Myocardial stunning could be another cause. It has been reported that HD-induced myocardial stunning causes hypotension during dialysis in 20–30% of patients (19). Myocardial stunning impairs systolic function of the left ventricle. Agati et al. (20) demonstrated that the energy dissipation index is positively correlated with both LVEF and global longitudinal strain (GLS) in patients with acute myocardial infarction with left ventricular dysfunction assessed by echo particle image velocimetry (Echo-PIV).
Akiyama et al. (21) reported a strong clockwise vortex under the anterior mitral leaflet and a weak counterclockwise vortex under the posterior mitral leaflet during the early diastolic phase in healthy people. The former gradually increases during the mid-diastolic phase to occupy the middle and basal segments of the left ventricle, while the latter gradually disappears. In addition, they found that the left ventricular EL during the diastolic phase was positively correlated with the E wave. Vortex momentum facilitates the ejection flow during the early systolic phase, in which the blood flow is accelerated and pumped from the left ventricular cavity into the left ventricular outflow tract, forming the peak systolic EL. During the late systolic phase, vortex momentum disappears, and the blood flow turns to the left ventricular outflow tract. In our study, EL remained consistently the highest during the early and mid-diastolic and the early systolic phases. Our research team showed that preload influences diastolic function mainly during early diastole (13). During this phase, most of the extra preload before HD is transferred into the left ventricle. Therefore, in the present study, EL during the late diastolic phase remained unchanged. In addition, the changes in EL before and after HD were observed mainly in the apex and mid segments of the left ventricle, which might be associated with the changes in cardiovascular hydromechanics during a single HD.
Measurement of WSS is commonly used to assess the stress of blood flow on the vascular wall (22) but rarely on the ventricular wall. Ji et al. (23) found that in patients with hypertrophic cardiomyopathy, the left ventricular WSS increases during rapid ejection and atrial systole and slightly decreases during the end of early diastole. Meng et al. (24) suggested that WSS decreases during the rapid filling phase of the left ventricle, atrial systolic phase, isovolumic contraction phase, and rapid ejection phase in patients with right ventricular septal pacing. In the present study, the left ventricular WSS significantly decreased during the mid-diastolic and early systolic phases. The WSS is calculated as follows: WSS = μ(dv/dy), where μ represents the blood flow viscosity coefficient, and dv/dy represents the shear rate. According to the above formula, WSS increases with intraventricular blood flow velocity. As body weight and fluid load decrease after hemodialysis, the intraventricular blood flow velocity drops, manifested by the low overall left ventricle WSS during the mid-diastolic phase and early systolic phases. We suggest that the left ventricle consumes more ineffective energy during the mid-diastole phase and early systolic phases with fluid overload.
Study limitations
Some limitations of this study should be noted. First, it was a preliminary study with a small sample size, and more cases are needed to eliminate potential biases. Second, we only analyzed 2D cardiac blood flow, which, in fact, is three-dimensional (3D). Therefore, 3D assessment of cardiac hydrodynamic parameters is needed in the future. Third, the Nyquist limit of 2D color Doppler imaging may influence the assessment of flow dynamic parameters. When the flow velocity is twice as high compared with the Nyquist limit, the flow dynamic parameters would not be accurate. Fourth, in this study we evaluated the changes in EL and WSS in patients before and after a single dialysis, and we need further analysis of the clinical implications of altered EL and WSS during the cardiac cycle in patients with uremic cardiomyopathy.
Conclusions
The EL and WSS during the partial systolic and diastolic phases before HD are significantly higher than those after HD in ESRD patients with cardiac overload. Assessed by VFM, both indexes are conducive to assess the left ventricular hydrodynamic state of patients receiving HD with varying liquid loads.
Acknowledgments
We thank the authors of the primary studies for their timely and helpful responses to our requests for information.
Funding: The present study was supported by the National Natural Science Foundation of China (No. 81871359) and the Natural Science Foundation of Jiangsu Province (No. BK20191496).
Footnote
Reporting Checklist: The authors have completed the STROBE reporting checklist. Available at https://qims.amegroups.com/article/view/10.21037/qims-21-1083/rc
Conflicts of Interest: All authors have completed the ICMJE uniform disclosure form (available at https://qims.amegroups.com/article/view/10.21037/qims-21-1083/coif). The authors have no conflicts of interest to declare.
