Evaluation of infarct core and ischemic penumbra by absolute quantitative cerebral dynamic susceptibility contrast perfusion magnetic resonance imaging using self-calibrated echo planar imaging sequencing in patients with acute ischemic stroke
Introduction
According to World Health Organization (WHO) criteria, stroke is defined as “rapidly developed clinical signs of focal (or global) disturbance of cerebral function, lasting more than 24 hours or leading to death, with no apparent cause other than of vascular origin”, including subarachnoid hemorrhage, intracranial hemorrhage, and cerebral infarction (1). Globally, stroke is one of the leading causes of mortality and disability. With the aging of the Chinese population, stroke has become the leading cause of mortality in China (2). About 85% of stroke in China is attributed to ischemic stroke (IS), defined as all atherosclerotic and thromboembolic events resulting in compromised blood flow to brain tissue and subsequent infarction (3). The absolute number of people with IS is predicted to increase annually until more effective preventive strategies, diagnostic protocols, and treatment programs are widely implemented.
The recommendations of the American Heart Association/American Stroke Association (AHA/ASA) have sparked new interest in tissue-based selection criteria to allow for a greater number of stroke patients to be safely treated (4). With the progress of medical imaging, there is an opportunity to develop a tissue-based imaging protocol for IS patients. Magnetic resonance (MR) diffusion-weighted imaging (DWI) is the most sensitive diagnostic imaging modality for early detection of IS, although DWI can occasionally be negative (approximately 8%) in IS (5). Dynamic susceptibility contrast (DSC) perfusion-weighted imaging (PWI) is known to be a valid tool for reflecting hemodynamic information by providing semi-quantitative parameters. The combination of DWI and PWI could offer a unique insight into IS pathophysiology and improve the sensitivity for IS diagnosis (to approximately 97.5%) (5,6). In the clinical setting, it is relatively straightforward to visually assess deficits, while it is difficult to quantify perfusion parameters and avoid subjective bias. Therefore, an absolute quantitative DSC PWI is needed to evaluate cerebral ischemic lesions and guide treatment.
An absolute quantitative DSC PWI technique, the multiscan Bookend technique, which is feasible in IS patients (7), has been reported previously. This technique has the potential to produce reproducible, accurate, and reliable quantitative perfusion metrics in patient studies, but it needs complex scanning steps and additional offline postprocessing time to obtain the absolute quantitative perfusion images (7-11). To solve this problem, a new magnetic resonance imaging (MRI) sequence was recently presented for quantitative cerebral perfusion imaging: the Self-CALibrated echo-planar imaging (EPI) Perfusion-Weighted Imaging (SCALE-PWI), eliminates the multiple steps in the Bookend mutliscan process and implements automatic, online image postprocessing (11). The quantitative cerebral blood flow (qCBF) images and quantitative cerebral blood volume (qCBV) images derived from SCALE-PWI require a total time of <3 minutes, potentially saving valuable time in clinical settings. Therefore, SCALE-PWI could be used in emergency settings, such as acute ischemic stroke (AIS), to obtain an immediate and accurate diagnosis.
This study aimed to investigate the clinical value of qCBF and qCBV derived from SCALE-PWI in distinguishing ischemic tissue from normal perfused tissue in AIS patients. We hypothesized that the qCBF and qCBV may be potential imaging biomarkers which could offer a unique insight into AIS pathophysiology, reflect the severity of AIS, and help to achieve the goal of tissue-based decision-making and personalized AIS treatment (12). We present the following article in accordance with the STARD reporting checklist (available at https://qims.amegroups.com/article/view/10.21037/qims-21-975/rc).
Methods
Participants
From September 2017 to June 2020, inpatients with AIS in the Department of Neurology of Zhengzhou University People’s Hospital were prospectively recruited as a consecutive convenience sample for this study. We confirmed the diagnosis of AIS all patients by MRI as MRI was considered the current gold standard imaging modality to diagnose IS (13).
