Clinical and imaging risk factors for the persistence of thromboembolism following acute pulmonary embolism
Introduction
Chronic thromboembolic pulmonary hypertension (CTEPH) remains a major cause of chronic pulmonary hypertension, which can lead to right-sided heart failure and death (1,2). CTEPH is the ultimate consequence of hemodynamic compromise and persistent pulmonary perfusion defects that result from a failure to resolve pulmonary vascular obstructions after acute pulmonary embolism. Several pathophysiological mechanisms for thrombus resolution have been suggested based on the evidence derived from studies in patients with established CTEPH (3,4). Some risk factors, including high thrombus load, diagnostic delay, failure to achieve complete thrombus resolution, recurrent symptomatic pulmonary embolism (PE), and pulmonary hypertension or right ventricular compromise at the baseline, have been identified in the development of CTEPH (5,6). However, less is known about the pathophysiological mechanisms for thrombus resolution after acute PE occurs, which may be the precedent stage of CTEPH (6).
A few studies have reported that complete resolution may be achieved in 70–85% of all patients 6 to 12 months after the initial PE diagnosis, with partial resolution occurring in the majority of the remaining patients (7-10). In the study by Sanchez et al. (11), 29% of the patients had perfusion defects during follow-up, and the patients were more likely to have dyspnea, a higher systolic pulmonary arterial pressure, and shorter distance walked during the 6-minute walk test than patients without perfusion defects. The persistence of residual fibrotic blood clots after at least 3 months of effective anticoagulation is a sign of chronic pulmonary thromboembolism (CPTE). Chronic thromboembolic disease (CTED) can be diagnosed in patients with CPTE if they present with exertional dyspnea [New York Heart Association class (NYHA) II, III, or IV], if they have normal pulmonary arterial pressure at rest, and if they have dead-space ventilation or pulmonary hypertension during exercise (13,14).
Computed tomography pulmonary angiography (CTPA) is increasingly used in the diagnosis of CPTE (15). While earlier trials comparing ventilation-perfusion (V/Q) scan and CT in the diagnosis of CPTE showed that V/Q was superior (16), more recent trials have shown comparable sensitivity and specificity between the 2 modalities (17,18). CTED and CTEPH share similar imaging characteristics of CPTE on CTPA, such as eccentric filling defects or the formation of cords or webs in the vessel lumen, which shows partial recanalization of a vessel following thrombosis (6,15). Currently, the early diagnosis of CTEPH remains a challenge, with a median time of 14 months between symptom onset and diagnosis in expert centers (19). Some predisposing factors have been identified for predicting CTEPH during imaging, including large pulmonary arterial thrombi and CTPA findings that suggest pre-existing CPTE (20,21). Although previous studies have mostly focused on predicting CTEPH, the risk factors for incomplete clot resolution after acute PE have not been thoroughly examined (5,22). Predicting the progression of acute pulmonary embolism (APE) to CPTE is essential to monitoring and improving the long-term prognosis of pulmonary embolism. Therefore, we aimed to identify those risk factors associated with the persistence of thromboembolism following APE (i.e., CPTE) to provide predictive information in a clinical setting. We present the following article in accordance with the STROBE reporting checklist (available at https://qims.amegroups.com/article/view/10.21037/qims-21-753/rc).
Methods
Study design
Patients with APE were classified into a CPTE group or a non-CPTE group according to follow-up results. Clinical characteristics, laboratory test results, imaging parameters, and treatment strategies of all APE patients were included in the analysis of the study.
Study patients
Data from cases with newly onset APE in the China-Japan Friendship Hospital from November 2016 to November 2019 were retrospectively collected. The diagnostic criteria for APE were established according to the 2019 ESC Guidelines for the diagnosis and management of acute pulmonary embolism (20). Subsequently, patients who had newly onset APE received three months of regular anticoagulation and were followed up for a minimum of 3 months. A follow-up CTPA was performed to investigate the recanalization of the pulmonary vessels. Additional V/Q scintigraphy or pulmonary angiography was performed when the follow-up CTPA was negative (Figure 1). Patients with positive results on CTPA, V/Q scintigraphy or pulmonary angiography were classified into the CPTE group and others were in the non-CPTE group (Figure 2).


Exclusion criteria were as the follows: (I) the patient’s survival was affected by a disease other than pulmonary embolism during the follow-up, (II) there was a lack of important clinical data or poor image quality of CTPA, and/or (III) the patient was lost to follow-up. The study was conducted in accordance with the Declaration of Helsinki (as revised in 2013). The study was approved by the China-Japan Friendship Hospital Institutional Review Board (Medical Ethics No. 2016-SSW-7), and individual consent for this retrospective analysis was waived.