Ethical Statement: The authors are accountable for all aspects of the work in ensuring that questions related to the accuracy or integrity of any part of the work are appropriately investigated and resolved. The study was conducted in accordance with the Declaration of Helsinki (as revised in 2013). The study was approved by Institutional Review Board of Jiangsu Province Hospital, and informed consent was provided by all individual participants.
Open Access Statement: This is an Open Access article distributed in accordance with the Creative Commons Attribution-NonCommercial-NoDerivs 4.0 International License (CC BY-NC-ND 4.0), which permits the non-commercial replication and distribution of the article with the strict proviso that no changes or edits are made and the original work is properly cited (including links to both the formal publication through the relevant DOI and the license). See: https://creativecommons.org/licenses/by-nc-nd/4.0/.
References
- McIntyre CW, Odudu A, Eldehni MT. Cardiac assessment in chronic kidney disease. Curr Opin Nephrol Hypertens 2009;18:501-6. [Crossref] [PubMed]
- Cerasola G, Nardi E, Palermo A, Mulè G, Cottone S. Epidemiology and pathophysiology of left ventricular abnormalities in chronic kidney disease: a review. J Nephrol 2011;24:1-10. [Crossref] [PubMed]
- Whalley GA, Marwick TH, Doughty RN, Cooper BA, Johnson DW, Pilmore A, Harris DC, Pollock CA, Collins JFIDEAL Echo Substudy Investigators. Effect of early initiation of dialysis on cardiac structure and function: results from the echo substudy of the IDEAL trial. Am J Kidney Dis 2013;61:262-70. [Crossref] [PubMed]
- Go AS, Chertow GM, Fan D, McCulloch CE, Hsu CY. Chronic kidney disease and the risks of death, cardiovascular events, and hospitalization. N Engl J Med 2004;351:1296-305. [Crossref] [PubMed]
- Liu F, Wang X, Liu D, Zhang C. Frequency and risk factors of impaired left ventricular global longitudinal strain in patients with end-stage renal disease: a two-dimensional speckle-tracking echocardiographic study. Quant Imaging Med Surg 2021;11:2397-405. [Crossref] [PubMed]
- Ünlü S, Şahinarslan A, Gökalp G, Seçkin Ö, Arınsoy ST, Boyacı NB, Çengel A. The impact of volume overload on right heart function in end-stage renal disease patients on hemodialysis. Echocardiography 2018;35:314-21. [Crossref] [PubMed]
- Wang Y, Hou D, Ma R, Ding G, Yin L, Zhang M. Early Detection of Left Atrial Energy Loss and Mechanics Abnormalities in Diabetic Patients with Normal Left Atrial Size: A Study Combining Vector Flow Mapping and Tissue Tracking Echocardiography. Med Sci Monit 2016;22:958-68. [Crossref] [PubMed]
- Rodriguez Muñoz D, Markl M, Moya Mur JL, Barker A, Fernández-Golfín C, Lancellotti P, Zamorano Gómez JL. Intracardiac flow visualization: current status and future directions. Eur Heart J Cardiovasc Imaging 2013;14:1029-38. [Crossref] [PubMed]
- Li CM, Bai WJ, Liu YT, Tang H, Rao L. Dissipative energy loss within the left ventricle detected by vector flow mapping in diabetic patients with controlled and uncontrolled blood glucose levels. Int J Cardiovasc Imaging 2017;33:1151-8. [Crossref] [PubMed]
- Cao Y, Sun XY, Zhong M, Li L, Zhang M, Lin MJ, Zhang YK, Jiang GH, Zhang W, Shang YY. Evaluation of hemodynamics in patients with hypertrophic cardiomyopathy by vector flow mapping: Comparison with healthy subjects. Exp Ther Med 2019;17:4379-88. [Crossref] [PubMed]
