Metabolite changes in prefrontal lobes and the anterior cingulate cortex correlate with processing speed and executive function in Parkinson disease patients
Introduction
Parkinson disease (PD) is one of the most common neurodegenerative diseases. The main pathological feature is degeneration of dopaminergic neurons in the substantia nigra (1). Clinically, typical motor symptoms of PD are characterized by bradykinesia, resting tremor, and rigidity. However, a wide range of nonmotor disturbances can occur in patients with PD. Cognitive impairment, especially impairment of executive function (EF), attention, visuospatial, and memory commonly present in patients with PD who have nonmotor symptoms. Longitudinal studies show that approximately 20% of the patients with PD develop dementia within 5 years of follow-up, which eventually develops to 80% at 20 years of follow-up (2,3).
Cognitive deterioration, especially of the executive dysfunction, has received considerable research attention as it may predict progression to dementia and has a significant impact on the patients’ daily life and their quality of life. The main components of EF include the allocation and internal control of attention, planning, inhibition, set shifting, concept formation, dual task performance, decision-making, and social cognition (4,5).
Neuroimaging is an attractive option for identifying biomarkers of cognitive status, especially progression to dementia, and may facilitate prompt interventions to slow cognitive decline. However, results from neuroimaging studies that explore EF and processing speed in patients with PD were not always consistent, which may be related to the vague concept of EF itself and the use of different cognitive assessment scales. In terms of understanding the neuroanatomical substrate of EF, previous studies support the notion that the frontal and parietal cortex play an important role in EF (6,7). However, identifying a clear correspondence between specific brain regions and subcomponents of EFs has remained a challenge in this field.
Structural and functional abnormalities of the anterior cingulate cortex (ACC) and the prefrontal cortex have been found in PD both for patients with mild cognitive impairment (PD-MCI) and for those with dementia. In our previous work, we found that the cortical thickness of the ACC correlated with the atrophy of the Meynert nucleus in patients with PD-MCI (8). Another study found the components of ACC structural covariance networks to be associated with cognitive impairment in patients with mild-to-moderate PD (9). A decrease of the regional cerebral blood flow (rCBF) in the ACC and the dorsolateral prefrontal cortex may reflect cognitive dysfunction in patients with PD (10). Previous studies have reported brain metabolism deficits in the ACC to be correlated with executive performance in patients with PD (11-13). In addition, a functional magnetic resonance imaging (fMRI) study demonstrated that a decline in the memory and visuospatial domains may be associated with stronger coupling between the dorsal caudate and the rostral ACC (14). These findings suggest that PD-related executive deficits and brain changes are heterogeneous; however, the processing speed-related changes remain to be fully elucidated.
Proton magnetic resonance spectroscopy (1H-MRS) is a method used to investigate the biochemical index and metabolic changes through quantification of a range of metabolites, including N-acetyl-aspartate (NAA), choline-containing compounds (Cho), and creatine (Cr), in different brain structures (15,16). NAA is an indicator of neuronal integrity, and the decreased ratio of NAA:Cr indicates neuronal and axonal loss or dysfunction (17). Meanwhile, Cho is an indicator of membrane metabolism, and an increased ratio of Cho to Cr corresponds with increased membrane turnover (17). One study found that, compared with healthy control (HC) subjects, PD-MCI patients showed a reduced NAA:Cr ratio in the occipital lobe and an increased Cho:Cr ratio in the posterior cingulate (18).
Therefore, the principal objective of this study is to explore the characteristics of cognitive function changes in patients with PD, especially in terms of the subcomponents of EF and processing speed. Specifically, we used 1H-MRS to investigate the metabolic changes of the frontal lobe and the ACC in patients with PD, and further explored the association between cognitive function and metabolic ratios in the frontal lobe and the ACC. We present the following article in accordance with the STROBE reporting checklist (available at https://qims.amegroups.com/article/view/10.21037/qims-21-1126/rc).
Methods
Participants
In this retrospective case-control study, patients with PD from the Department of Neurology at Guangdong Provincial Peoples’ Hospital in China and age-, sex-, and years-of-education-matched HCs were enrolled in this study from October 2018 to November 2020. All patients with PD fulfilled the following criteria: (I) they met the Movement Disorder Society (MDS) Clinical Diagnostic Criteria for PD (19); (II) they were not treated with any antidepressant medications; and (III) they did not have other Parkinsonian syndrome or other neurological diseases. The flowchart of the excluded patients with PD can be seen in Figure 1. In all, 59 patients with PD and 30 HCs were available for the study for clinical and demographic data. One of the patients with PD was excluded because the baseline of the MRS spectra was unstable. Finally, 58 patients were available for MRS analysis.
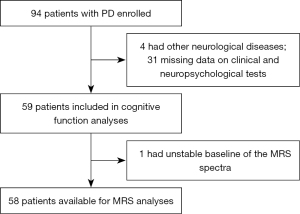
Clinical and neuropsychological evaluation
The motor symptoms were measured with the modified Hoehn-Yahr scale (20) and the MDS Unified Parkinson Disease Rating Scale (MDS-UPDRS) part III (21). The severity of depression and anxiety were assessed using the scores of the 24-item Hamilton Depression Rating Scale (22) and Hamilton Anxiety Rating Scale (23). The neuropsychological test battery included the Mini-Mental State Examination (MMSE) (24) and the Montreal Cognitive Assessment (MoCA) (25) for global cognition. The tests to assess EF and processing speed were as follows: verbal fluency test-semantic (26), Wechsler Adult Intelligence Scale (WAIS)-Similarities (27), WAIS-Digit symbol coding (27), Stroop color-word test (28), WAIS-Digit span, and Wisconsin Card Sorting Test (WCST-128) (29). Processing speed was measured using the Stroop A (word naming test) and the WAIS-Digit symbol test. The speed of performance on the color-word trial was subtracted from the speed on the color naming test to calculate interference [Stroop interference effect (SIE)]. The number of completed categories in WSCT is frequently used as a measure of shift and deduction functions. Most prominently, deficits in cognitive flexibility are commonly thought to be reflected in the number of perseverative errors in WSCT. The levodopa equivalent daily dose (LEDD) was appropriately calculated (30). This study was conducted in accordance with the Declaration of Helsinki (as revised in 2013). All participants provided written informed consent to participate in the study. This study was approved by the Medical Ethics Committee of Guangdong Provincial People’s Hospital (No. GDREC2019490H[R1]). The demographic and clinical characteristics of the patient and control groups are shown in Table 1.