The inclusion criteria for patients were as follows: (I) clinically stable, 18–80 years old; (II) hyperintensity on DWI and hypointensity on apparent diffusion coefficient (ADC) maps without obvious chronic lesions, suggesting a recent AIS; (III) lesions in the middle cerebral artery (MCA) white matter (WM) territory of unilateral moderate or severe MCA occlusion (stenosis: >50%), confirmed by computed tomography angiography (CTA) and/or magnetic resonance angiography (MRA); (IV) time from symptom onset to SCALE-PWI scanning within 28 days was selected as the cutoff between acute and chronic stroke (3,14); (V) recording of the National Institutes of Health Stroke Scale (NIHSS) scores on the day of SCALE-PWI scanning; (VI) recording of the Modified Rankin Scale (mRS) scores on the 90th day after symptom onset; and (VII) standard medical therapy received except for reperfusion therapy, which would alter local perfusion within the region affected by IS.
The exclusion criteria for patients were as follows: (I) DWI with only cerebral lacunar infarction; (II) preexisting neurological diseases; (III) hemorrhagic transformation and other complication; (IV) motion artifacts leading to poor imaging quality; (V) contraindications or severe claustrophobia; and (VI) incomplete patient data. This study was conducted in accordance with the Declaration of Helsinki (as revised in 2013). This study was approved by the institutional review board of Zhengzhou University People’s Hospital (No. 201967) and informed consent was provided by all the participants or their family members before participation.
Data acquisition
All participants underwent MRI scanning and clinical assessments. The NIHSS scores were evaluated and recorded by 2 neurologists independently on the day of the SCALE-PWI scanning and the mRS scores on the 90th day after symptom onset. For the NIHSS scores that were collected to determine stroke severity, a score of up to 4 points was considered a minor stroke, 5 to 15 points a moderate stroke, 16 to 20 points a severe stroke, and 21 points or over a very severe stroke (15). For the mRS scores that were used to assess stroke disability, the score was divided between 0 to 2 (mild disability) and 3 to 5 (moderate to severe disability) (15). All assessors were blinded to imaging findings.
The MRI protocols included conventional MRI, MRA, susceptibility-weighted imaging (SWI), and SCALE-PWI using the MAGNETOM Prisma 3T MR scanner (Siemens Healthcare, Erlangen, Germany) equipped with a 64-channel head-neck coil. The conventional MRI included an axial T1-weighted imaging (T1WI), an axial T2-weighted imaging (T2WI), an axial T2-weighted dark-fluid image, and a sagittal T1WI.
The parameters of the axial T1WI were as follows: repetition time/time to echo (TR/TE) =400/2.46 ms, matrix size =320×224, flip angle =70º, field of view (FOV) =230×230 mm2, slice thickness =6 mm, and acquisition time =0:50 minutes.
The parameters of the T2WI were as follows: TR/TE =4,600/97 ms, matrix size =320×224, flip angle =150º, FOV =230×230 mm2, slice thickness =6 mm, and acquisition time =1:04 minutes.
The parameters of the T2-weighted dark-fluid image were as follows: TR/TE =8,440/108 ms, matrix size =320×224, flip angle =120º, FOV =240×240 mm2, slice thickness =6 mm, and acquisition time =1:58 minutes.
The parameters of the sagittal T1WI were as follows: TR/TE =200/2.46 ms, matrix size =320×224, flip angle =70º, FOV =240×240 mm2, slice thickness =6 mm, and acquisition time =0:54 minutes.
The parameters of the DWI were as follows: TR/TE =2,400/54 ms, matrix size =160×160, flip angle =180º, FOV =230×230 mm2, slice thickness =6 mm, b-value =0 and 1,000 s/mm2, 3 directions and acquisition time =1:05 minutes.
The parameters of the MRA were as follows: TR/TE =21/3.45 ms, matrix size =320×256, flip angle =18º, FOV =200×180 mm2, slice thickness =0.6 mm, and acquisition time =3:36 minutes.
The parameters of the SWI were as follows: TR/TE =26/17.5 ms, matrix size =512×256, flip angle =15º, FOV =220×199 mm2, slice thickness =2 mm, and acquisition time =3:07 minutes.
