Predictive value of the echocardiographic noninvasive myocardial work index for left ventricular reverse remodeling in patients with multivessel coronary artery disease after percutaneous coronary intervention
Introduction
Coronary artery disease (CAD) is a common type of cardiovascular disease with an increasing incidence and, in recent years, has shown a trend toward affecting younger people. The 1-year mortality of patients with multiple vessel CAD is significantly higher than that for patients with single-vessel disease (1), and multiple vessel CAD is often accompanied by cardiac enlargement, heart failure, arrhythmia, and other complications.
CAD can lead to left ventricular (LV) remodeling, which, in adverse cases, has been associated with heart failure and increased mortality (2). Percutaneous coronary intervention (PCI) is an effective method for coronary revascularization, which can increase myocardial blood and oxygen supply, retract an enlarged left ventricle, reverse ventricular remodeling, and improve prognosis (3,4). Accordingly, accurate evaluation of LV reverse remodeling plays an important role in clinical practice.
LV global longitudinal strain (GLS), derived from two-dimensional (2D) speckle tracking echocardiography, can predict LV adverse remodeling and subsequent cardiac events in patients with acute myocardial infarction treated with PCI (5). However, the LV GLS is load-dependent and cannot truly represent LV systolic function (6). The noninvasive myocardial work index (NIMWI), measured with echocardiogram pressure-strain loops (PSLs), is a new technology that is based on 2D speckle tracking technology and accounts for loading conditions. The NIMWI integrates the relationship between LV deformation and pressure in a cardiac cycle, and it can quantitatively evaluate the LV systolic function in a noninvasive, convenient, and rapid manner (7,8). Russell et al. (9) reported a strong correlation between LV work evaluated using noninvasive echocardiography in the LV NIMWI and cardiac work measured with invasive catheterization.
The aim of this study was to evaluate the predictive value of NIMWIs for LV reverse remodeling in patients with multivessel CAD after PCI. We hypothesized that GCW, among the NIMWIs, may be the major predictor of LV reverse remodeling in patients with multivessel CAD after PCI. We present the following article in accordance with STROBE (Strengthening the Reporting of Observational Studies in Epidemiology) reporting checklist (available at https://qims.amegroups.com/article/view/10.21037/qims-21-1066/rc).
Methods
Study population
The study was conducted in accordance with the Declaration of Helsinki (as revised in 2013). The prospective study was approved by the ethics committee of the Henan Provincial People’s Hospital (Zhengzhou, China; approval No. 202031), and informed consent was obtained from all the patients. A total of 130 potentially eligible participants were identified based on symptoms including chest tightness, chest pain, and palpitations, but 32 patients were not treated with PCI because the degree of coronary artery stenosis was <70% as determined by coronary angiography. Of the 98 eligible participants enrolled in the study, 4 were missing an echocardiogram at follow-up, and 6 had insufficient image quality, which precluded quantitative ultrasound analysis. The remaining 88 participants, who were followed up until 3 months after PCI, had adequate image quality for echocardiography analysis (Figure 1).
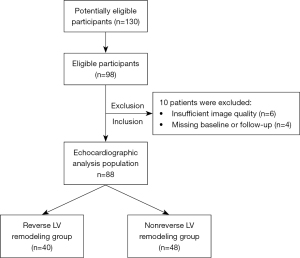
A total of 88 consecutive patients with CAD between 26 and 77 years of age (56.9±14.1 years) were prospectively identified at the Henan Provincial People’s Hospital between May 2019 and May 2021. Among this group, 77 were male, 11 were female, and 57 patients had acute coronary syndrome, which included 5 patients with ST-segment elevation myocardial infarction and 31 patients with chronic coronary syndrome (10). Patients with CAD were diagnosed using coronary angiography with ≥70% stenosis of 2 or more main coronary arteries (11). In accordance with the European PCI guidelines, fractional flow reserve (FFR) measurements were performed to determine the presence of myocardial ischemia in borderline lesions with no evidence of ischemia and coronary angiography showing 50–90% stenosis (12). Coronary angiography was performed via radial or femoral access. PCI was performed on multiple lesions in the CAD group. Early LV reverse remodeling was defined as a decrease in the LV end diastolic volume (LVEDV) ≥15% from the baseline LVEDV at 3 months after PCI in patients with multivessel CAD (13).
The inclusion criteria for the patients with CAD were a confirmed diagnosis of multivessel CAD by coronary angiography. The exclusion criteria for the patients with CAD were coronary artery chronic total occlusions, hypertension, significant valvular heart disease (valvular stenosis or > mild function regurgitation), hypertrophic cardiomyopathy, dilated cardiomyopathy, atrial fibrillation at the time of examination, left bundle branch block, diabetes, pulmonary heart diseases, unclear images from diagnostic procedures, and surgical indications for coronary artery bypass grafting. All patients with CAD were examined using ultrasonography 1 week before PCI and 3 months after PCI.