CTPA
CTPA was performed with a multi-slice CT scanner (Lightspeed VCT/64, GE Healthcare; Philips iCT/256) in the craniocaudal direction using a standard CTPA protocol. Scanning parameters were as follows: tube voltage 100–120 kVP, tube current 100–300 mAs, slice thickness 0.625–1 mm, table speed 39.37 mm/s, gantry rotation time 0.8 s, and reconstruction increment 1–1.25 mm. Soft tissue was used to reconstruct the nucleus. Lopromide (UV, 370 mg/mL) was intravenously injected with a mechanical syringe at a flow rate of 5.0 mL/s. To obtain the best intraluminal contrast enhancement, the automatic bolus-tracking technique positioned the region of interest at the level of the main pulmonary artery with a 100-Hu predefined threshold.
Data collection
We obtained the patients’ clinical data, serological results, and treatment strategy by searching the electronic medical record system (Goodwill E-Health Info Co., Ltd.). Clinical data included age, sex, etiology, risk stratification, presence of recurrent PE, and previous deep vein thrombosis. The etiology of APE includes secondary factors (including recent trauma, fracture, surgical intervention, hospitalization, pregnancy, active cancer, long term immobilization and the use of oral contraceptives or hormone-replacement therapy), primary factors (including deficiency of antithrombin, protein C, or protein S; coagulation factor abnormality; mutation in the factor V Leiden or prothrombin gene; the presence of lupus anticoagulants, and hyperhomocysteinemia), and idiopathic factors (i.e., pulmonary embolism of unknown cause). According to the 2019 European Society of Cardiology (ESC) Guidelines for the diagnosis and management of acute pulmonary embolism (20) and the different treatment options, we divided patients with APE into a low-intermediate risk group and a high-intermediate risk group. The low-intermediate risk group comprised low-risk and intermediate-low risk patients, including those who had signs of right ventricle (RV) dysfunction on echocardiography or CTPA or elevated cardiac biomarkers. The high-intermediate risk group comprised (I) those at intermediate-high risk, including patients with evidence of both RV dysfunction and elevated cardiac biomarker levels in the circulation—particularly a positive cardiac troponin test—and (II) those at high risk, including patients with hemodynamic instability and/or RV dysfunction on echocardiography. In addition, we determined whether APE was recurrent either by outpatient clinic visits or by telephone contact.
Serological examinations consisted of prothrombin time (PT), prothrombin time activity (PTA), fibrinogen (Fbg), activated partial thromboplastin time (APTT), thrombin time (TT), antithrombin III, protein C (PC) activity, protein S (PS) activity, myoglobin (Myo), cardiac troponin-T (cTnT), N-terminal-pro-brain natriuretic peptide (NT-pro-BNP), creatine kinase isoenzyme (CK-MB), hematocrit (HCT), red cell distribution width (RDW), uric acid, creatinine (Cr), homocysteine, and blood type.
All patients underwent CTPA examination at the onset of APE. The CTPA images were independently evaluated using the Picture Archiving and Communication Systems by 2 radiologists with an experience of more than 5 years reading CTPA images. They observed and recorded the proximal location of the pulmonary embolus and categorized the location as the main (main pulmonary trunk, left or right pulmonary artery), lobar, segmental, or subsegmental artery. The presence of pulmonary infarction, pleural effusion, and pericardial effusion was also observed. Measurement and calculation of the parameters included the main pulmonary artery diameter (MPAd), the aorta diameter (Aod), the ratio of MPAd to Aod (MPAd/Aod), the sum of residual segmental pulmonary artery diameter (Sd), the ratio of Sd to MPAd (Sd/MPAd), the right ventricle maximum transverse diameter (RVd), the left ventricular maximum transverse diameter (LVd), the ratio of RVd to LVd (RVd/LVd), and Mastora score (23). Sd referred to the sum of the residual patent diameters of all segmental pulmonary arteries where they branched from the lobar pulmonary artery, including the sum of the residual segmental pulmonary arterial diameters of the left lung (sLd = sL1d + sL2d + sL3d + sL4d+ sL5d + sL6d + sL7d + sL8d) and the right lung (sRd = sR1d + sR2d + sR3d + sR4d + sR5d + sR6d + sR7d + sR8d + sR9d + sR10d), or Sd=sLd + sRd (d: diameter; Figure 3).
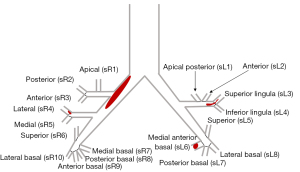
Statistical analysis
Intraclass correlation coefficients (ICC) were used to evaluate the consistency of the measured results. Univariate analysis was performed to the detect differences between the CPTE group and the non-CPTE group. The t test was used for normally distributed data, the Mann-Whitney test was used for nonnormally distributed data, and the continuous corrected chi-square test (when N≥40, and 1≤ T <5) or Fisher’s exact test (when n<40, or T <1) were used for categorical variables. Following this, all variables with a univariate level of significance of less than 0.05 were included in a backward, stepwise multivariate logistic-regression model to detect the risk factors for the CPTE. All calculations were performed with the use of SPSS 20.0 (IBM Corp., Armonk, NY, USA).