The SCALE-PWI consisted of 3 modules: the pre- and post-contrast single-shot inversion recovery Look-Locker echo planar imaging (IR LL EPI) for T1 mapping of a single calibration slice, which made up the calibration module, and a multislice single-shot gradient echo (GRE) EPI DSC PWI. The GRE EPI DSC PWI could potentially produce a conventional PWI as the reference standard. In addition, a plus T1 sequence was necessary for determining the best slice position for the calibration module, which had the same resolution and coverage of the SACLE-PWI sequence. This slice was the first slice just above the ventricle, potentially giving the greatest amount of WM and consequently the most reliable values. The parameters of the T1WI and the prototype SCALE-PWI were identical: TR/TE =1,600/30 ms, matrix size =128×128, flip angle =90º, FOV =220×220 mm2, slice thickness =6 mm, and the total time =2:22 minutes. A single dose (0.1 mmol/kg body weight) of gadolinium diethylenetriamine penta-acetic acid (Gd-DTPA) contrast agent (Magnevist, Berlex Laboratories, Montville, NJ, USA) was automatically injected by a power injector with a delay of 46 seconds after SCALE-PWI scanning, followed by a 20 mL saline flush at a rate of 4 mL/second.
Image postprocessing
The SCALE-PWI could automatically produce the quantitative maps (qCBF map and qCBV map) online immediately after the scan, based on the premise that relative perfusion images can be quantified if a proper calibration can be determined (7,11). The calibration module was used to calculate T1 changes and calibrate the DSC module for quantitative perfusion (4). The quantitative CBV value in the WM (qCBVWM) was calculated from the pre- and post-contrast T1 changes in the WM and the blood, relying on the effects of intravascular to extravascular water exchange. The sagittal sinus was used for measuring the T1 value of blood. The regions of interests (ROIs) of WM and blood were automatically identified on the T1 maps, while the slices of T1 maps were manually determined before SCALE-PWI scanning. The DSC module was processed with a standard deconvolution approach, and the final perfusion quantification in a brain voxel was calculated as Eq. [1] and Eq. [2] (11), as follows:
Note: rCBV, relative CBV value; rCBF, relative CBF value; rCBVWM, relative CBV value in the white matter; qCBVWM, quantitative CBV value in the white matter.
MRI data analysis
The MRI data were transferred to the workstation (Syngo Via VB10; Siemens Healthcare Solutions, Erlangen, Germany) for analyses. The 2 radiologists (XYM and YW) independently analyzed all the MRI data and were blinded to the clinical data. First, they reviewed the MR images to confirm the diagnosis and lesion position. Second, they respectively chose 1 slice with a maximum lesion on DWI for each patient. The slices of other images were positioned at the same slice as the selected DWI images. Third, they respectively hand-drew the ROI of the lesion on each map and recorded the sizes and the values of ROIs for the statistical analyses. The ROI1 contained the entire abnormal areas on the qCBF and qCBV maps, and the ROI2 contained the corresponding contralateral normal tissues. The ROI3 contained the entire lesions that appeared hyperintense on DWI as the ischemic core, and the ROI4 contained the corresponding contralateral normal tissues. The mismatch of ROI3 on DWI and ROI1 on the qCBF was the ischemic penumbra. Fourth, 1 author (MYW) independently checked the slices and ROIs. The authors discussed any inconsistencies, reached a unanimous decision, and implemented the following steps. We subsequently calculated the value of qCBF5 and qCBV5 in the DWI/PWI mismatch areas and qCBF6 and qCBV6 in the corresponding contralateral normal tissues (according to Eq. [3]).
Note: Vn, the value of qCBF, qCBV in ROI5 or ROI6; Vn-4, the value of qCBF, qCBV in ROI1 or ROI2; Vn-2, the value of qCBF, qCBV in ROI3 or ROI4; Sn-4, the size of ROI1 or ROI2; Sn-2, the size of ROI3 or ROI4.
Statistical analysis
All statistical analyses were performed with the software SPSS 26.0 (IBM Corp., Armonk, NY, USA, RRID: SCR_019096). Continuous variables were expressed as mean ± standard deviation or median (interquartile range), as appropriate. The 2-sided paired t-tests were performed for normally distributed continuous variables and Wilcoxon signed-rank tests for non-normally distributed continuous variables to detect the differences in the qCBF1 and qCBF2, qCBF3 and qCBF4, qCBF5 and qCBF6, qCBF3 and qCBF5, qCBV1 and qCBV2, qCBV3 and qCBV4, qCBV5 and qCBV6, and qCBV3 and qCBV5. The 2-sided Spearman’s rank correlation tests were performed to explore the correlation between the scores of NIHSS, mRS, and the values of qCBF1, qCBF3, qCBF5, qCBV1, qCBV3, and qCBV5. Receiver operating characteristic (ROC) curve analysis was used to identify the optimal critical qCBF thresholds of infarct core and ischemic penumbra. A value of P<0.05 was considered a statistically significant difference with 95% confidence intervals (CI).