Table 1
Variables | PD (n=59) | HC (n=30) | t/Z/χ2 | P |
---|---|---|---|---|
Male/female | 34/25 | 14/16 | 0.962 | 0.373 |
Age (years) | 60.47±8.74 | 61.10±7.67 | −0.332 | 0.741 |
Education years | 10.67±3.61 | 11.17±3.57 | −0.616 | 0.539 |
PD duration (months) | 24 [12–36] | – | – | – |
Age at PD onset (years) | 57.78±8.87 | – | – | – |
LEDD total (mg/day) | 87.5 [0–337.5] | – | – | – |
Modified Hoehn-Yahr | 2 [2–2.5] | – | – | – |
MDS-UPDRS-III | 32.27±13.72 | – | – | – |
HAMD | 11 [5–16] | 2 [0–3.25] | −6.253 | <0.001 |
HAMA | 7 [4–13] | 2 [0–5] | −4.461 | <0.001 |
MMSE | 28 [27–29] | 29 [28–29.25] | −1.628 | 0.103 |
MoCA | 24 [19–26] | 27 [26–28] | −5.041 | <0.001 |
Age, education years, age at PD onset, and MDS-UPDRS-III are expressed as mean ± standard deviation. PD duration, LEDD total, HAMD, HAMA, MMSE, and MoCA are expressed as median [interquartile range]. PD, Parkinson disease; HC, healthy control; LEDD, Levodopa equivalent daily dose, MDS-UPDRS-III, Movement Disorder Society-sponsored Revision of the Unified Parkinson Disease Rating Scale part III; HAMD, Hamilton Depression Scale; HAMA, Hamilton Anxiety Scale; MMSE, Mini-Mental State Examination; MoCA, Montreal Cognitive Assessment.
Neuroimaging
Routine MRI and 1H-MRS were performed with a GE Signa Excite 3.0 T scanner (GE Healthcare) with a standard 8-channel head coil. Single-voxel 1H-MRS was used with point resolved spectroscopy sequences (PRESS) with a repetition time (TR)/echo time (TE) of 1,500/144 ms and 128 acquisitions. Spectra were shimmed to achieve a full-width half maximum (FWHM) of <12 Hz, and the percentage of water suppression was higher than 95% to minimize the influence of the signal-to-noise ratio. Volumes of interest (2 cm × 2 cm × 2 cm) were located within the bilateral prefrontal cortex and the ACC (Figure 2). The spectra were postprocessed automatically using the Sage 7.0 spectrum processing software system within the magnetic resonance (MR) scanner for filtering and reconstruction, zero filling, phase, and baseline correction. For each patient, the ratios of NAA and Cho relative to Cr were computed.
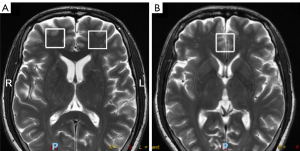
Statistical analysis
Analyses were carried out using the SPSS v. 20 (IBM SPSS, Armonk, NY, USA). The Shapiro-Wilk test was used to assess the normality of the data. Between the PD group and HC group, demographic clinical, cognitive, and MRS variables were analyzed with two-sample t-tests for normally distributed variables, while the Mann-Whitney test was used for nonnormally distributed variables. The chi-squared test was used to analyze categorical data. The preliminary correlation analysis between processing speed and ratios of metabolite concentration distribution was conducted for the PD group. Hierarchical multiple regressions were applied to assess the association between cognitive function and the ratios of metabolite concentrations in the prefrontal lobe and the ACC in patients with PD, with age and MDS-UPDRS-III entered in the first step as control variables, and metabolite ratios entered in the second step. Multiple comparisons were corrected using the Bonferroni procedure with a factor of 6 to account for 3 regions of interest (ROIs) and 2 metabolic measures (P<0.0083). Statistical significance was set at a P value <0.05. Furthermore, in the post-hoc analysis, metabolite ratios were compared among the different stages of general cognitive impairment (i.e., MoCA score ≤20, MoCA score =21–25, MoCA score ≥26) from patients with PD using one-way analysis of variance (ANOVA), and the Bonferroni test was used to correct for multiple comparisons in post-hoc comparisons.
Results
In all, 59 patients with PD and 30 HCs were available for the study and had clinical and demographic data. In the MRS analysis, one of the patients with PD was excluded because the baseline of the MRS spectra was unstable. No significant statistical difference was found in gender (χ2=0.962; P=0.373), age (t=–0.332; P=0.741), or education (t=–0.616; P=0.539). The MoCA scores in general cognition were significantly decreased in the PD group (Z=–5.041; P<0.001). However, the MMSE scores were not significantly different between the 2 groups (Z=–1.628; P=0.103). The detailed results are shown in Table 1.