Results
A total of 77 patients with APE, including 34 males and 43 females (male:female = 1:1.26) were enrolled in this study, and their mean age was 60 years (range, 25–83 years) with the average follow-up time of 5 months (range, 3–7 months). According to the follow-up results, all APE patients were subsequently divided into the CPTE group (43 cases, 55.84%) and the non-CPTE group (34 cases, 44.16%); 16 patients (16/77, 20.78%) reported dyspnea at the follow-up, including 13 (13/77, 16.88%) in the CPTE group and 3 (3/77, 3.90%) in the non-CPTE group.
Univariate analysis
Clinical and laboratory tests
The etiology, risk stratification, presence of recurrent PE, and previous deep vein thrombosis were compared between the 2 groups (Table 1). We found that only risk stratification was statistically different between the 2 groups and that high-intermediate risk individuals with APE were more likely to progress to CPTE (χ2=8.043; P=0.005).
Table 1
Risk factors | CPTE group (n=43) | Non-CPTE group (n=34) | P value |
---|---|---|---|
Age (year) | 59.19±15.74 | 61.85±14.65 | 0.449 |
Male/female | 23/20 | 11/23 | 0.064 |
Etiology, n (%) | 0.921 | ||
Secondary PE | 33 (76.74) | 25 (73.53) | |
Primary PE | 5 (11.63) | 4 (11.76) | |
Idiopathic PE | 5 (11.63) | 5 (14.71) | |
Recurrent PE, n (%) | 10 (23.26) | 5 (14.71) | 0.347 |
Risk stratification | 0.005 | ||
Low-intermediate risk | 24 (55.81) | 30 (88.24) | |
High-intermediate risk | 19 (44.19) | 4 (11.76) | |
Previous DVT, n (%) | 22 (51.16) | 17 (50.00) | 0.919 |
APE, acute pulmonary embolism; CPTE, chronic pulmonary thromboembolism; PE, pulmonary embolism; DVT, deep vein thrombosis.
The comparison of serological examinations between the 2 groups is listed in Table 2. Results showed that only protein S activity was statistically different between the 2 groups. For the application in clinical practice, we chose the lower bound of the normal value (55%) as the cutoff value and found that APE patients with PS activity <55% were more likely to develop CPTE (χ2=5.551; P=0.018).
Table 2
Risk factors | CPTE group | Non-CPTE group | P value | |||
---|---|---|---|---|---|---|
n | Values | n | Values | |||
PT (s) | 43 | 14.06±2.73 | 34 | 14.63±1.86 | 0.298 | |
PTA (%) | 43 | 86.28±14.62 | 34 | 83.09±16.35 | 0.370 | |
Fbg (g/L) | 43 | 4.22±1.55 | 34 | 4.50±1.59 | 0.441 | |
APTT (s) | 43 | 45.31±9.91 | 34 | 42.40±13.79 | 0.285 | |
TT (s) | 43 | 27.59±35.87 | 34 | 19.89±19.16 | 0.262 | |
D-D (mg/L) | 42 | 3.20±3.02 | 34 | 3.16±3.80 | 0.952 | |
Antithrombin III (%) | 37 | 86.84±11.94 | 26 | 87.88±10.70 | 0.722 | |
PC (<70%) | 35 | 6 | 23 | 3 | 0.349 | |
PS (<55%) | 34 | 12 | 18 | 1 | 0.018 | |
Myo (ng/mL) | 31 | 53.76±64.17 | 27 | 32.74±34.36 | 0.134 | |
cTnT (ng/mL) | 34 | 0.03±0.04 | 25 | 0.04±0.09 | 0.751 | |
NT-pro-BNP (pg/mL) | 32 | 2,087±4,132 | 24 | 1,363±2,792 | 0.462 | |
CK-MB (ng/mL) | 38 | 2.54±3.95 | 30 | 1.38±1.45 | 0.131 | |
Hct (%) | 39 | 33.94±12.83 | 31 | 35.01±8.74 | 0.693 | |
RDW (>15%) | 40 | 3 | 31 | 5 | 0.500 | |
Uric acid (μmol/L) | 38 | 341.4±147.62 | 32 | 307.34±131.80 | 0.315 | |
Homocysteine (μmol/L) | 26 | 18.46±11.94 | 24 | 14.12±7.83 | 0.139 | |
Cr (μmol/L) | 39 | 75.48±25.56 | 32 | 70.84±27.17 | 0.462 |
APE, acute pulmonary embolism; CPTE, chronic pulmonary thromboembolism; PT, prothrombin time; PTA, prothrombin time activity; Fbg, fibrinogen; APTT, activated partial thromboplastin time; TT, thrombin time; D-D, D-dimer; PC, protein C; PS, protein S; Myo, myoglobin; cTnT, cardiac troponin-T; NT-pro-BNP, N-terminal-pro-brain natriuretic peptide; CK-MB, creatine kinase isoenzyme; Hct, hematocrit; RDW, red cell distribution width; Cr, creatinine.