Results
Figure 1 shows a flow diagram of the participant selection process. Among 20 charts reviewed, 14 patients met our inclusion criteria. Of those patients with AIS included in the analysis, 6 were females, 8 were males (mean age, 60.43 years; age range, 36 to 73 years). The time from the symptom onset to the SCALE-PWI scanning was 5.50 (10.25) days. The NIHSS score was (6.50±3.80), reflecting 35.71% minor stroke, 64.29% moderate severity stroke, and 0% severe stroke. The mRS score was 1.00 (0.25), reflecting 85.71% mild disability and 14.29% moderate to severe disability. Table 1 shows the patient demographic information in detail.
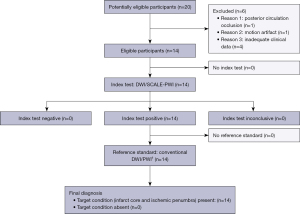
Table 1
Patient number | Gender | Age (year) | Time (day)† | NIHSS score†† | mRS score††† | Stroke subtypes†††† | Main stroke risk factors |
---|---|---|---|---|---|---|---|
2 | F | 51 | 1 | 3 | 1 | LAD | TIA* |
3 | F | 68 | 1 | 1 | 0 | LAD | Dyslipidemia |
4 | M | 62 | 15 | 11 | 5 | LAD | Dyslipidemia, coronary artery disease, smoking**, and drinking*** |
6 | M | 64 | 3 | 5 | 2 | LAD | Smoking |
7 | M | 66 | 11 | 10 | 1 | LAD | Dyslipidemia and smoking |
9 | F | 49 | 8 | 3 | 1 | LAD | Endometrial cancer resection before 4 years |
10 | M | 67 | 11 | 8 | 2 | LAD | Smoking |
12 | M | 44 | 11 | 8 | 1 | LAD | Smoking and drinking |
15 | M | 73 | 12 | 15 | 3 | LAD | Dyslipidemia and coronary artery disease |
16 | M | 36 | 2 | 6 | 1 | LAD | Obesity, smoking, and drinking |
17 | F | 59 | 2 | 4 | 1 | LAD | Hypertension |
18 | F | 65 | 1 | 3 | 1 | LAD | Dyslipidemia |
19 | M | 71 | 12 | 8 | 0 | LAD | Hypertension |
20 | F | 71 | 1 | 6 | 1 | LAD | Age |
Note: †, the time from symptom onset to SCALE-PWI scanning; ††, NIHSS scores recorded on the day of SCALE-PWI scanning; †††, mRS scores recored on the 90th day after symptom onset; ††††, the stroke subtypes were classified according to the TOAST criteria: large-artery atherosclerosis LAD, SVD, CE, ODE, UDE, and incomplete evaluation. *, previous TIA; **, current cigarette smoker; ***, regular alcohol consumption in the 3 months preceding the stroke. NIHSS, National Institutes of Health Stroke Scale; mRS, modified Rankin Scale; LAD, large-artery atherosclerosis; TIA, transient ischemic attack; SCALE-PWI, Self-CALibrated Echo-planar imaging PerfusionWeighted Imaging; TOAST, Trial of Org 10172 in Acute Stroke Treatment; SVD, small-vessel occlusion; CE, cardioembolism; ODE, other determined etiology; UDE, undetermined etiology.