The verbal fluency test (t=–4.765; P<0.001) and the similarity test (t=–6.786; P<0.001) performances that indicated task fluency and information generation ability, were worse in patients with PD. In the processing speed measurement, patients with PD showed significantly decreased performance, which was reflected by significantly lower scores on digit symbol coding and by the longer completion times in the Stroop word naming and color naming subtests. Compared to HCs, patients with PD showed significantly poorer performance on tests of attention and working memory (WAIS-Digit span; Z=–4.958; P<0.001), but not on set-shifting (WSCT; P>0.05) or response inhibition (SIE; P>0.05). The detailed results of the comparison of cognitive function between the 2 groups are shown in Table 2.
Table 2
Variables | PD (n=59) | HC (n=30) | t/Z | P |
---|---|---|---|---|
Verbal fluency test-semantic | 14.98±3.89 | 20.13±5.23 | −4.765 | <0.001 |
WAIS-Similarities | 12.37±4.80 | 19.27±3.95 | −6.786 | <0.001 |
WAIS-Digit symbol coding | 26.98±10.49 | 42.43±9.86 | −6.701 | <0.001 |
Stroop A times | 32 [28–39] | 26 [23–30] | −3.786 | <0.001 |
Stroop B times | 52 [45–64] | 33.5 [30.75–39.5] | −6.147 | <0.001 |
Stroop C times | 89 [74–103] | 63.5 [59.5–72.5] | −4.758 | <0.001 |
Stroop SIE times | 35 [23–49] | 30 [20–37] | −1.337 | 0.181 |
WAIS-Digit span | 12 [10–12] | 14 [12.75–16] | −4.958 | <0.001 |
WSCT-completed categories | 6 [5–6] | 6 [5.75–6] | −1.236 | 0.216 |
WSCT-perseverative errors | 38.75±19.59 | 30.23±18.95 | 1.959 | 0.053 |
Verbal fluency test, Similarity test, Digit symbol coding, WSCT-perseverative errors, and WSCT-completed categories are expressed as mean ± standard deviation. Stroop A, B, C, and SIE times, as well as WAIS-Digit span, are expressed as median [interquartile range]. PD, Parkinson disease; HC, healthy control; SIE, Stroop interference effects (SIE = Stroop C time − Stroop B time); WAIS, Wechsler Adult Intelligence Scale; WSCT, Wisconsin Card Sorting Test.
Compared to HCs, patients with PD showed significantly increased Cho:Cr ratios in the right prefrontal cortex (RPF; t=2.16; P=0.034) and ACC (Z=–2.20; P=0.028) regions (Table 3, Figure 3). The typical MRS spectra are shown in Figure 4. However, these differences did not survive correction for multiple comparisons (P<0.0083).
Table 3
Metabolite ratios | PD (n=58) | HC (n=30) | t/Z | P |
---|---|---|---|---|
ACC | ||||
NAA:Cr | 1.40±0.14 | 1.44±0.16 | −1.07 | 0.285 |
Cho:Cr | 1.09 [1.00–1.15] | 1.025 [0.90–1.10] | −2.20 | 0.028* |
RPF | ||||
NAA:Cr | 1.66 [1.53–1.85] | 1.72 [1.49–1.86] | −0.29 | 0.768 |
Cho:Cr | 1.09±0.18 | 1.00±0.18 | 2.16 | 0.034* |
LPF | ||||
NAA:Cr | 1.78±0.27 | 1.84±0.32 | −0.87 | 0.385 |
Cho:Cr | 1.08 [0.94–1.20] | 1.03 [0.86–1.17] | −1.53 | 0.126 |
Data are expressed as mean ± standard deviation or median [interquartile range]. *, P<0.05. PD, Parkinson disease; HC, healthy control; ACC, anterior cingulate cortex; RPF, right prefrontal cortex; LPF, left prefrontal cortex; Cho, choline-containing compounds; Cr, creatine; NAA, N-acetyl-aspartate.
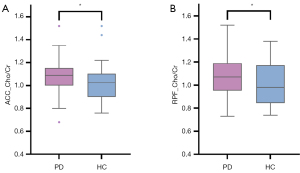
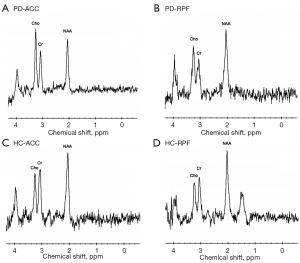
In the correlation analysis, the NAA:Cr ratio in the RPF was significantly related to the scores of the WAIS-Digit symbol coding test. The relationship between NAA:Cr ratio in the ACC and Stroop A completion times was not significant (P=0.055) (Table S1). The MDS-UPDRS-III score was not correlated with processing speed in the PD group significantly (P=0.062) (Table S2). We performed a correlation analysis between metabolites in the PD group to narrow the independent variables of the regression analysis (Table S3). A strong correlation between the Cho:Cr ratio in the RPF and the ACC was found, and thus the Cho:Cr ratio in the RPF was not included in further regression analysis. Therefore, in the hierarchical multiple regression analysis, age and the MDS-UPDRS-III were included as control variables. In hierarchical multiple regression model 1, age and MDS-UPDRS-III accounted for 4.2% of the variance on step 1. On step 2, metabolite measurements in the ACC accounted for a further 15.6% of the variance in the Stroop A completion times. Examination of β weights suggested that the correlate of Stroop A completion times was NAA:Cr ratio in the ACC (P<0.05), while a positive relationship with Cho:Cr ratio in the ACC was not significant (P=0.058). In model 2, with the WAIS-Digit symbol coding test score as the dependent variable, the significant correlate at P<0.05 was the NAA:Cr ratio of the RPF. Overall, model 2 accounted for 22.4% of the variance in the WAIS-Digit symbol coding test score (Table 4). These correlations were not found in the HC groups (Table S4).