Transthoracic echocardiography
The ultrasound instrument GE Vivid E95 (GE Vingmed Ultrasound, Horten, Norway) and 2D M5Sc-D (Integrity Medical Service, Englewood, CO, USA) with a frequency of 1.4–4.6 MHz were used in this study. The real-time blood pressure (BP) of the participants in a lying position was measured with the cuff method 5–10 min before echocardiography, and patients’ data were recorded. An electrocardiogram was administered for patients synchronously while in the left lying position, and the 2D probe was enabled. The images of 3 consecutive cardiac cycles were collected from the section of the apical LV long axis, the 4-chamber heart section, and the 2-chamber heart section. The images were exported to an EchoPAC workstation (EchoPAC Version 203, GE Vingmed Ultrasound) in the original data format. Transthoracic echocardiography was used to measure aortic valve forward velocity, mitral orifice velocity, interventricular septal thickness in diastole (IVSD), left ventricular posterior wall thickness in diastole (LVPWD), left ventricular end diastolic volume index (LVEDVI), left ventricular end systolic volume index (LVESVI), and left ventricular ejection fraction (LVEF) with the modified biplane Simpson method according to the guidelines of the American Society of Echocardiography (ASE)/European Association of Cardiovascular Imaging (EACVI) issued in 2015 (14). Left ventricular mass (LVM) was calculated according to the following formula: LVM (g) = {1.04 × [IVS (cm) + LVDd (cm) + LVPW (cm)]3 − [LVDd (cm3)]} × 0.8 + 0.6 (15). The left ventricular mass index (LVMI) was obtained, and the ratio between the early diastolic velocity of the mitral valve (E) and the speed of movement of the mitral annulus at the interventricular septum (e’) was determined.
Analysis of myocardial work
Offline analyses of the stored images in the EchoPAC workstation were conducted. The ejection phase and the duration of the LV isovolumic systole were determined in accordance with the opening and closing of the aortic and the mitral valves. Automatic functional imaging (AFI) was used to describe the collected images of the endocardium in the LV long axis and the 4- and 2-chamber heart sections. This software can automatically track the LV endocardium and epicardium. The envelope of the endocardium and epicardium was manually adjusted if automatic tracking could not sufficiently ensure satisfactory tracking and to obtain the strain results of the global and segmental left ventricle. The BP values measured with the upper arm cuff method were entered into the myocardial work menu (Figure 2) to display the LV PSL curve and calculate the myocardial work index parameters, including the global work index (GWI), global constructive work (GCW), global wasted work (GWW), global work efficiency (GWE), and GLS. In the present study, the absolute value of GLS was used for analysis. LV PSL refers to the total work of the LV myocardium from the closure to the opening of the mitral valve; GWI refers to the area of the global LV PSL; GCW refers to the work generated from shortening of the myocytes during systole and their lengthening during isovolumic relaxation. By contrast, GWW refers to the work generated from the myocardial lengthening during systole and shortening during the isovolumic relaxation; GWE can be computed with the following formula: GWE = GCW/(GCW + GWW).
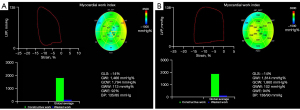
Statistical analysis
SPSS 22.0 software (IBM Corp., Armonk, NY, USA) was used to conduct statistical analyses. Continuous data, when normally distributed, are expressed as mean ± SD, while categorical data are expressed as frequencies and percentages. Categorical variables were compared with the χ2 test. A paired sample t-test was used for comparison of measurements before and after PCI. The independent samples t-test was conducted for the between-group comparison of measurement data. Univariate logistic regression was used to determine variables correlated with LV reverse remodeling, and parameters with P<0.1 in univariate analysis were incorporated into the multivariate analysis to determine the independent associations (P<0.05). Multicollinearity between variables was not allowed in the multivariate analysis. Receiver operating characteristic (ROC) curve analysis and the Youden index were used to assess optimal cutoff points in the prediction of early LV reverse remodeling. Ten cases were selected randomly from both the reverse LV remodeling group and the nonreverse LV remodeling group to test the repeatability of the cardiac work parameters with Bland-Altman plot analysis. All tests were 2-sided, and a P value <0.05 was considered statistically significant.
Results
Study population and clinical characteristics
The time interval between transthoracic echocardiography and myocardial work analysis in the patients with CAD was approximately 160±9 s, and no clinical interventions were required.
No significant difference was observed between the reverse LV and nonreverse LV remodeling groups in age, sex, height, weight, body surface area, heart rate, diastolic BP, total cholesterol (TC), or low-density lipoprotein (LDL; P>0.05); however, the systolic BP was higher in the reverse LV remodeling group than in the nonreverse LV remodeling group (P<0.05; Table 1).