Figure 2 shows the example of imaging manifestation in patients with left proximal MCA occlusion and the ROIs placement on a representative DWI-qCBF fusion image. Table 2 shows the values of qCBF and qCBV in all ROIs. The values of qCBF and qCBV in ROI1 were significantly lower than those in ROI2 (P=0.000 and 0.005, 95% CI, respectively). The values of qCBF and qCBV in ROI3 were lower than those in ROI4 (P=0.000 and 0.028, 95% CI, respectively). The values of qCBF and qCBV in ROI5 were lower than that in ROI6 (P=0.000 and 0.011, 95% CI, respectively). In summary, all the values of qCBF and qCBV in the lesions were lower than those of the contralateral normal tissues (all P<0.05, 95% CI). The values of qCBF and qCBV in the infarct core, ROI3, were lower than those in the ischemic penumbra, ROI5 (mean value: 16.42 vs. 21.54 mL/100 g/min, P=0.013, 95% CI, and 1.23 vs. 1.47 mL/100 g, P=0.049, 95% CI, respectively). The areas under the ROC curves (AUCs) were analyzed as measures to differentiate the infarct core from the ischemic penumbra (Figure 3). The AUC analysis yielded 0.84 for qCBF in identifying the ischemic penumbra (threshold, 28.09 mL/100 g/min; sensitivity, 78.60%; specificity, 85.70%; 95% CI). The AUC analysis yielded 0.68 for qCBF in identifying the infarct core (threshold, 18.18 mL/100 g/min; sensitivity, 71.40%; specificity, 64.30%; 95% CI). There was no statistical correlation between the scores of NIHSS, mRS, and the values of qCBF and qCBV (all P>0.05, 95% CI). There were no adverse events recorded in this study.
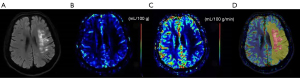
Table 2
ROI1 | ROI2 | ROI3 | ROI4 | ROI5 | ROI6 | |
---|---|---|---|---|---|---|
qCBF (mL/100 g/min) | 20.75±8.35 | 35.03±13.85 | 16.42±8.24 | 30.14±10.07 | 21.54±8.64 | 35.16±15.68 |
qCBV (mL/100 g) | 1.43±0.66 | 1.79±0.46 | 1.23±0.63 | 1.28 (0.67)† | 1.47±0.71 | 1.85±0.48 |
Note: †, nonparametric variable was expressed as median (interquartile range). Other continuous variables were expressed as mean ± standard deviation. qCBF, quantitative cerebral blood flow; qCBV, quantitative cerebral blood volume; SCALE-PWI, Self-Calibrated Echo planar imaging Perfusion-Weighted Imaging; ROI, region of interest.
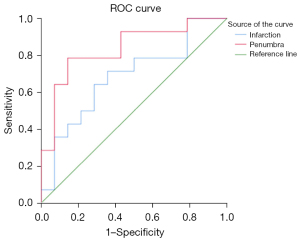
Discussion
To our knowledge, this was the first prospective study to describe the absolute quantitative perfusion parameters detected by SCALE-PWI in AIS patients. We found that both qCBV and qCBF were significantly reduced in ischemic lesions. In addition, qCBF in the infarct core decreased much more than that in the ischemic penumbra. These findings indicate that SCALE-PWI parameters are potential imaging biomarkers to reflect the severity and progression of pathologic disorder.
An AIS event occurs when the blood supply to the cerebral tissue is suddenly unable to be sufficiently and consistently maintained. In this study, SCALE-PWI was used to detect insufficient blood supply. The SCALE-PWI is a new sequence which produces an absolute scale for the quantification of cerebral perfusion based on the conventional, multiscan Bookend technique (11). The structure of the SCALE-PWI sequence is best achieved by having a single-shot EPI acquisition over all 3 modules with parallel imaging [i.e., generalized autocalibrating partially parallel acquisitions (GRAPPA)], which would accelerate both the calibration module and the DSC module (11). This provides the advantages of improving image quality and saving both scan time and post-processing time in clinical settings. The qCBF derived from the conventional Bookend technique provides enhanced discrimination of IS lesions compared to time-based imaging metrics, such as time to peak (Tmax) and mean transit time (MTT) (7). In addition, an excellent agreement is depicted between SCALE-PWI and the conventional Bookend technique (11). Therefore, this study incorporated an ROI analysis on qCBF images. The value of qCBF in the ischemic lesion was significantly lower than that in the corresponding contralateral normal tissue (20.75 vs. 35.03 mL/100 g/min and 16.42 vs. 30.14 mL/100 g/min, respectively). The value of qCBV in the ischemic lesion was also lower than that of the corresponding contralateral normal tissue (1.43 vs. 1.79 mL/100 g and 1.23 vs. 1.28 mL/100 g/min). Overall, the ischemic tissue was a result of lack of blood supply, which was in agreement with previous research (16).