Table 4
Models | β | t | P | R2 | R2 change | F change | F change P value |
---|---|---|---|---|---|---|---|
Model 1 (DV: Stroop A completion times) | |||||||
Step 1 | 0.042 | 0.042 | 1.213 | 0.305 | |||
Age | 0.147 | 1.108 | 0.273 | ||||
MDS-UPDRS-III | 0.156 | 1.181 | 0.243 | ||||
Step 2 | 0.156 | 0.114 | 3.568 | 0.035* | |||
Age | 0.221 | 1.687 | 0.098a | ||||
MDS-UPDRS-III | 0.207 | 1.618 | 0.112 | ||||
ACC_NAA/Cr | −0.292 | −2.238 | 0.029* | ||||
ACC_Cho:Cr | 0.259 | 1.938 | 0.058a | ||||
Model 2 (DV: WAIS-Digit symbol coding test) | |||||||
Step 1 | 0.151 | 0.151 | 4.908 | 0.011* | |||
Age | −0.272 | −2.181 | 0.033* | ||||
MDS-UPDRS-III | −0.301 | −2.418 | 0.019* | ||||
Step 2 | 0.224 | 0.072 | 1.617 | 0.197 | |||
Age | −0.305 | −2.407 | 0.020* | ||||
MDS-UPDRS-III | −0.270 | −2.147 | 0.036* | ||||
ACC_NAA/Cr | −0.005 | −0.040 | 0.968 | ||||
ACC_Cho:Cr | −0.130 | −1.003 | 0.321 | ||||
RPF_NAA/Cr | 0.257 | 2.032 | 0.047* |
a, trend-level, 0.05<P<0.1; *, P<0.05. β, standardized coefficient; DV, dependent variable; PD, Parkinson disease; MDS-UPDRS-III, Movement Disorder Society-sponsored Revision of the Unified Parkinson Disease Rating Scale part III; ACC, anterior cingulate cortex; RPF, right prefrontal cortex; LPF, left prefrontal cortex; Cho, choline-containing compounds; Cr, creatine; NAA, N-acetyl-aspartate; WAIS, Wechsler Adult Intelligence Scale.
In the post-hoc analysis among the different stages of cognitive impairment from patients with PD, a significant reduction in the metabolite ratios of NAA:Cr in the right prefrontal cortex was observed in PD group A (MoCA score ≤20) compared to the PD group C (MoCA score ≥26) (P=0.044, Bonferroni corrected; Table 5).
Table 5
Metabolite ratios | PD group A (MoCA score ≤20) (n=19) | PD group B (MoCA score =21–25) (n=21) | PD group C (MoCA score ≥26) (n=18) | ANOVA | Post-hoc analyses (Bonferroni corrected) | ||||
---|---|---|---|---|---|---|---|---|---|
F | P | PD-group A vs. PD-group B | PD-group A vs. PD-group C | PD-group B vs. PD-group C | |||||
ACC_NAA:Cr | 1.384±0.162 | 1.390±0.124 | 1.439±0.131 | 0.867 | 0.426 | 1.000 | 0.715 | 0.826 | |
ACC_Cho:Cr | 1.029±0.119 | 1.085±0.152 | 1.132±0.152 | 2.462 | 0.095 | 0.657 | 0.093 | 0.906 | |
RPF_NAA:Cr | 1.582±0.221 | 1.723±0.239 | 1.770±0.216 | 3.510 | 0.037* | 0.161 | 0.044* | 1.000 | |
RPF_Cho:Cr | 1.056±0.200 | 1.090±0.126 | 1.121±0.198 | 0.625 | 0.539 | 1.000 | 0.807 | 1.000 | |
LPF_NAA:Cr | 1.689±0.254 | 1.784±0.275 | 1.869±0.278 | 2.070 | 0.136 | 0.813 | 0.141 | 0.987 | |
LPF_Cho:Cr | 1.066±0.270 | 1.069±0.176 | 1.172±0.218 | 1.349 | 0.268 | 1.000 | 0.470 | 0.473 |
Data are expressed as mean ± standard deviation. Bonferroni-corrected for multiple comparisons. *, P<0.05. PD, Parkinson disease; MoCA, Montreal Cognitive Assessment; ANOVA, analysis of variance; ACC, anterior cingulate cortex; RPF, right prefrontal cortex; LPF, left prefrontal cortex; Cho, choline-containing compounds; Cr, creatine; NAA, N-acetyl-aspartate.
Discussion
The present study comprehensively investigated the executive profiles and processing speed in patients with PD, who in turn showed worse performance in EF and processing speed compared with HCs. The Cho:Cr ratios in the ACC and the right prefrontal cortex were significantly increased in patients with PD compared to HCs. Hierarchical multiple regressions showed that the metabolic ratios in the ACC of patients with PD correlated with the Stroop A completion times, while the NAA:Cr ratios of the right prefrontal cortex correlated with the scores of the WAIS-Digit symbol substitution subtest.
The scores for the processing speed test were significantly decreased in patients with PD compared with those in HCs. Furthermore, the performance of multiple executive domains, such as attention and working memory tests, were poorer in patients with PD compared with HCs. However, selective domain-specific tests for set-shifting and response inhibition were not found to be significantly worse in the PD group compared to HCs. The decline in information processing speed can be manifested as impairment of the initiation ability and reaction speed, which is related to the ability to detect and react to stimuli in order to maintain attentional and motivational status (31,32). Therefore, processing speed plays a role in executive dysfunction. To date, the most influential neuropsychology and cognitive test models in cognitive science are the processing speed theory (33) and the prefrontal executive theory (34). Processing speed theory contends that age-related cognitive declines can be accounted for by a single or global slowing of cognitive processing. The generalized slowdown is thought to be due to a disruption of white matter integrity throughout the whole brain. In contrast, the prefrontal executive theory states that cognitive declines, specifically in executive abilities, lead to more general cognitive deficits and may be attributed to local structural and functional changes in the frontal cortex areas. However, the respective roles of processing speed and EF in age-related cognitive decline have not yet been fully elucidated (35). EF can represent a combination of established cognitive abilities, such as reasoning and perception speed (35). Previous studies have revealed that the differences in age-related central EF can be eliminated by controlling for the processing speed (36). However, there is also evidence that the decline in age-related EF is not only in processing speed (37). It is worth noting that the patients with PD in this study were mainly at Hoehn-Yahr stage 2–2.5. The different states of the disease may be important influencing factors of inhibitory function. A previous study found reactive inhibition but not proactive inhibition to be more impaired in patients with PD at Hoehn-Yahr stage 1 compared to HCs (38). Early dopaminergic medication could increase the dopaminergic level of the dorsal striatum to improve the function of the dorsal frontal lobe-striatum circuit and ameliorate the related cognitive functions, such as planning and set shift ability (39). Cognitive function impairment may also be associated with limbic and orbitofrontal circuits, such as conditional associative, reverse learning, and reward learning (40,41).