Measures on CTPA
The results of imaging parameters are listed in Table 3. The ICC between the 2 radiologists was 0.848 (95% CI: 0.713–0.920; P<0.001). Patients with APE and an increased Sd/MPAd were more likely to progress to CPTE (t=–2.103; P=0.039). For the application in clinical practice, we took the mean value of Sd/MPAd (1.97) as the cutoff value, and the difference between the two groups was statistically significant when it was ≥1.97 (χ2=3.970; P=0.046). The embolus was located in the main pulmonary artery in 18 cases (23.38%), in the lobar pulmonary artery in 29 cases (37.66%), in the segmental pulmonary artery in 27 cases (35.06%), and in the subsegmental pulmonary artery in 3 cases (3.90%). There was a statistically significant difference in the distribution of emboli and Mastora score between the 2 groups (P<0.001). In addition, 18 patients developed pulmonary infarction (23.38%). Pleural effusion was found in 20 patients and pericardial effusion in 11 patients. However, there were no statistically significant differences between the 2 groups in pulmonary infarction (P=0.110), pericardial effusion (P=0.281), or pleural effusion (P=0.256).
Table 3
Risk factors | CPTE group (n=43) | Non-CPTE group (n=34) | P |
---|---|---|---|
MPAd (mm) | 30.11±4.44 | 20.05±5.97 | 0.965 |
Aod (mm) | 33.44±5.49 | 33.12±5.07 | 0.797 |
MPAd/Aod | 0.92±0.16 | 0.91±0.13 | 0.822 |
Sd/MPAd | 2.05±0.34 | 1.89±0.30 | 0.039 |
Sd/MPAd ≥1.97 | 25 | 12 | 0.046 |
RVd (mm) | 39.03±5.68 | 37.44±7.40 | 0.288 |
LVd (mm) | 43.49±8.44 | 41.08±5.51 | 0.155 |
RVd/LVd | 0.93±0.22 | 0.91±0.13 | 0.706 |
Mastora score | 43.86±29.46 | 19.79±21.11 | <0.001 |
Location of embolism | <0.001 | ||
Subsegmental | 0 | 3 | |
Segmental | 8 | 19 | |
Lobar | 19 | 10 | |
Main | 16 | 2 | |
Pulmonary infarction | 13 | 5 | 0.110 |
Pleural effusion | 9 | 11 | 0.256 |
Pericardial effusion | 4 | 7 | 0.281 |
APE, acute pulmonary embolism; CPTE, chronic pulmonary thromboembolism; MPAd, the main pulmonary artery diameter; Aod, the aorta diameter; MPAd/Aod, the ratio of MPAd to Aod; Sd, sum of residual segment pulmonary artery diameter; Sd/MPAd, the ratio of Sd to MPAd; RVd, the right ventricle maximum transverse diameter; LVd, the left ventricular maximum transverse diameter; RVd/LVd, the ratio of RVd to LVd.
Therapy
All 77 patients received 3 months of uninterrupted anticoagulation therapy prior to repeat imaging. This included 4 treatment strategies: oral anticoagulation only (5 cases, 6.5%), intravenous anticoagulation (66 cases, 85.7%), combined anticoagulation with thrombolysis (3 cases, 3.9%), and combined anticoagulation with interventional thrombolysis (3 cases, 3.9%). However, there was no statistically significant difference between the 2 groups in treatment option (P=0.381; Table 4).
Table 4
Treatment | CPTE group (n=43), n (%) | Non-CPTE group (n=34), n (%) | P value |
---|---|---|---|
Oral anticoagulation | 3 (7.0) | 2 (5.9) | 0.381 |
Intravenous anticoagulation | 36 (83.7) | 30 (88.2) | |
Combined anticoagulation with thrombolysis | 1 (2.3) | 2 (5.9) | |
Combined anticoagulation with interventional thrombolysis | 3 (7.0) | 0 (0.0) |
APE, acute pulmonary embolism; CPTE, chronic pulmonary thromboembolism.
Summary
Univariate analysis indicated the risk factors that may affect the progression of APE to CPTE were as follows: (I) risk stratification—high-intermediate risk individuals with APE were more likely to progress to CPTE (χ2=8.043; P=0.005); (II) protein S activity—55% was selected as the cutoff value in this study, which found that APE patients with protein S activity <55% were more likely to develop CPTE (χ2=5.551; P=0.018); (III) Sd/MPAd—APE patients with increased Sd/MPAd were more likely to progress to CPTE (t=–2.103; P=0.039), and the difference between the 2 groups was statistically significant when it was ≥1.97 (χ2=3.970; P =0.046); (IV) Mastora score—patients with higher scores were more likely to progress to CPTE (U=362.500; P<0.001); and (V) embolus location—there was a statistically significant difference in the distribution of emboli between the 2 groups (χ2=16.969; P<0.001; Tables 1-4).
However, there were no statistically significant differences between the 2 groups in age, gender, etiology, or treatment options (P>0.05).