The research on IS has focused not only on early detection of lesions but also on the goal of tissue-based decision-making and individualized AIS treatment (12), which are associated with the infarct core and the ischemic penumbra. The original concept of the ischemic penumbra, as defined by Astrup et al., is based on CBF thresholds (17). Astrup et al. defined 2 CBF thresholds: an upper CBF threshold where cells exhibit electrical failure, sustained energy metabolism, and low extracellular potassium, and a lower CBF threshold where extracellular potassium is increased alongside electrical failure and energy failure (17-19). There are 2 CBF thresholds because there are 2 types of energy demand of cerebral tissue: 87% of the total energy consumed is required for signaling, mainly in action potential propagation and postsynaptic ion fluxes, and only 13% is expended in maintaining membrane resting potential (16,20). Cerebral tissue with CBF between the 2 thresholds is defined as ischemic penumbra. The ischemic penumbra has the potential for recovery if reperfusion treatment is successful and prompt. However, prolongation of cerebral tissue with CBF below the lower threshold is a guaranteed cause of irreversible tissue death. According to statistics, 180 million neurons die per minute without prompt treatment after AIS (21,22). The SCALE-PWI enables us to differentiate between the infarct core and the ischemic penumbra by qCBF, which has the potential to provide imaging biomarker for improving the clinical outcomes and the quality of life of patients with AIS.
Over the past 2 decades, DWI-PWI parameters have become the imaging biomarkers for estimating the infarct core and the ischemic penumbra, and the PWI/DWI mismatch has been proposed as a surrogate marker of efficacy of AIS treatment (23). The assessment of the ischemic penumbra with MRI has widely used DSC PWI to identify its outer edge and DWI to identify its inner edge (24). According to this method, we contoured the infarct core as ROI3 and the ischemic penumbra as ROI5. In this study, the conventional PWI was replaced by SCALE-PWI. Different from the previous semi-quantitative PWI, the SCALE-PWI technique not only detects the perfusion deficit but also provides absolute quantitative hemodynamic information which could be used to detect the infarct core and the ischemic penumbra in a relatively short scan time. The mean qCBF value of the infarct core was lower than that of the ischemic penumbra (16.42 vs. 21.54 mL/100 g/min). According to the theory proposed by Astrup et al., this study explored the 2 optimal critical qCBF thresholds by ROC curve analysis. The lower qCBF threshold was 18.18 mL/100 g/min and the upper qCBF threshold was 28.09 mL/100 g/min, which could be used to the identify the infarct core and the ischemic penumbra. Clinically, this type of analysis could improve the observer independent assessment of a stroke area and enhance the value of MRI, while traditional DSC PWI remains limited with a high subjective bias.
As is known, positron emission tomography (PET) is still considered the current ‘state of the art’ technology for quantifying cerebral perfusion and the gold standard for detecting ischemic penumbra (11,25). However, owing to its invasive nature and high cost, PET is not used as commonly as MRI, in both the clinical work and research settings. The optimal critical PET-based CBF thresholds (12 and 22 mL/100 g/min) are lower than the SCALE-PWI-based qCBF thresholds (18.18 and 28.09 mL/100 g/min) because the imaging and processing methods are different. In addition, the contrast agents are different, consisting of a non-diffusible intravascular agent (Gd-DTPA) in SCALE-PWI and a diffusible agent (15O-Water) in PET (16,26). A comparative study of SCALE-PWI/PET in patients with AIS is warranted.
In conclusion, this study indicates that SCALE-PWI is feasible in AIS patients and can provide reliable quantitative hemodynamic information to detect the infarct core and ischemic penumbra in a relatively short scan time. We propose that SCALE-PEI can offer a unique insight into AIS pathophysiology and potentially serve as an imaging biomarker for tissue-based decision-making and personalized AIS treatment.