Table 1
Variable | All patients (n=88) | Reverse LV remodeling group (n=40) | Nonreverse LV remodeling group (n=48) | P value |
---|---|---|---|---|
Age (years) | 56.2±14.1 | 54.5±13.6 | 57.6±14.5 | 0.298 |
Male, n (%) | 77 (87.5) | 34 (85.0) | 43 (89.6) | 0.517 |
Height (cm) | 167.4±7.1 | 168.0±7.5 | 166.8±6.8 | 0.454 |
Weight (kg) | 67.8±11.0 | 65.9±9.8 | 69.3±11.8 | 0.158 |
Body surface area (m2) | 1.7±0.2 | 1.7±0.2 | 1.8±0.2 | 0.298 |
Heart rate (bpm) | 74.2±10.9 | 74.7±9.5 | 73.7±12.1 | 0.680 |
Systolic pressure (mmHg) | 127.4±10.5 | 130.1±7.6 | 125.2±12.0 | 0.021 |
Diastolic pressure (mmHg) | 83.2±8.3 | 82.9±7.5 | 83.4±9.1 | 0.757 |
Smoker, n (%) | 45 (51.1) | 18 (45.0) | 27 (56.2) | 0.293 |
TC (mmol/L) | 4.2±0.7 | 4.2±0.8 | 4.2±0.6 | 0.657 |
LDL (mmol/L) | 2.9±0.6 | 2.8±0.5 | 3.0±0.7 | 0.201 |
LAD, n (%) | 70 (79.5) | 32 (80.0) | 38 (79.2) | 0.999 |
LCx, n (%) | 61 (69.3) | 28 (70.0) | 33 (68.8) | 0.999 |
RCA, n (%) | 66 (75.0) | 30 (75.0) | 36 (75.0) | 0.999 |
Stents placed, n | 3.1±0.8 | 3.3±0.8 | 3.0±0.7 | 0.124 |
Aspirin, n (%) | 84 (95.5) | 38 (95.0) | 46 (95.8) | 0.852 |
Beta blocker, n (%) | 80 (90.9) | 36 (90.0) | 44 (91.6) | 0.787 |
Statin, n (%) | 88 (100.0) | 40 (100.0) | 48 (100.0) | 1.000 |
ACEI or ARB, n (%) | 83 (94.3) | 38 (95.0) | 45 (93.7) | 0.801 |
Data are expressed as n (%) or mean ± SD. LV, left ventricular; TC, total cholesterol; LDL, low-density lipoprotein; LAD, left anterior descending coronary artery; LCx, left circumflex artery; RCA, right coronary artery; ACEI, angiotensin-converting-enzyme inhibitor; ARB, angiotensin receptor blocker.
Regarding the conventional echocardiography parameters, significantly higher LVEDVI, LVESVI, LVMI, and E/e’ and lower LVEF were observed in the reverse LV remodeling group compared with the nonreverse LV remodeling group (P≤0.05), whereas no significant differences were observed in IVSD, LVPWD, and septal e’ between the groups 1 week before PCI (P>0.05). The LVEDVI, LVESVI, LVMI, and E/e’ of the reverse LV remodeling group 3 months after PCI were significantly lower and the LVEF was higher than the values obtained 1 week before PCI. Moreover, the LVEDVI, LVESVI, and LVMI of the nonreverse LV remodeling group 3 months after PCI were significantly lower than the values obtained 1 week before PCI. Nevertheless, the absolute value of the differences in LVEDVI, LVESVI, LVMI, and LVEF between 3 months after PCI and 1 week before PCI in the reverse LV remodeling group was higher than that in the nonreverse LV remodeling group (P<0.05; Table 2).
Table 2
Variable | All patients (n=88) | Reverse LV remodeling group (n=40) | Nonreverse LV remodeling group (n=48) | |||||
---|---|---|---|---|---|---|---|---|
1 week before PCI | 3 months after PCI | ∆t | 1 week before PCI | 3 months after PCI | ∆t | |||
LVEDVI (mL/m2) | 76.9±15.2 | 85.2±9.3†‡ | 68.3±8.6 | −16.9±3.2† | 70.1±15.8‡ | 67.3±14.6 | −2.8±5.4 | |
LVESVI (mL/m2) | 35.9±9.4 | 42.6±4.1†‡ | 32.0±3.6† | −10.6±2.3† | 30.3±8.9‡ | 29.0±7.1 | −1.3±4.1 | |
LVEF (%) | 53.9±4.6 | 50.0±3.2†‡ | 52.9±2.5† | 2.9±1.4† | 57.3±2.7 | 57.1±1.8 | −0.2±1.3 | |
IVSD (mm) | 9.2±1.1 | 8.9±1.0 | 8.8±0.9 | −0.1±1.5 | 9.4±1.2 | 9.2±0.9 | −0.2±1.5 | |
LVPWD (mm) | 8.8±0.8 | 8.6±0.8 | 8.8±0.8 | 0.1±1.2 | 9.0±0.9 | 8.9±0.8 | 0.1±1.9 | |
LVMI (g/m2) | 106.6±11.4 | 112.0±13.9†‡ | 95.2±9.7 | −16.7±7.5† | 102.1±6.0‡ | 98.1±7.6 | −4±4.3 | |
Septal e’ (cm/s) | 4.5±1.0 | 4.3±1.1 | 4.2±1.3 | −0.07±1.8 | 4.6±0.9 | 4.3±1.2 | −0.3±1.7 | |
E/e’ | 14.0±4.5 | 16.3±5.5†‡ | 15.5±3.6† | −0.8±2.5 | 12.2±2.3 | 12.1±2.0 | −0.1±1.3 |
†, significantly different (P<0.05) compared with the nonreverse LV remodeling group; ‡, significantly different (P<0.05) compared with the reverse LV remodeling group or nonreverse LV remodeling group 3 months after PCI. ∆t, the difference between 3 months after PCI and 1 week before PCI; E/e’, the ratio of early diastolic velocity of mitral valve (E) to the movement speed of mitral annulus at interventricular septum (e’). PCI, percutaneous coronary intervention; LV, left ventricular; LVEDVI, left ventricular end diastolic volume index; LVESVI, left ventricular end systolic volume index; LVEF, left ventricular ejection fraction; IVSD, interventricular septal thickness in diastole; LVPWD, left ventricular posterior wall thickness in diastole; LVMI, left ventricular mass index.