We compared the PD cognition-related prefrontal cortex and ACC metabolic profile alteration between PD and HC groups using 1H-MRS. The increase in Cho:Cr ratios was evident within the right prefrontal cortex and the ACC in the PD group compared with the HC group. However, as the data did not survive Bonferroni correction, this finding should be interpreted with caution. The Cho signal, which mainly includes membrane phospholipid derivatives, is considered to be a marker of cell membrane turnover and breakdown (42,43). Increased Cho:Cr ratios might be the result of membrane structure damage of neurons (44). Furthermore, Cho levels may also be related to an early neuroinflammatory condition in PD (45).
In hierarchical multiple regression models, a change in metabolic ratios in the ACC and RPF was associated with a poor Stroop A test (word reading) performance and poor scores on the WAIS-Digit symbol coding test in patients with PD. These two tests reflect the processing speed performance of patients. Processing speed has been found associated with functional connectivity of the cingulo-opercular network (C-O), including the anterior insula/frontal operculum and the ACC, which can reflect the functions of attentional initiation, allocation, and behavioral adjustment in response to tasks (46). In one study, the C-O network was activated during perceptual and episodic memory search tasks, which involved trial initiation, target detection, decision-making, and response, indicating its consistent involvement in a broad range of cognitive processes (31). Furthermore, the C-O network is related to the maintenance of performance during tasks (46).
We found that the reduction of NAA levels in the right prefrontal cortex of patients with PD was associated with worse performance in the digit symbol coding test and a slowdown of processing speed. NAA represents the degree of amino acid concentration within neurons and is viewed as a marker of neuronal function. NAA plays a role in mediating osmoregulation and acid-base homeostasis. Reductions of NAA on 1H-MRS are considered as either a depletion of the number of neurons or a loss of neuronal function (47-49). NAA concentrations may be used as one of the earliest neuroimaging markers at the subjective cognitive decline stage (50). Therefore, the loss of the number and function of neurons in the right prefrontal cortex may be important factors that cause processing speed to slowdown. Neuroimaging studies using single-photon emission computed tomography (SPECT) associated processing slowness with a decreased glucose metabolism in the prefrontal cortex (51). Prefrontal Cho/Cr may characterize PD-associated fronto-striatal cognitive syndrome and affect EF. A previous study using data-driven independent component analysis found that reduced connectivity between the dorsal attention network and right fronto-insular regions in PD-MCI patients was related to attention and executive dysfunction (7).
In our post hoc analysis, we examined the MRS metabolite ratios in different stages of general cognitive impairment (MoCA score ≤20, MoCA score =21–25, MoCA score ≥26) from patients with PD: the PD group with MoCA scores ≤20 displayed significantly deceased ratios of NAA:Cr in the right prefrontal cortex. Consistent with the literature, our study found decreased a NAA:Cr ratio in the PD group with the most severe cognitive impairment (MoCA <20). Although not a substitute for comprehensive neuropsychological examination, the MoCA of global screening scales is recommended by the MDS Task Force in their rating scale to assess PD (52). The scale consists of 9 assessment parts: memory, visuo-constructional skills, attention, concentration, EFs, language, conceptual thinking, calculations, and orientation. Generally, cutoffs of 25/26 points for PD-MCI and 20/21 points for Parkinson disease dementia (PD-D) are suggested for screening in clinical practice (52). As mentioned above, when the MoCA scale is weighted toward frontal domains like executive abilities or attention, it may be more likely to be altered in a larger proportion of patients with PD. Indeed, one study found executive, visuospatial, and memory deficits to be associated with a higher risk of dementia conversion, with frontal/executive dysfunction contributing most to the occurrence of PD-D (53).
However, in our study, further multiple linear regression analysis unexpectedly showed that metabolite ratios for the bilateral prefrontal cortex and the ACC were only associated with the processing speed measure, with no correlation with measures of EF, such as semantic fluency, Stroop inhibition, WAIS-Similarities, shift, or deduction functions in the WSCT test. Therefore, caution should be exercised when using 1H-MRS to serve as a marker of EF. These findings need further confirmation with longitudinal data.
Our study had some limitations. First, we used MRS ratios in reference to Cr but lacked concentration quantification. Second, we only selected 3 ROIs in the PD to analyze focal damage using MRS rather than analyzing more diffuse damage with other functional imaging techniques. The ROI measurements in prefrontal lobes were mainly in the white matter wherein projections with other cortical and thalamic projections might have been involved. Therefore, functional imaging techniques should be explored further to assess the impact of diffuse brain pathology on cognitive impairment in patients with PD. Third, the modified Hoehn-Yahr scale has some limitations, such as the nonlinear relationship with disease progression, more focus on postural instability and mobility problems. However, this scale is widely used to quantify disease severity and functional burden, and may be useful for clinical studies that evaluate therapeutic interventions and prognostic factors in Parkinsonism (54,55). Finally, the effects of antiparkinsonian medications on the current findings cannot be fully ruled out. Future work using multicenter prospective PD cohorts could aim to evaluate the degeneration of the prefrontal lobes and ACC and the effects on cognitive function.