Limitations
This study had some limitations: it was a single-center study using advanced imaging to quantify CBF and CBV; the sample size was relatively small. Moreover, SCALE-PWI was not directly compared with the gold-standard PET measurements used in patients with AIS and microspheres in animal models.
Acknowledgments
Part of the study was presented as a poster at the 27th International Society for Magnetic Resonance in Medicine (ISMRM) Annual Meeting in 2019.
Funding: This work was supported by the National Natural Science Foundation of China (No. 81720108021), National Key R&D Program of China (No. 2017YFE0103600), and the Youth Project of Henan Medical Science and Technology Research Project (No. SBGJ202103078).
Footnote
Reporting Checklist: The authors have completed the STARD reporting checklist. Available at https://qims.amegroups.com/article/view/10.21037/qims-21-975/rc
Conflicts of Interest: All authors have completed the ICMJE uniform disclosure form (available at https://qims.amegroups.com/article/view/10.21037/qims-21-975/coif). The authors have no conflicts of interest to declare.
Ethical Statement: The authors are accountable for all aspects of the work in ensuring that questions related to the accuracy or integrity of any part of the work are appropriately investigated and resolved. This study was conducted in accordance with the Declaration of Helsinki (as revised in 2013). This study was approved by the institutional review board of Zhengzhou University People’s Hospital (No. 201967) and informed consent was provided by all the participants or their family members before participation.
Open Access Statement: This is an Open Access article distributed in accordance with the Creative Commons Attribution-NonCommercial-NoDerivs 4.0 International License (CC BY-NC-ND 4.0), which permits the non-commercial replication and distribution of the article with the strict proviso that no changes or edits are made and the original work is properly cited (including links to both the formal publication through the relevant DOI and the license). See: https://creativecommons.org/licenses/by-nc-nd/4.0/.
References
- Aho K, Harmsen P, Hatano S, Marquardsen J, Smirnov VE, Strasser T. Cerebrovascular disease in the community: results of a WHO collaborative study. Bull World Health Organ 1980;58:113-30. [PubMed]
- Wang W, Jiang B, Sun H, Ru X, Sun D, Wang L, Wang L, Jiang Y, Li Y, Wang Y, Chen Z, Wu S, Zhang Y, Wang D, Wang Y, Feigin VL. NESS-China Investigators. Prevalence, Incidence, and Mortality of Stroke in China: Results from a Nationwide Population-Based Survey of 480 687 Adults. Circulation 2017;135:759-71. [Crossref] [PubMed]
- GBD 2016 Stroke Collaborators. Global, regional, and national burden of stroke, 1990-2016: a systematic analysis for the Global Burden of Disease Study 2016. Lancet Neurol 2019;18:439-58. [Crossref] [PubMed]
- Jeong YI, Christoforidis GA, Saadat N, Kawaji K, Cantrell CG, Roth S, Niekrasz M, Carroll TJ. Absolute quantitative MR perfusion and comparison against stable-isotope microspheres. Magn Reson Med 2019;81:3567-77. [Crossref] [PubMed]
- Simonsen CZ, Madsen MH, Schmitz ML, Mikkelsen IK, Fisher M, Andersen G. Sensitivity of diffusion- and perfusion-weighted imaging for diagnosing acute ischemic stroke is 97.5%. Stroke 2015;46:98-101. [Crossref] [PubMed]
- Bateman M, Slater LA, Leslie-Mazwi T, Simonsen CZ, Stuckey S, Chandra RV. Diffusion and Perfusion MR Imaging in Acute Stroke: Clinical Utility and Potential Limitations for Treatment Selection. Top Magn Reson Imaging 2017;26:77-82. [Crossref] [PubMed]