Multivariate regression analysis
Based on the results of the univariate analysis, variables were selected to perform multivariate logistic-regression analysis to determine the independent risk factors for progression to CPTE. The variables included were age at onset, etiology, risk stratification, protein S activity, Sd/MPAd, Mastora score, embolus location, and therapeutic approaches. For convenience, the embolus location was simplified to be in the central pulmonary artery (main pulmonary trunk, left or right pulmonary artery or lobar artery; 35 cases in the CPTE group and 12 cases in the non-CPTE group) and the peripheral pulmonary artery (segmental or subsegmental pulmonary artery; 8 cases in the CPTE group and 22 cases in the non-CPTE group).
We performed multivariable risk factor analysis and found that protein S activity <55%, Sd/MPAd ≥1.97, and the embolus being located in the central pulmonary artery were independent risk factors associated with the development of CPTE (Table 5).
Table 5
Risk factors | OR (95% CI) | P value |
---|---|---|
Protein S activity | ||
≥55% | 1.00 | |
<55% | 18.41 (1.45, 234.05) | 0.025 |
Sd/MPAd | ||
<1.97 | 1.00 | |
≥1.97 | 5.43 (1.47, 20.06) | 0.011 |
Embolus location | ||
Peripheral | 1.00 | |
Central | 13.21 (3.43, 50.88) | <0.001 |
CPTE, chronic pulmonary thromboembolism; Sd/MPAd, the ratio of sum of residual segmental pulmonary artery diameter to the main pulmonary artery diameter; OR, odds ratio.
Discussion
In this retrospective study, the patients with APE were followed up and investigated to explore those risk factors associated with the persistence of thromboembolism after APE. We finally determined that PS activity <55%, Sd/MPAd ≥1.97, and the embolus being located in the central pulmonary artery were independent risk factors for the progression of APE to CPTE.
There exist a few studies regarding the risk factors for CTEPH development after APE (21,24). It is well known that CTEPH is an important long-term complication and cause of death in patients with pulmonary embolism. Although the pulmonary artery pressure of CPTE is normal, pulmonary artery remodeling and hemodynamic changes may occur (25). Therefore, patients with CPTE are suitable for strict surveillance and treatment to improve the prognosis of pulmonary embolism, and thus knowledge concerning the development of CPTE after APE is essential for clinical observation. Currently, the true incidence of CPTE is unknown, and the rate in this study was 55.84%, which was higher than the 15–30% reported in the literature (11,26). The difference may be attributed to the shorter interval of follow-up in our study relative to the 6–12 months used in prior studies. However, it is worth noting that the resolution of thrombi appears to reach a plateau phase after 3 months of adequate treatment, as the remaining clots have been remodeled into permanent fibrous scars (10,27,28).
To our knowledge, this is the first study to propose a new imaging quantitative parameter, Sd/MPAd, for predicting persistent thromboemboli following APE. One noteworthy finding was that Sd/MPAd ≥1.97 was an independent risk factor for CPTE. We have proposed the calculation of the parameter based on the pathophysiological mechanism of the pulmonary vascular obstruction, which is described in detail in the Methods section. Sd was used to represent the total residual cross-sectional area of segmental vessels, and Sd/MPAd was used in order to reduce individual differences as much as possible. According to Poiseuille’s law, when the emboli block some of the pulmonary vessels, hypoxia, mechanical stimulation, and other factors that result from the pulmonary embolism cause vasoconstriction and decrease the total cross-sectional area, which leads to an increase in vascular resistance and a decrease in the regional blood flow. Blood stasis that results from vessel obstruction increases the release of tissue plasminogen activator and activates the fibrinolytic system, which are the accelerating factors for dissolving the thrombosis (29). Partially blocked vessels may not be able to thoroughly activate the fibrinolytic system, so they have more potential for residual thrombi. This also explains to some extent why the Mastora score was not an independent risk factor. It may be possible that thrombolysis is mainly determined by the hemodynamics-related activation of the fibrinolytic system rather than by the clot burden, so which should not be deemed as the counterpart of the Mastora score. The Mastora score is an index that shows obstruction, while Sd/MPAd is suggestive of both residual lumens and secondary vessel reaction to the impaired hemodynamics. Overall, according to our results, an Sd/MPAd ≥1.97 is an independent risk factor of incomplete resolution and organization of thrombus in patients following APE. However, this index should be widely tested in further research.
We also found that the embolus being located in the central pulmonary artery to be an independent risk factor of CPTE, which is consistent with findings by Guérin et al. (30). However, the results of previous studies on the effect of embolus location have been inconsistent. Yu et al. (31) reported that an embolus located in the segmental or subsegmental pulmonary arteries was more likely to be associated with an increased risk of CTEPH, while Tosun et al. (32) reported that embolus location had no effect on this risk. We speculate that an embolus located in the central pulmonary artery is usually overextended and is difficult to dissolve completely at some places, such as branching sites. Furthermore, in the univariate model, we found that RVd/LVd was not a risk factor for CPTE. Guérin et al. (30) reported that APE patients with increased RVd/LVd were more likely to develop CTEPH. Further research designed to explore the relationship between the above risk factors and the prognosis of APE is required.