Conclusions
Multiple executive domains and processing speed were impaired in patients with PD. The increase in Cho:Cr ratios was evident within the right prefrontal cortex and the ACC in patients with PD, which may be associated with membrane structure damage of neuronal cells. Loss of the number and function of neurons in the right prefrontal cortex and the ACC may be important factors that cause a slowdown of processing speed in patients with PD.
Acknowledgments
Funding: This work was supported by the National Natural Science Foundation of China (No. 82071419), the Guangzhou Municipal People’s Livelihood Science and Technology Project (No. 201803010085), the High-level Hospital Construction Project (No. DFJH201907), the Supporting Research Funds for Outstanding Young Medical Talents in Guangdong Province (No. KJ012019442), and the Medical Scientific Research Foundation of Guangdong Province, China (No. A2019141).
Footnote
Reporting Checklist: The authors have completed the STROBE reporting checklist. Available at https://qims.amegroups.com/article/view/10.21037/qims-21-1126/rc
Conflicts of Interest: All authors have completed the ICMJE uniform disclosure form (available at https://qims.amegroups.com/article/view/10.21037/qims-21-1126/coif). The authors have no conflicts of interest to declare.
Ethical Statement: The authors are accountable for all aspects of the work in ensuring that questions related to the accuracy or integrity of any part of the work are appropriately investigated and resolved. This study was conducted in accordance with the Declaration of Helsinki (as revised in 2013). All participants provided written informed consent to participate in the study. This study was approved by the Medical Ethics Committee of Guangdong Provincial People’s Hospital (No. GDREC2019490H[R1]).
Open Access Statement: This is an Open Access article distributed in accordance with the Creative Commons Attribution-NonCommercial-NoDerivs 4.0 International License (CC BY-NC-ND 4.0), which permits the non-commercial replication and distribution of the article with the strict proviso that no changes or edits are made and the original work is properly cited (including links to both the formal publication through the relevant DOI and the license). See: https://creativecommons.org/licenses/by-nc-nd/4.0/.
References
- Tolosa E, Garrido A, Scholz SW, Poewe W. Challenges in the diagnosis of Parkinson's disease. Lancet Neurol 2021;20:385-97. [Crossref] [PubMed]
- Aarsland D, Andersen K, Larsen JP, Lolk A, Kragh-Sørensen P. Prevalence and characteristics of dementia in Parkinson disease: an 8-year prospective study. Arch Neurol 2003;60:387-92. [Crossref] [PubMed]
- Hely MA, Reid WG, Adena MA, Halliday GM, Morris JG. The Sydney multicenter study of Parkinson's disease: the inevitability of dementia at 20 years. Mov Disord 2008;23:837-44. [Crossref] [PubMed]
- Dirnberger G, Jahanshahi M. Executive dysfunction in Parkinson's disease: a review. J Neuropsychol 2013;7:193-224. [Crossref] [PubMed]
- Godefroy O, Martinaud O, Narme P, Joseph PA, Mosca C, Lhommée E, et al. Dysexecutive disorders and their diagnosis: A position paper. Cortex 2018;109:322-35. [Crossref] [PubMed]
- Hou Y, Yang J, Luo C, Song W, Ou R, Liu W, Gong Q, Shang H. Dysfunction of the Default Mode Network in Drug-Naïve Parkinson's Disease with Mild Cognitive Impairments: A Resting-State fMRI Study. Front Aging Neurosci 2016;8:247. [Crossref] [PubMed]
- Baggio HC, Segura B, Sala-Llonch R, Marti MJ, Valldeoriola F, Compta Y, Tolosa E, Junqué C. Cognitive impairment and resting-state network connectivity in Parkinson's disease. Hum Brain Mapp 2015;36:199-212. [Crossref] [PubMed]
- Rong S, Li Y, Li B, Nie K, Zhang P, Cai T, Mei M, Wang L, Zhang Y. Meynert nucleus-related cortical thinning in Parkinson's disease with mild cognitive impairment. Quant Imaging Med Surg 2021;11:1554-66. [Crossref] [PubMed]
- de Schipper LJ, van der Grond J, Marinus J, Henselmans JML, van Hilten JJ. Loss of integrity and atrophy in cingulate structural covariance networks in Parkinson's disease. Neuroimage Clin 2017;15:587-93. [Crossref] [PubMed]
- Wakamori T, Agari T, Yasuhara T, Kameda M, Kondo A, Shinko A, Sasada S, Sasaki T, Furuta T, Date I. Cognitive functions in Parkinson's disease: relation to disease severity and hallucination. Parkinsonism Relat Disord 2014;20:415-20. [Crossref] [PubMed]