- Shah MK, Shin W, Parikh VS, Ragin A, Mouannes J, Bernstein RA, Walker MT, Bhatt H, Carroll TJ. Quantitative cerebral MR perfusion imaging: preliminary results in stroke. J Magn Reson Imaging 2010;32:796-802. [Crossref] [PubMed]
- Sakaie KE, Shin W, Curtin KR, McCarthy RM, Cashen TA, Carroll TJ. Method for improving the accuracy of quantitative cerebral perfusion imaging. J Magn Reson Imaging 2005;21:512-9. [Crossref] [PubMed]
- Shin W, Cashen TA, Horowitz SW, Sawlani R, Carroll TJ. Quantitative CBV measurement from static T1 changes in tissue and correction for intravascular water exchange. Magn Reson Med 2006;56:138-45. [Crossref] [PubMed]
- Shin W, Horowitz S, Ragin A, Chen Y, Walker M, Carroll TJ. Quantitative cerebral perfusion using dynamic susceptibility contrast MRI: evaluation of reproducibility and age- and gender-dependence with fully automatic image postprocessing algorithm. Magn Reson Med 2007;58:1232-41. [Crossref] [PubMed]
- Srour JM, Shin W, Shah S, Sen A, Carroll TJ. SCALE-PWI: A pulse sequence for absolute quantitative cerebral perfusion imaging. J Cereb Blood Flow Metab 2011;31:1272-82. [Crossref] [PubMed]
- Bateman M, Slater LA, Leslie-Mazwi T, Simonsen CZ, Stuckey S, Chandra RV. Diffusion and Perfusion MR Imaging in Acute Stroke: Clinical Utility and Potential Limitations for Treatment Selection. Top Magn Reson Imaging 2017;26:77-82. [Crossref] [PubMed]
- Lemahafaka G, Camara A, Rajaonarison L, Vallet F. Ischemic stroke despite normal brain MRI: about a case. Pan Afr Med J 2016;25:22. [PubMed]
- Kamalian S, Lev MH. Stroke Imaging. Radiol Clin North Am 2019;57:717-32. [Crossref] [PubMed]
- Ramos-Lima MJM, Brasileiro IC, Lima TL, Braga-Neto P. Quality of life after stroke: impact of clinical and sociodemographic factors. Clinics (Sao Paulo) 2018;73:e418. [Crossref] [PubMed]
- Heiss WD, Zaro Weber O. Validation of MRI Determination of the Penumbra by PET Measurements in Ischemic Stroke. J Nucl Med 2017;58:187-93. [Crossref] [PubMed]
- Astrup J, Symon L, Branston NM, Lassen NA. Cortical evoked potential and extracellular K+ and H+ at critical levels of brain ischemia. Stroke 1977;8:51-7. [Crossref] [PubMed]
- McCabe C, Arroja MM, Reid E, Macrae IM. Animal models of ischaemic stroke and characterisation of the ischaemic penumbra. Neuropharmacology 2018;134:169-77. [Crossref] [PubMed]
- Astrup J, Siesjö BK, Symon L. Thresholds in cerebral ischemia - the ischemic penumbra. Stroke 1981;12:723-5. [Crossref] [PubMed]
- Laughlin SB, de Ruyter van Steveninck RR, Anderson JC. The metabolic cost of neural information. Nat Neurosci 1998;1:36-41. [Crossref] [PubMed]
- Ran YC, Zhu M, Li SJ, Zhang ZX, Wang X, Zhang Y, Cheng JL. Related Research and Recent Progress of Ischemic Penumbra. World Neurosurg 2018;116:5-13. [Crossref] [PubMed]
- Saver JL. Time is brain--quantified. Stroke 2006;37:263-6. [Crossref] [PubMed]
- Donnan GA, Baron JC, Ma H, Davis SM. Penumbral selection of patients for trials of acute stroke therapy. Lancet Neurol 2009;8:261-9. [Crossref] [PubMed]
- Leigh R, Knutsson L, Zhou J, van Zijl PC. Imaging the physiological evolution of the ischemic penumbra in acute ischemic stroke. J Cereb Blood Flow Metab 2018;38:1500-16. [Crossref] [PubMed]
- Heiss WD, Grond M, Thiel A, von Stockhausen HM, Rudolf J, Ghaemi M, Löttgen J, Stenzel C, Pawlik G. Tissue at risk of infarction rescued by early reperfusion: a positron emission tomography study in systemic recombinant tissue plasminogen activator thrombolysis of acute stroke. J Cereb Blood Flow Metab 1998;18:1298-307. [Crossref] [PubMed]
- Raichle ME, Martin WR, Herscovitch P, Mintun MA, Markham J. Brain blood flow measured with intravenous H2(15)O. II. Implementation and validation. J Nucl Med 1983;24:790-8. [PubMed]