As for serological markers, PS activity <55% was found to be an independent risk factor for CPTE in this study. A previous study showed there to be no association between a deficiency of antithrombin, protein C, or protein S and CTEPH (33). PS, a vitamin K-dependent plasma protein, is an essential cofactor of the coagulation proteins involved in hemostasis and thrombosis. People with inherited disorders related to the production or function of PS may have a 10-fold risk for venous thrombosis (34-36). In a population-based study, Pintao et al. (37) demonstrated that patients at risk of venous thrombosis could be identified at levels <0.10th percentile of free protein S. During the short period after the onset of thrombosis or intaking warfarin, some patients show a decrease of PS activity due to consumption, with a variable extent and period. PS might regulate the process of thrombus dissolution via a series of cofactors (38,39). Therefore, it is worth investigating the role of reduced PS activity in the development of chronic embolus.
There were several limitations to this retrospective study. First, as Sd/MPAd is a new risk factor for predicting CPTE, whether it is related to persistent functional impairment needs to be tested. Moreover, the sample size of this study was insufficient for a study of CTED, and thus a large, multicenter prospective study should be conducted to further verify the role of the imaging parameters. Second, the follow-up interval varied in this study although our patients underwent a standard anticoagulant therapy more than 3 months following APE. Recently, increasing attention has been paid to the need for follow-up care to recognize and prevent long-term sequelae. However, when and how best to screen and evaluate patients for post-PE syndrome remains an area of active research (5). Nonetheless, the difference in the follow-up interval might have had an impact on the grouping of CPTE patients. Third, the prognosis of CPTE is mainly related to the hemodynamic changes of pulmonary blood vessels, and persistent thromboemboli may not definitely represent hemodynamic impairments. However, our findings provide novel insights concerning the hemodynamic and pathophysiological effects on the treatment outcome.
In conclusion, the protein S activity, Sd/MPAd, and the location of embolus at APE onset may suggest whether or not anticoagulant therapy can achieve a complete resolution of PE.
Acknowledgments
Funding: This work was supported by the Elite Medical Professionals Project of China-Japan Friendship Hospital (No. ZRJY2021-BJ02).
Footnote
Reporting Checklist: The authors have completed the STROBE reporting checklist. Available at https://qims.amegroups.com/article/view/10.21037/qims-21-753/rc
Conflicts of Interest: All authors have completed the ICMJE uniform disclosure form (available at https://qims.amegroups.com/article/view/10.21037/qims-21-753/coif). The authors have no conflicts of interest to declare.
Ethical Statement: The authors are accountable for all aspects of the work in ensuring that questions related to the accuracy or integrity of any part of the work are appropriately investigated and resolved. The study was conducted in accordance with the Declaration of Helsinki (as revised in 2013). The study was approved by the China-Japan Friendship Institutional Review Board (Medical Ethics No. 2016-SSW-7), and individual consent for this retrospective analysis was waived.
Open Access Statement: This is an Open Access article distributed in accordance with the Creative Commons Attribution-NonCommercial-NoDerivs 4.0 International License (CC BY-NC-ND 4.0), which permits the non-commercial replication and distribution of the article with the strict proviso that no changes or edits are made and the original work is properly cited (including links to both the formal publication through the relevant DOI and the license). See: https://creativecommons.org/licenses/by-nc-nd/4.0/.
References
- Kim NH, Delcroix M, Jais X, Madani MM, Matsubara H, Mayer E, Ogo T, Tapson VF, Ghofrani HA, Jenkins DP. Chronic thromboembolic pulmonary hypertension. Eur Respir J 2019;53:1801915. [Crossref] [PubMed]
- McNeil K, Dunning J. Chronic thromboembolic pulmonary hypertension (CTEPH). Heart 2007;93:1152-8. [Crossref] [PubMed]
- Wagenvoort CA. Pathology of pulmonary thromboembolism. Chest 1995;107:10S-7S. [Crossref] [PubMed]