- Christopher L, Duff-Canning S, Koshimori Y, Segura B, Boileau I, Chen R, Lang AE, Houle S, Rusjan P, Strafella AP. Salience network and parahippocampal dopamine dysfunction in memory-impaired Parkinson disease. Ann Neurol 2015;77:269-80. [Crossref] [PubMed]
- Picco A, Morbelli S, Piccardo A, Arnaldi D, Girtler N, Brugnolo A, Bossert I, Marinelli L, Castaldi A, De Carli F, Campus C, Abbruzzese G, Nobili F. Brain (18)F-DOPA PET and cognition in de novo Parkinson's disease. Eur J Nucl Med Mol Imaging 2015;42:1062-70. [Crossref] [PubMed]
- Ekman U, Eriksson J, Forsgren L, Mo SJ, Riklund K, Nyberg L. Functional brain activity and presynaptic dopamine uptake in patients with Parkinson's disease and mild cognitive impairment: a cross-sectional study. Lancet Neurol 2012;11:679-87. [Crossref] [PubMed]
- Manza P, Zhang S, Li CS, Leung HC. Resting-state functional connectivity of the striatum in early-stage Parkinson's disease: Cognitive decline and motor symptomatology. Hum Brain Mapp 2016;37:648-62. [Crossref] [PubMed]
- Graff-Radford J, Boeve BF, Murray ME, Ferman TJ, Tosakulwong N, Lesnick TG, Maroney-Smith M, Senjem ML, Gunter J, Smith GE, Knopman DS, Jack CR Jr, Dickson DW, Petersen RC, Kantarci K. Regional proton magnetic resonance spectroscopy patterns in dementia with Lewy bodies. Neurobiol Aging 2014;35:1483-90. [Crossref] [PubMed]
- Zanigni S, Testa C, Calandra-Buonaura G, Sambati L, Guarino M, Gabellini A, Evangelisti S, Cortelli P, Lodi R, Tonon C. The contribution of cerebellar proton magnetic resonance spectroscopy in the differential diagnosis among parkinsonian syndromes. Parkinsonism Relat Disord 2015;21:929-37. [Crossref] [PubMed]
- Gujar SK, Maheshwari S, Björkman-Burtscher I, Sundgren PC. Magnetic resonance spectroscopy. J Neuroophthalmol 2005;25:217-26. [Crossref] [PubMed]
- Nie K, Zhang Y, Huang B, Wang L, Zhao J, Huang Z, Gan R, Wang L. Marked N-acetylaspartate and choline metabolite changes in Parkinson's disease patients with mild cognitive impairment. Parkinsonism Relat Disord 2013;19:329-34. [Crossref] [PubMed]
- Postuma RB, Berg D, Stern M, Poewe W, Olanow CW, Oertel W, Obeso J, Marek K, Litvan I, Lang AE, Halliday G, Goetz CG, Gasser T, Dubois B, Chan P, Bloem BR, Adler CH, Deuschl G. MDS clinical diagnostic criteria for Parkinson's disease. Mov Disord 2015;30:1591-601. [Crossref] [PubMed]
- Goetz CG, Poewe W, Rascol O, Sampaio C, Stebbins GT, Counsell C, Giladi N, Holloway RG, Moore CG, Wenning GK, Yahr MD, Seidl LMovement Disorder Society Task Force on Rating Scales for Parkinson's Disease. Movement Disorder Society Task Force report on the Hoehn and Yahr staging scale: status and recommendations. Mov Disord 2004;19:1020-8. [Crossref] [PubMed]
- Goetz CG, Tilley BC, Shaftman SR, Stebbins GT, Fahn S, Martinez-Martin P, et al. Movement Disorder Society-sponsored revision of the Unified Parkinson's Disease Rating Scale (MDS-UPDRS): scale presentation and clinimetric testing results. Mov Disord 2008;23:2129-70. [Crossref] [PubMed]
- Hamilton M. A rating scale for depression. J Neurol Neurosurg Psychiatry 1960;23:56-62. [Crossref] [PubMed]
- Hamilton M. The assessment of anxiety states by rating. Br J Med Psychol 1959;32:50-5. [Crossref] [PubMed]
- Folstein MF, Folstein SE, McHugh PR. "Mini-mental state". A practical method for grading the cognitive state of patients for the clinician. J Psychiatr Res 1975;12:189-98. [Crossref] [PubMed]
- Nasreddine ZS, Phillips NA, Bédirian V, Charbonneau S, Whitehead V, Collin I, Cummings JL, Chertkow H. The Montreal Cognitive Assessment, MoCA: a brief screening tool for mild cognitive impairment. J Am Geriatr Soc 2005;53:695-9. [Crossref] [PubMed]
- Tombaugh TN, Kozak J, Rees L. Normative data stratified by age and education for two measures of verbal fluency: FAS and animal naming. Arch Clin Neuropsychol 1999;14:167-77. [PubMed]
- Dai XJAPS. A comparison of factor analytic studies among Wechsler Adult Intelligence Scale-Revised in china(WAIS-RC),WAIS AND WAIS-R. 1987.
- Stroop JRJJoEPG. Studies of interference in serial verbal reactions. 1992;121:15-23.
- Chelune G, Curtis G, Heaton R, Curtiss G, Kay G, Talley JJPAR. Wisconsin Cart Sorting Test manual: Revised and expanded. 1993.