Myocardial work indices in patients with and without reverse LV remodeling
A significantly lower GLS absolute value, GWI, GCW, and GWE and higher GWW were observed in the reverse LV remodeling group compared with the nonreverse LV remodeling group (P<0.05). The GLS, GWI, GCW, GWW, and GWE in both the reverse LV remodeling group and the nonreverse LV remodeling group 3 months after PCI were significantly higher than the values obtained 1 week before PCI (P<0.05; Table 3).
Table 3
Variable | Reverse LV remodeling group (n=40) | Nonreverse LV remodeling group (n=48) | |||
---|---|---|---|---|---|
1 week before PCI | 3 months after PCI | 1 week before PCI | 3 months after PCI | ||
GLS (%) | 10.8±2.3†‡ | 13.5±5.4† | 14.9±2.3†‡ | 15.7±2.2 | |
GWI (mmHg%) | 1,246.9±231.2†‡ | 1,624.3±192.2† | 1,691.3±266.2†‡ | 1,864.8±179.4 | |
GCW (mmHg%) | 1,334.1±243.7†‡ | 1,766.9±168.5† | 1,774.6±287.6†‡ | 1,969.3±146.4 | |
GWW (mmHg%) | 190.3±101.9†‡ | 262.2±105.2† | 156.8±46.0†‡ | 180.9±48.4 | |
GWE (%) | 85.0±6.1†‡ | 91.8±3.9† | 90.8±2.4†‡ | 93.3±1.5 |
†, significantly different (P<0.05) compared with the nonreverse LV remodeling group; ‡, significantly different (P<0.05) compared with the reverse LV remodeling group or nonreverse LV remodeling group 3 months after PCI. GLS, global longitudinal strain; PCI, percutaneous coronary intervention; LV, left ventricular; GWI, global work index; GCW, global constructive work; GWW, global wasted work; GWE, global work efficiency.
Prediction of early LV reverse remodeling based on clinical and echocardiographic parameters
Logistic regression analysis was performed to identify independent predictors of early LV reverse remodeling. In the univariate analysis, TG, high density lipoprotein cholesterol (HDL), GLS, LVEF, LVMI, GCW, and GWE were significantly associated with early LV reverse remodeling. In multivariate analysis, LVMI, GCW, and GWE were independently associated with early LV reverse remodeling (Table 4).
Table 4
Variable | Univariate | Multivariate | |||
---|---|---|---|---|---|
OR (95% CI) | P value | OR (95% CI) | P value | ||
Age (years) | 0.984 (0.947–1.023) | 0.417 | – | – | |
Heart rate (bpm) | 1.005 (0.967–1.159) | 0.849 | – | – | |
Systolic pressure (mmHg) | 1.001 (0.950–1.056) | 0.959 | – | – | |
Diastolic pressure (mmHg) | 0.999 (0.928–1.076) | 0.985 | – | – | |
TG (mmol/L) | 0.297 (0.125–0.701) | 0.006 | – | – | |
HDL (mmol/L) | 0.033 (0.003–0.357) | 0.005 | – | – | |
LAD | 1.250 (0.348–4.486) | 0.732 | – | – | |
LCx | 1.333 (0.421–4.222) | 0.625 | – | – | |
RCA | 1.100 (0.323–3.746) | 0.879 | – | – | |
GLS | 0.571 (0.400–0.669) | <0.001 | – | – | |
LVEF | 0.364 (0.223–0.596) | <0.001 | – | – | |
LVMI (g/m2) | 1.109 (1.057–1.164) | <0.001 | 1.109 (1.042–1.181) | 0.001 | |
GCW (mmHg%) | 0.994 (0.992–0.997) | <0.001 | 0.995 (0.992–0.999) | 0.005 | |
GWW (mmHg%) | 1.008 (1.000–1.016) | 0.065 | – | – | |
GWE (%) | 0.603 (0.477–0.762) | <0.001 | 0.731 (0.538–0.995) | 0.046 |
LV, left ventricular; CAD, coronary artery disease; OR, odds ratio; TG, serum triglyceride; HDL, high density lipoprotein cholesterol; LAD, left anterior descending coronary artery; LCx, left circumflex artery; RCA, right coronary artery; GLS, global longitudinal strain; LVEF, left ventricular ejection fraction; LVMI, left ventricular mass index; GCW, global constructive work; GWW, global wasted work; GWE, global work efficiency.