- Rosenberg RD, Aird WC. Vascular-bed--specific hemostasis and hypercoagulable states. N Engl J Med 1999;340:1555-64. [Crossref] [PubMed]
- Fabyan KD, Holley AB. Postpulmonary embolism syndrome. Curr Opin Pulm Med 2021;27:335-41. [Crossref] [PubMed]
- Klok FA, van der Hulle T, den Exter PL, Lankeit M, Huisman MV, Konstantinides S. The post-PE syndrome: a new concept for chronic complications of pulmonary embolism. Blood Rev 2014;28:221-6. [Crossref] [PubMed]
- Alonso-Martínez JL, Anniccherico-Sánchez FJ, Urbieta-Echezarreta MA, García-Sanchotena JL, Herrero HG. Residual pulmonary thromboemboli after acute pulmonary embolism. Eur J Intern Med 2012;23:379-83. [Crossref] [PubMed]
- Cosmi B, Nijkeuter M, Valentino M, Huisman MV, Barozzi L, Palareti G. Residual emboli on lung perfusion scan or multidetector computed tomography after a first episode of acute pulmonary embolism. Intern Emerg Med 2011;6:521-8. [Crossref] [PubMed]
- Golpe R, de Llano LA, Castro-Añón O, Vázquez-Caruncho M, González-Juanatey C, Fariñas MC. Long-term outcome of patients with persistent vascular obstruction on computed tomography pulmonary angiography 6 months after acute pulmonary embolism. Acta Radiol 2012;53:728-31. [Crossref] [PubMed]
- Korkmaz A, Ozlu T, Ozsu S, Kazaz Z, Bulbul Y. Long-term outcomes in acute pulmonary thromboembolism: the incidence of chronic thromboembolic pulmonary hypertension and associated risk factors. Clin Appl Thromb Hemost 2012;18:281-8. [Crossref] [PubMed]
- Sanchez O, Helley D, Couchon S, Roux A, Delaval A, Trinquart L, Collignon MA, Fischer AM, Meyer G. Perfusion defects after pulmonary embolism: risk factors and clinical significance. J Thromb Haemost 2010;8:1248-55. [Crossref] [PubMed]
- Wang L, Liu M, Zhang PY, Dai JZ, Ma HY, Tao XC, Xie WM, Wan J, Jing A. Analysis of right ventricular flow with 4-dimensional flow cardiovascular magnetic resonance imaging in patients with pulmonary arterial hypertension. Quant Imaging Med Surg 2021;11:3655-65. [Crossref] [PubMed]
- Le Gal G, Carrier M, Castellucci LA, Cuker A, Hansen JB, Klok FA, Langlois NJ, Levy JH, Middeldorp S, Righini M, Walters SISTH CDE Task Force. Development and implementation of common data elements for venous thromboembolism research: on behalf of SSC Subcommittee on official Communication from the SSC of the ISTH. J Thromb Haemost 2021;19:297-303. [Crossref] [PubMed]
- Klok FA, Delcroix M, Bogaard HJ. Chronic thromboembolic pulmonary hypertension from the perspective of patients with pulmonary embolism. J Thromb Haemost 2018;16:1040-51. [Crossref] [PubMed]
- Haramati A, Haramati LB. Imaging of Chronic Thromboembolic Disease. Lung 2020;198:245-55. [Crossref] [PubMed]
- Tunariu N, Gibbs SJ, Win Z, Gin-Sing W, Graham A, Gishen P, Al-Nahhas A. Ventilation-perfusion scintigraphy is more sensitive than multidetector CTPA in detecting chronic thromboembolic pulmonary disease as a treatable cause of pulmonary hypertension. J Nucl Med 2007;48:680-4. [Crossref] [PubMed]
- He J, Fang W, Lv B, He JG, Xiong CM, Liu ZH, He ZX. Diagnosis of chronic thromboembolic pulmonary hypertension: comparison of ventilation/perfusion scanning and multidetector computed tomography pulmonary angiography with pulmonary angiography. Nucl Med Commun 2012;33:459-63. [Crossref] [PubMed]
- Miles S, Rogers KM, Thomas P, Soans B, Attia J, Abel C, Holt E, D'Este CA, Hensley MJ. A comparison of single-photon emission CT lung scintigraphy and CT pulmonary angiography for the diagnosis of pulmonary embolism. Chest 2009;136:1546-53. [Crossref] [PubMed]
- Pepke-Zaba J, Jansa P, Kim NH, Naeije R, Simonneau G. Chronic thromboembolic pulmonary hypertension: role of medical therapy. Eur Respir J 2013;41:985-90. [Crossref] [PubMed]
- Konstantinides SV, Meyer G, Becattini C, Bueno H, Geersing GJ, Harjola VP, et al. 2019 ESC Guidelines for the diagnosis and management of acute pulmonary embolism developed in collaboration with the European Respiratory Society (ERS): The Task Force for the diagnosis and management of acute pulmonary embolism of the European Society of Cardiology (ESC). Eur Respir J 2019;54:1901647. [Crossref] [PubMed]
- Delcroix M, Kerr K, Fedullo P. Chronic Thromboembolic Pulmonary Hypertension. Epidemiology and Risk Factors. Ann Am Thorac Soc 2016;13:S201-6. [Crossref] [PubMed]
- Morris TA. Why acute pulmonary embolism becomes chronic thromboembolic pulmonary hypertension: clinical and genetic insights. Curr Opin Pulm Med 2013;19:422-9. [Crossref] [PubMed]