- Tomlinson CL, Stowe R, Patel S, Rick C, Gray R, Clarke CE. Systematic review of levodopa dose equivalency reporting in Parkinson's disease. Mov Disord 2010;25:2649-53. [Crossref] [PubMed]
- Sestieri C, Corbetta M, Spadone S, Romani GL, Shulman GL. Domain-general signals in the cingulo-opercular network for visuospatial attention and episodic memory. J Cogn Neurosci 2014;26:551-68. [Crossref] [PubMed]
- Vlagsma TT, Koerts J, Tucha O, Dijkstra HT, Duits AA, van Laar T, Spikman JM. Mental slowness in patients with Parkinson's disease: Associations with cognitive functions? J Clin Exp Neuropsychol 2016;38:844-52. [Crossref] [PubMed]
- Salthouse TA. The processing-speed theory of adult age differences in cognition. Psychol Rev 1996;103:403-28. [Crossref] [PubMed]
- West RL. An application of prefrontal cortex function theory to cognitive aging. Psychol Bull 1996;120:272-92. [Crossref] [PubMed]
- Albinet CT, Boucard G, Bouquet CA, Audiffren M. Processing speed and executive functions in cognitive aging: how to disentangle their mutual relationship? Brain Cogn 2012;79:1-11. [Crossref] [PubMed]
- Fisk JE, Sharp CA. Age-related impairment in executive functioning: updating, inhibition, shifting, and access. J Clin Exp Neuropsychol 2004;26:874-90. [Crossref] [PubMed]
- Keys BA, White DA. Exploring the relationship between age, executive abilities, and psychomotor speed. J Int Neuropsychol Soc 2000;6:76-82. [Crossref] [PubMed]
- Di Caprio V, Modugno N, Mancini C, Olivola E, Mirabella G. Early-stage Parkinson's patients show selective impairment in reactive but not proactive inhibition. Mov Disord 2020;35:409-18. [Crossref] [PubMed]
- Kish SJ, Shannak K, Hornykiewicz O. Uneven pattern of dopamine loss in the striatum of patients with idiopathic Parkinson's disease. Pathophysiologic and clinical implications. N Engl J Med 1988;318:876-80. [Crossref] [PubMed]
- Cools R, Barker RA, Sahakian BJ, Robbins TW. Enhanced or impaired cognitive function in Parkinson's disease as a function of dopaminergic medication and task demands. Cereb Cortex 2001;11:1136-43. [Crossref] [PubMed]
- Poon K. Hot and Cool Executive Functions in Adolescence: Development and Contributions to Important Developmental Outcomes. Front Psychol 2018;8:2311. [Crossref] [PubMed]
- Su L, Blamire AM, Watson R, He J, Hayes L, O'Brien JT. Whole-brain patterns of (1)H-magnetic resonance spectroscopy imaging in Alzheimer's disease and dementia with Lewy bodies. Transl Psychiatry 2016;6:e877. [Crossref] [PubMed]
- Xu H, Zhang H, Zhang J, Huang Q, Shen Z, Wu R. Evaluation of neuron-glia integrity by in vivo proton magnetic resonance spectroscopy: Implications for psychiatric disorders. Neurosci Biobehav Rev 2016;71:563-77. [Crossref] [PubMed]
- Chaudhary S, Kumaran SS, Goyal V, Kalaivani M, Kaloiya GS, Sagar R, Mehta N, Srivastava AK, Jagannathan NR. Frontal lobe metabolic alterations characterizing Parkinson's disease cognitive impairment. Neurol Sci 2021;42:1053-64. [Crossref] [PubMed]
- Ciurleo R, Bonanno L, Di Lorenzo G, Bramanti P, Marino S. Metabolic changes in de novo Parkinson's disease after dopaminergic therapy: A proton magnetic resonance spectroscopy study. Neurosci Lett 2015;599:55-60. [Crossref] [PubMed]
- Schmidt EL, Burge W, Visscher KM, Ross LA. Cortical thickness in frontoparietal and cingulo-opercular networks predicts executive function performance in older adults. Neuropsychology 2016;30:322-31. [Crossref] [PubMed]
- Urenjak J, Williams SR, Gadian DG, Noble M. Proton nuclear magnetic resonance spectroscopy unambiguously identifies different neural cell types. J Neurosci 1993;13:981-9. [Crossref] [PubMed]
- Dautry C, Vaufrey F, Brouillet E, Bizat N, Henry PG, Condé F, Bloch G, Hantraye P. Early N-acetylaspartate depletion is a marker of neuronal dysfunction in rats and primates chronically treated with the mitochondrial toxin 3-nitropropionic acid. J Cereb Blood Flow Metab 2000;20:789-99. [Crossref] [PubMed]
- Firbank MJ, Harrison RM, O'Brien JT. A comprehensive review of proton magnetic resonance spectroscopy studies in dementia and Parkinson's disease. Dement Geriatr Cogn Disord 2002;14:64-76. [Crossref] [PubMed]
- Yang Z, Wan X, Zhao X, Rong Y, Wu Y, Cao Z, Xie Q, Luo M, Liu Y. Brain neurometabolites differences in individuals with subjective cognitive decline plus: a quantitative single- and multi-voxel proton magnetic resonance spectroscopy study. Quant Imaging Med Surg 2021;11:4074-96. [Crossref] [PubMed]
- Frings L, Heimbach B, Meyer PT, Hellwig S. Intrinsic Alertness Is Impaired in Patients with Nigrostriatal Degeneration: A Prospective Study with Reference to [123I]FP-CIT SPECT and [18F]FDG PET. J Alzheimers Dis 2020;78:1721-9. [Crossref] [PubMed]
- Skorvanek M, Goldman JG, Jahanshahi M, Marras C, Rektorova I, Schmand B, van Duijn E, Goetz CG, Weintraub D, Stebbins GT, Martinez-Martin P. members of the MDS Rating Scales Review Committee. Global scales for cognitive screening in Parkinson's disease: Critique and recommendations. Mov Disord 2018;33:208-18. [Crossref] [PubMed]
- Chung SJ, Lee HS, Kim HR, Yoo HS, Lee YH, Jung JH, Baik K, Ye BS, Sohn YH, Lee PH. Factor analysis-derived cognitive profile predicting early dementia conversion in PD. Neurology 2020;95:e1650-9. [Crossref] [PubMed]
- Goetz CG, Poewe W, Rascol O, Sampaio C, Stebbins GT, Counsell C, Giladi N, Holloway RG, Moore CG, Wenning GK, Yahr MD, Seidl LMovement Disorder Society Task Force on Rating Scales for Parkinson's Disease. Movement Disorder Society Task Force report on the Hoehn and Yahr staging scale: status and recommendations. Mov Disord 2004;19:1020-8. [Crossref] [PubMed]
- Zhao YJ, Wee HL, Chan YH, Seah SH, Au WL, Lau PN, Pica EC, Li SC, Luo N, Tan LC. Progression of Parkinson's disease as evaluated by Hoehn and Yahr stage transition times. Mov Disord 2010;25:710-6. [Crossref] [PubMed]