According to ROC analysis, the area under the curve (AUC) of GCW was larger than that of GWE for predicting early LV reverse remodeling. According to the Youden index, a cutoff value of 1,438.5 mmHg% for GCW showed the highest accuracy in predicting early LV reverse remodeling, with a sensitivity of 85% and specificity of 70% (AUC =0.867; 95% CI: 0.793–0.942; P<0.001; Figure 3).

Repeatability of noninvasive myocardial work
Bland-Altman analysis showed good repeatability in terms of GWI, GCW, GWW, and GWE (Table 5). The intraobserver variability for GWI, GCW, GWW, and GWE had mean biases of 5.3, 4.9, 6.2, and −0.2 mmHg%, respectively, and high agreement (Figure 4A-4D). The interobserver variability for GWI, GCW, GWW, and GWE revealed mean biases of 14.8, 1.75, 7.3, and 0.6 mmHg%, respectively, and high agreement (Figure 5A-5D).
Table 5
Variable | Intraobserver variability | Interobserver variability | |||||
---|---|---|---|---|---|---|---|
d | ICC | 95% CI | d | ICC | 95% CI | ||
GWI (mmHg%) | 5.3±96.0 | 0.954 | −39.6 to 50.3 | 14.8±110.7 | 0.942 | −36.9 to 66.6 | |
GCW (mmHg%) | 4.9±96.6 | 0.963 | −40.4 to 50.1 | 1.75±113.9 | 0.945 | −51.5 to 55.1 | |
GWW (mmHg%) | 6.2±50.5 | 0.891 | −17.4 to 29.8 | 7.3±56.3 | 0.886 | −19.0 to 33.7 | |
GWE (%) | −0.2±2.8 | 0.912 | −1.5 to 1.1 | −0.6±5.0 | 0.890 | −1.7 to 3.0 |
d, difference; ICC, intraclass correlation coefficient; GWI, global work index; GCW, global constructive work; GWW, global wasted work; MW, myocardial work; GWE, global work efficiency.
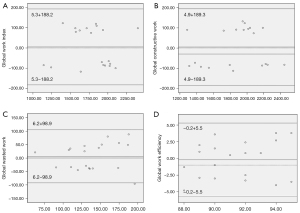
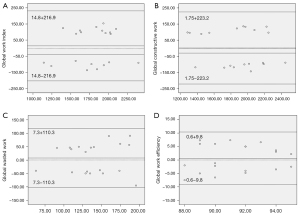
Discussion
In the present study, we demonstrated that patients with multivessel CAD who developed early LV reverse remodeling (reverse modeling group) had significantly lower preinterventional GWI, GCW, and GWE than did patients in the nonreverse LV remodeling group. In addition, LVMI, GCW, and GWE were independently associated with early LV reverse remodeling. Furthermore, GCW was found to be an independent predictor of early LV reverse remodeling.
Reverse LV remodeling and PCI
CAD involves long-term coronary stenosis or occlusion. This occlusion in turn leads to the ischemia and anoxia of cardiac myocytes and cell metabolism dysfunction; subsequently, increases are observed in LV inner diameter, cardiac mass, and ventricular remodeling, whereas decreases are observed in cardiac systolic and diastolic functions. The ultimate result is heart failure and greater rates of complications and mortality (16,17). In the current study, higher LVEDVI, LVESVI, LVMI, and E/e’ and lower LVEF were observed in the reverse LV remodeling group, thus indicating substantial LV reconstruction before PCI. Lower GWI, GCW, and GWE were observed in patients who developed early LV reverse remodeling before PCI. During myocardial ischemia, the heart decreases ATP synthesis and myocardial work because of changes in metabolism (18). Using positron emission tomography, Russell et al. (9) demonstrated there to be a strong correlation between the myocardial work index and regional myocardial glucose metabolism. Through timely and effective PCI treatment, the function of impaired but still alive myocardia can be restored, and the LV remodeling can be reversed (19,20).
Traditional echocardiographic parameters to evaluate early LV reverse remodeling
Conventional parameters used to evaluate LV reverse remodeling have been reported to decrease LVEDV by more than 15% (13,21) or LVESV by more than 10% (22,23) in patients with CAD 3 months after the baseline LVEDV or LVESV. Daubert et al. (24) defined improved LV systolic function by an increase in LVEF ≥5% and reported that reverse LV remodeling can occur after PCI in patients with complex CAD and reduced LVEF, with this being associated with improved clinical outcomes.
Recently, Pastore et al. (3) indicated that GLS is strongly correlated with outcomes in patients with CAD, including recurrence of acute myocardial infarction, LV remodeling, and death or hospitalization for heart failure. However, GLS is afterload dependent (25), and NIMWI combining GLS and afterload may overcome the limitations of GLS (26,27).