- Mastora I, Remy-Jardin M, Masson P, Galland E, Delannoy V, Bauchart JJ, Remy J. Severity of acute pulmonary embolism: evaluation of a new spiral CT angiographic score in correlation with echocardiographic data. Eur Radiol 2003;13:29-35. [Crossref] [PubMed]
- Zhang M, Wang N, Zhai Z, Zhang M, Zhou R, Liu Y, Yang Y. Incidence and risk factors of chronic thromboembolic pulmonary hypertension after acute pulmonary embolism: a systematic review and meta-analysis of cohort studies. J Thorac Dis 2018;10:4751-63. [Crossref] [PubMed]
- Lang IM, Marsh JJ, Olman MA, Moser KM, Loskutoff DJ, Schleef RR. Expression of type 1 plasminogen activator inhibitor in chronic pulmonary thromboemboli. Circulation 1994;89:2715-21. [Crossref] [PubMed]
- den Exter PL, van Es J, Kroft LJ, Erkens PM, Douma RA, Mos IC, Jonkers G, Hovens MM, Durian MF, ten Cate H, Beenen LF, Kamphuisen PW, Huisman MVPrometheus Follow-Up Investigators. Thromboembolic resolution assessed by CT pulmonary angiography after treatment for acute pulmonary embolism. Thromb Haemost 2015;114:26-34. [Crossref] [PubMed]
- Aghayev A, Furlan A, Patil A, Gumus S, Jeon KN, Park B, Bae KT. The rate of resolution of clot burden measured by pulmonary CT angiography in patients with acute pulmonary embolism. AJR Am J Roentgenol 2013;200:791-7. [Crossref] [PubMed]
- Begic A, Jögi J, Hadziredzepovic A, Kucukalic-Selimović E, Begovic-Hadzimuratovic S, Bajc M. Tomographic ventilation/perfusion lung scintigraphy in the monitoring of the effect of treatment in pulmonary embolism: serial follow-up over a 6-month period. Nucl Med Commun 2011;32:508-14. [Crossref] [PubMed]
- Singh S, Houng AK, Reed GL. Venous stasis-induced fibrinolysis prevents thrombosis in mice: role of α2-antiplasmin. Blood 2019;134:970-8. [Crossref] [PubMed]
- Guérin L, Couturaud F, Parent F, Revel MP, Gillaizeau F, Planquette B, Pontal D, Guégan M, Simonneau G, Meyer G, Sanchez O. Prevalence of chronic thromboembolic pulmonary hypertension after acute pulmonary embolism. Prevalence of CTEPH after pulmonary embolism. Thromb Haemost 2014;112:598-605. [Crossref] [PubMed]
- Yu Y, Yang L, Zhang Y, Dong L, Xia J, Zhu N, Han X, Fang L, Chai Y, Niu M, Liu L, Yang X, Qu S, Li S. Incidence and risk factors of chronic thromboembolic pulmonary hypertension in patients with diagnosis of pulmonary embolism for the first time in real world. Clin Respir J 2018;12:2551-8. [Crossref] [PubMed]
- Tosun H, Kırkıl G, Deveci F, Dağlı N, Özcan M, Telo S. The Incidence and Related Risk Factors of Chronic Thromboembolic Pulmonary Hypertension after Acute Pulmonary Embolism. Turk Thorac J 2016;17:53-8. [Crossref] [PubMed]
- Lang IM, Pesavento R, Bonderman D, Yuan JX. Risk factors and basic mechanisms of chronic thromboembolic pulmonary hypertension: a current understanding. Eur Respir J 2013;41:462-8. [Crossref] [PubMed]
- Castoldi E, Maurissen LF, Tormene D, Spiezia L, Gavasso S, Radu C, Hackeng TM, Rosing J, Simioni P. Similar hypercoagulable state and thrombosis risk in type I and type III protein S-deficient individuals from families with mixed type I/III protein S deficiency. Haematologica 2010;95:1563-71. [Crossref] [PubMed]
- Lijfering WM, Mulder R, ten Kate MK, Veeger NJ, Mulder AB, van der Meer J. Clinical relevance of decreased free protein S levels: results from a retrospective family cohort study involving 1143 relatives. Blood 2009;113:1225-30. [Crossref] [PubMed]
- Ten Kate MK, Platteel M, Mulder R, Terpstra P, Nicolaes GA, Reitsma PH, van der Steege G, van der Meer J. PROS1 analysis in 87 pedigrees with hereditary protein S deficiency demonstrates striking genotype-phenotype associations. Hum Mutat 2008;29:939-47. [Crossref] [PubMed]
- Pintao MC, Ribeiro DD, Bezemer ID, Garcia AA, de Visser MC, Doggen CJ, Lijfering WM, Reitsma PH, Rosendaal FR. Protein S levels and the risk of venous thrombosis: results from the MEGA case-control study. Blood 2013;122:3210-9. [Crossref] [PubMed]
- Chattopadhyay R, Sengupta T, Majumder R. Inhibition of intrinsic Xase by protein S: a novel regulatory role of protein S independent of activated protein C. Arterioscler Thromb Vasc Biol 2012;32:2387-93. [Crossref] [PubMed]
- Dahlbäck B. Pro- and anticoagulant properties of factor V in pathogenesis of thrombosis and bleeding disorders. Int J Lab Hematol 2016;38:4-11. [Crossref] [PubMed]