- Yoshida S, Miyagawa S, Fukushima S, Yoshikawa Y, Hata H, Saito S, Yoshioka D, Kainuma S, Domae K, Matsuura R, Nakatani S, Toda K, Sawa Y. Cardiac Function and Type of Mitral Valve Surgery Affect Postoperative Blood Flow Pattern in the Left Ventricle. Circ J 2018;83:130-8. [Crossref] [PubMed]
- Nakashima K, Itatani K, Kitamura T, Oka N, Horai T, Miyazaki S, Nie M, Miyaji K. Energy dynamics of the intraventricular vortex after mitral valve surgery. Heart Vessels 2017;32:1123-9. [Crossref] [PubMed]
- Wang X, Hong J, Zhang T, Xu D. Changes in left ventricular and atrial mechanics and function after dialysis in patients with end-stage renal disease. Quant Imaging Med Surg 2021;11:1899-908. [Crossref] [PubMed]
- Zhong Y, Liu Y, Wu T, Song H, Chen Z, Zhu W, Cai Y, Zhang W, Bai W, Tang H, Rao L. Assessment of Left Ventricular Dissipative Energy Loss by Vector Flow Mapping in Patients With End-Stage Renal Disease. J Ultrasound Med 2016;35:965-73. [Crossref] [PubMed]
- Wang Y, Ma R, Ding G, Hou D, Li Z, Yin L, Zhang M. Left Ventricular Energy Loss Assessed by Vector Flow Mapping in Patients with Prediabetes and Type 2 Diabetes Mellitus. Ultrasound Med Biol 2016;42:1730-40. [Crossref] [PubMed]
- Pedrizzetti G, Domenichini F, Tonti G. On the left ventricular vortex reversal after mitral valve replacement. Ann Biomed Eng 2010;38:769-73. [Crossref] [PubMed]
- Rodríguez Muñoz D, Moya Mur JL, Lozano Granero C, Fernández-Golfín C, Zamorano Gómez JL. Flow collision in early aortic ejection: an additional source of kinetic energy loss in patients with mitral prosthetic valves. Eur Heart J Cardiovasc Imaging 2015;16:608. [Crossref] [PubMed]
- Kakizaki R, Nabeta T, Ishii S, Koitabashi T, Itatani K, Inomata T, Ako J. Cardiac resynchronization therapy reduces left ventricular energy loss. Int J Cardiol 2016;221:546-8. [Crossref] [PubMed]
- Breidthardt T, McIntyre CW. Dialysis-induced myocardial stunning: the other side of the cardiorenal syndrome. Rev Cardiovasc Med 2011;12:13-20. [Crossref] [PubMed]
- Agati L, Cimino S, Tonti G, Cicogna F, Petronilli V, De Luca L, Iacoboni C, Pedrizzetti G. Quantitative analysis of intraventricular blood flow dynamics by echocardiographic particle image velocimetry in patients with acute myocardial infarction at different stages of left ventricular dysfunction. Eur Heart J Cardiovasc Imaging 2014;15:1203-12. [Crossref] [PubMed]
- Akiyama K, Maeda S, Matsuyama T, Kainuma A, Ishii M, Naito Y, Kinoshita M, Hamaoka S, Kato H, Nakajima Y, Nakamura N, Itatani K, Sawa T. Vector flow mapping analysis of left ventricular energetic performance in healthy adult volunteers. BMC Cardiovasc Disord 2017;17:21. [Crossref] [PubMed]
- Saito K, Abe S, Kumamoto M, Uchihara Y, Tanaka A, Sugie K, Ihara M, Koga M, Yamagami H. Blood Flow Visualization and Wall Shear Stress Measurement of Carotid Arteries Using Vascular Vector Flow Mapping. Ultrasound Med Biol 2020;46:2692-9. [Crossref] [PubMed]
- Ji L, Hu W, Yong Y, Wu H, Zhou L, Xu D. Left ventricular energy loss and wall shear stress assessed by vector flow mapping in patients with hypertrophic cardiomyopathy. Int J Cardiovasc Imaging 2018;34:1383-91. [Crossref] [PubMed]
- Meng Q, Wang S, Yan S, Xu Y, Wu Z, Zhou J, Guo Z, Yin L, Deng Y, Feng T. Evaluating the left ventricular hemodynamic phenomena of DDD septum pacemaker implants using vector flow mapping. Echocardiography 2020;37:77-85. [Crossref] [PubMed]