NIMWI and early LV reverse remodeling
NIMWI is noninvasive, accurate, fast, and convenient, and can account for the influence of the afterload on myocardial function (28,29). In the study by Lustosa et al. (30), patients with LV remodeling exhibited a greater degree of impaired myocardial work indices than did those patients without LV remodeling at 3-month follow-up after ST-segment elevation myocardial infarction. Another study performed by the above group (31) used NIMWI to evaluate LV myocardial work and LV remodeling in patients with ST-segment elevation myocardial infarction 3 months after PCI, demonstrating the regional cardiac work index in the culprit vessel territory before discharge to be independently associated with early adverse LV remodeling. However, few studies have been performed on reverse LV remodeling, and the association between NIMWI and reverse LV remodeling in multivessel CAD has not been evaluated.
In the present study, GCW and GWE were independently associated with early LV reverse remodeling in patients with multivessel CAD, but GCW was superior to GWE in predicting LV reverse remodeling. The cutoff value of 1,438.5 mmHg% for GCW showed the highest accuracy, with a sensitivity of 85% and a specificity of 70%. GCW is constructive work generated from the shortening of the myocytes during systole and their lengthening during isovolumic relaxation. GCW may be better at detecting viable myocardial segments because it consists only of positive work, which contributes more to LV systolic and diastolic function. Meimoun et al. (32) found GCW to be an independent predictor of LV remodeling in patients with acute ST-elevation myocardial infarction treated with PCI. Therefore, GCW can help identify early systolic dysfunction with LV reverse remodeling in patients with multivessel CAD after PCI; in addition, it may provide a reference for the timing of intervention.
Limitations
This study has several limitations which should be mentioned. (I) Diminished image quality caused by patient breathing, body shape, heart movement, and other factors might have affected the identification of LV endocardium and epicardium, as well as the strain measurements. NIMWI accuracy and the consistency of results within and between examiners might also have been affected. (II) The sample size was relatively small and impacted the regression results, and the follow-up time was short. (III) This study did not consider the effects of acute coronary syndrome, chronic coronary syndrome, different coronary lesion sites, coronary artery stenosis, or coronary artery occlusion on left ventricular myocardial work. (IV) The LVEF of patients with CAD was not further divided into subgroups. Finally, (V) intervendor differences in strain calculation might potentially limit the clinical applicability of our findings.
Conclusions
Among NIMWIs, GCW, a noninvasive and repeatable examination method, may be the major predictor of LV reverse remodeling in patients with multivessel CAD after PCI.
Acknowledgments
We are grateful to Songyan Liu for offering technical support.
Funding: This research was supported by the National Natural Science Foundation of China (Nos. 82071950, 61802330, and 81800287), the Medical Science and Technology Project of Henan Province (Nos. SB201901099, LHGJ20200084, and LHGJ20190805), and Natural Science Foundation of Henan Province for Excellent Young Scientists (No. 202300410364).
Footnote
Reporting Checklist: The authors have completed the STROBE reporting checklist. Available at https://qims.amegroups.com/article/view/10.21037/qims-21-1066/rc
Conflicts of Interest: All authors have completed the ICMJE uniform disclosure form (available at https://qims.amegroups.com/article/view/10.21037/qims-21-1066/coif). The authors have no conflicts of interest to declare.
Ethical Statement: The authors are accountable for all aspects of the work in ensuring that questions related to the accuracy or integrity of any part of the work are appropriately investigated and resolved. The study was conducted in accordance with the Declaration of Helsinki (as revised in 2013). The prospective study was approved by the ethics committee of the Henan Provincial People’s Hospital (Zhengzhou, China; approval No. 202031), and informed consent was obtained from all patients.
Open Access Statement: This is an Open Access article distributed in accordance with the Creative Commons Attribution-NonCommercial-NoDerivs 4.0 International License (CC BY-NC-ND 4.0), which permits the non-commercial replication and distribution of the article with the strict proviso that no changes or edits are made and the original work is properly cited (including links to both the formal publication through the relevant DOI and the license). See: https://creativecommons.org/licenses/by-nc-nd/4.0/.
References
- Ge J, Li J, Yu H, Hou B. Hypertension Is an Independent Predictor of Multivessel Coronary Artery Disease in Young Adults with Acute Coronary Syndrome. Int J Hypertens 2018;2018:7623639. [Crossref] [PubMed]
- Cohn JN, Ferrari R, Sharpe N. Cardiac remodel—g--concepts and clinical implications: a consensus paper from an international forum on cardiac remodeling. Behalf of an International Forum on Cardiac Remodeling. J Am Coll Cardiol 2000;35:569-82. [Crossref] [PubMed]
- Pastore MC, De Carli G, Mandoli G. E’ D'Ascenzi F, Focardi M, Contorni F, Mondillo S, Cameli M. The prognostic role of speckle tracking echocardiography in clinical practice: evidence and reference values from the literature. Heart Fail Rev 2021;26:1371-81. [Crossref] [PubMed]
- Ma H, Dai X, Yang X, Zhao X, Wang R, Zhang J. Clinical and imaging predictors of impaired myocardial perfusion in symptomatic patients after percutaneous coronary intervention: insights from dynamic CT myocardial perfusion imaging. Quant Imaging Med Surg 2021;11:3327-37. [Crossref] [PubMed]
- Lacalzada J, de la Rosa A, Izquierdo MM, Jiménez JJ, Iribarren JL, García-González MJ, López BM, Duque MA, Barragán A, Hernández C, Carrillo-Pérez M, Laynez I. Left ventricular global longitudinal systolic strain predicts adverse remodeling and subsequent cardiac events in patients with acute myocardial infarction treated with primary percutaneous coronary intervention. Int J Cardiovasc Imaging 2015;31:575-84. [Crossref] [PubMed]
- Cikes M, Solomon SD. Beyond ejection fraction: an integrative approach for assessment of cardiac structure and function in heart failure. Eur Heart J 2016;37:1642-50. [Crossref] [PubMed]
- Hubert A, Le Rolle V, Leclercq C, Galli E, Samset E, Casset C, Mabo P, Hernandez A, Donal E. Estimation of myocardial work from pressure-strain loops analysis: an experimental evaluation. Eur Heart J Cardiovasc Imaging 2018;19:1372-9. [Crossref] [PubMed]
- Chan J, Shiino K, Obonyo NG, Hanna J, Chamberlain R, Small A, Scalia IG, Scalia W, Yamada A, Hamilton-Craig CR, Scalia GM, Zamorano JL. Left Ventricular Global Strain Analysis by Two-Dimensional Speckle-Tracking Echocardiography: The Learning Curve. J Am Soc Echocardiogr 2017;30:1081-90. [Crossref] [PubMed]
- Russell K, Eriksen M, Aaberge L, Wilhelmsen N, Skulstad H, Remme EW, Haugaa KH, Opdahl A, Fjeld JG, Gjesdal O, Edvardsen T, Smiseth OA. A novel clinical method for quantification of regional left ventricular pressure-strain loop area: a non-invasive index of myocardial work. Eur Heart J 2012;33:724-33. [Crossref] [PubMed]
- Knuuti J, Wijns W, Saraste A, Capodanno D, Barbato E, Funck-Brentano C, et al. 2019 ESC Guidelines for the diagnosis and management of chronic coronary syndromes. Eur Heart J 2020;41:407-77. [Crossref] [PubMed]
- Shah R, Yow E, Jones WS, Kohl LP 3rd, Kosinski AS, Hoffmann U, Lee KL, Fordyce CB, Mark DB, Lowe A, Douglas PS, Patel MR. Comparison of visual assessment of coronary stenosis with independent quantitative coronary angiography: Findings from the Prospective Multicenter Imaging Study for Evaluation of Chest Pain (PROMISE) trial. Am Heart J 2017;184:1-9. [Crossref] [PubMed]
- Windecker S, Kolh P, Alfonso F, Collet JP, Cremer J, et al. 2014 ESC/EACTS Guidelines on myocardial revascularization: The Task Force on Myocardial Revascularization of the European Society of Cardiology (ESC) and the European Association for Cardio-Thoracic Surgery (EACTS)Developed with the special contribution of the European Association of Percutaneous Cardiovascular Interventions (EAPCI). Eur Heart J 2014;35:2541-619. [Crossref] [PubMed]
- Porciani MC, Lilli A, Macioce R, Cappelli F, Demarchi G, Pappone A, Ricciardi G, Padeletti L. Utility of a new left ventricular asynchrony index as a predictor of reverse remodelling after cardiac resynchronization therapy. Eur Heart J 2006;27:1818-23. [Crossref] [PubMed]
- Lang RM, Badano LP, Mor-Avi V, Afilalo J, Armstrong A, Ernande L, Flachskampf FA, Foster E, Goldstein SA, Kuznetsova T, Lancellotti P, Muraru D, Picard MH, Rietzschel ER, Rudski L, Spencer KT, Tsang W, Voigt JU. Recommendations for cardiac chamber quantification by echocardiography in adults: an update from the American Society of Echocardiography and the European Association of Cardiovascular Imaging. J Am Soc Echocardiogr 2015;28:1-39.e14. [Crossref] [PubMed]
- Milan A, Puglisi E, Magnino C, Naso D, Abram S, Avenatti E, Rabbia F, Mulatero P, Veglio F. Left atrial enlargement in essential hypertension: role in the assessment of subclinical hypertensive heart disease. Blood Press 2012;21:88-96. [Crossref] [PubMed]
- Stevens SM, Reinier K, Chugh SS. Increased left ventricular mass as a predictor of sudden cardiac death: is it time to put it to the test? Circ Arrhythm Electrophysiol 2013;6:212-7. [Crossref] [PubMed]
- Awadalla H, Saleh MA, Abdel Kader M, Mansour A. Left ventricular torsion assessed by two-dimensional echocardiography speckle tracking as a predictor of left ventricular remodeling and short-term outcome following primary percutaneous coronary intervention for acute myocardial infarction: A single-center experience. Echocardiography 2017;34:1159-69. [Crossref] [PubMed]
- Buja LM. Myocardial ischemia and reperfusion injury. Cardiovasc Pathol 2005;14:170-5. [Crossref] [PubMed]
- Ryan MJ, Perera D. Identifying and Managing Hibernating Myocardium: W’at's New and What Remains Unknown? Curr Heart Fail Rep 2018;15:214-23. [Crossref] [PubMed]
- Jiang Z, Tang H, Shi J, Zhou Y, Wang C, Li D, Shan Q, Zhou W. Myocardial stunning-induced left ventricular dyssynchrony on gated single-photon emission computed tomography myocardial perfusion imaging. Nucl Med Commun 2018;39:725-31. [Crossref] [PubMed]
- Yu CM, Gorcsan J 3rd, Bleeker GB, Zhang Q, Schalij MJ, Suffoletto MS, Fung JW, Schwartzman D, Chan YS, Tanabe M, Bax JJ. Usefulness of tissue Doppler velocity and strain dyssynchrony for predicting left ventricular reverse remodeling response after cardiac resynchronization therapy. Am J Cardiol 2007;100:1263-70. [Crossref] [PubMed]
- Spinelli L, Morisco C, Assante di Panzillo E, Izzo R, Trimarco B. Reverse left ventricular remodeling after acute myocardial infarction: the prognostic impact of left ventricular global torsion. Int J Cardiovasc Imaging 2013;29:787-95. [Crossref] [PubMed]
- Carrabba N, Parodi G, Valenti R, Migliorini A, Bellandi B, Antoniucci D. Prognostic value of reverse left ventricular remodeling after primary angioplasty for STEMI. Atherosclerosis 2012;222:123-8. [Crossref] [PubMed]
- Daubert MA, Massaro J, Liao L, Pershad A, Mulukutla S, Magnus Ohman E, Popma J. ’ O'Neill WW, Douglas PS. High-risk percutaneous coronary intervention is associated with reverse left ventricular remodeling and improved outcomes in patients with coronary artery disease and reduced ejection fraction. Am Heart J 2015;170:550-8. [Crossref] [PubMed]
- Boe E, Russell K, Eek C, Eriksen M, Remme EW, Smiseth OA, Skulstad H. Non-invasive myocardial work index identifies acute coronary occlusion in patients with non-ST-segment elevation-acute coronary syndrome. Eur Heart J Cardiovasc Imaging 2015;16:1247-55. [Crossref] [PubMed]
- Edwards NFA, Scalia GM, Shiino K, Sabapathy S, Anderson B, Chamberlain R, Khandheria BK, Chan J. Global Myocardial Work Is Superior to Global Longitudinal Strain to Predict Significant Coronary Artery Disease in Patients With Normal Left Ventricular Function and Wall Motion. J Am Soc Echocardiogr 2019;32:947-57. [Crossref] [PubMed]
- Li Y, Zheng Q, Cui C, Liu Y, Hu Y, Huang D, Wang Y, Liu J, Liu L. Application value of myocardial work technology by non-invasive echocardiography in evaluating left ventricular function in patients with chronic heart failure. Quant Imaging Med Surg 2022;12:244-56. [Crossref] [PubMed]
- Cui C, Liu L, Li Y, Liu Y, Huang D, Hu Y, Zhang L. Left Ventricular Pressure-Strain Loop-Based Quantitative Examination of the Global and Regional Myocardial Work of Patients with Dilated Cardiomyopathy. Ultrasound Med Biol 2020;46:2834-45. [Crossref] [PubMed]
- Manganaro R, Marchetta S, Dulgheru R, Ilardi F, Sugimoto T, Robinet S, et al. Echocardiographic reference ranges for normal non-invasive myocardial work indices: results from the EACVI NORRE study. Eur Heart J Cardiovasc Imaging 2019;20:582-90. [Crossref] [PubMed]
- Lustosa RP, van der Bijl P, El Mahdiui M, Montero-Cabezas JM, Kostyukevich MV, Ajmone Marsan N, Bax JJ, Delgado V. Noninvasive Myocardial Work Indices 3 Months after ST-Segment Elevation Myocardial Infarction: Prevalence and Characteristics of Patients with Postinfarction Cardiac Remodeling. J Am Soc Echocardiogr 2020;33:1172-9. [Crossref] [PubMed]
- Lustosa RP, Fortuni F, van der Bijl P, Goedemans L, El Mahdiui M, Montero-Cabezas JM, Kostyukevich MV, Ajmone Marsan N, Bax JJ, Delgado V, Knuuti J. Left ventricular myocardial work in the culprit vessel territory and impact on left ventricular remodelling in patients with ST-segment elevation myocardial infarction after primary percutaneous coronary intervention. Eur Heart J Cardiovasc Imaging 2021;22:339-47. [Crossref] [PubMed]
- Meimoun P, Abdani S, Stracchi V, Elmkies F, Boulanger J, Botoro T, Zemir H, Clerc J. Usefulness of Noninvasive Myocardial Work to Predict Left Ventricular Recovery and Acute Complications after Acute Anterior Myocardial Infarction Treated by Percutaneous Coronary Intervention. J Am Soc Echocardiogr 2020;33:1180-90. [Crossref] [PubMed]