Preliminary study on the assessment of early cartilage degeneration by quantitative ultrashort echo time magnetic resonance imaging in vivo
Introduction
Osteoarthritis (OA) is a common degenerative joint disease, characterized by degeneration of articular cartilage. Currently, there are no effective drugs to arrest the progression of OA, and total knee arthroplasty is recommended when OA has progressed to an advanced stage (1-3). Therefore, a noninvasive diagnosis of OA at an early stage is clinically important for implementing early intervention and alleviating symptoms. With an echo time (TE) of approximately 10–80 ms, the sensitivity of conventional magnetic resonance imaging (MRI) for detecting early cartilage degeneration is poor, partly due to the inability of MRI to obtain a signal from many short T2 tissues or tissue components in the knee joint, such as deep layer cartilage, subchondral bone, menisci, ligaments, and tendons (4). Ultrashort echo time (UTE) MRI techniques with TEs in the order of 10 ms allow for the direct imaging and quantitative assessment of the short T2 components in tissues (4-6).
In addition, conventional T2 and T1ρ scans are subject to the magic angle effect, and so the values are significantly affected when the orientation of tissue fiber approaches 54.7° relative to the main magnetic field (7). Shao et al. claimed that T2 values increased by 231.8% and T1ρ values increased by 92% on average near the magic angle, and the changes due to the magic angle effect can be several times larger than those caused by cartilage degeneration (8), which may affect the accuracy of quantitative results. Ma et al. (9) showed that the ultrashort echo time-based magnetization transfer (UTE-MT) technique was resistant to the magic angle effect in a two-pool model by scanning 5 cadaveric Achilles tendon samples in which orientations ranged from 0 to 90° relative to the main static (B0) magnetic field. Wu et al. (10) investigated the greatly decreased magic angle effect of three-dimensional (3D) UTE-adiabatic T1ρ sequences compared to regular 3D UTE Cones-CW-T1ρ and Cones-T2* sequences by repeating human patellar samples from different angular orientations. The feature of UTE-adiabatic T1ρ and UTE-MT showing less sensitivity to the magic angle effect than other conventional sequences is important for the quantitative evaluation of cartilage degeneration. Previous studies have indicated that the UTE-T2* values of deep articular cartilage typically decrease with more severe cartilage degeneration, and the UTE-T2* values are susceptible to water content and collagen orientation in early OA (11-13). The T1ρ values may reflect changes to the extracellular matrix (ECM), such as proteoglycan (PG) loss. Many in vitro studies have shown that UTE-T1ρ enables the quantitative assessment of short T2 tissues in the musculoskeletal system, and UTE-T1ρ values increase with structural changes in short T2 tissues (14-18). The magnetization transfer ratio (MTR) provides a measure of the magnetization exchange rate of hydrogen protons bound to macromolecules such as collagen and free water (19). The UTE-MTR values have been used to evaluate the pools of protons indirectly by analyzing the interactions between the bound and pore water proton pools (20,21). However, the capability of these UTE-based quantitative techniques to evaluate cartilage degeneration at an early stage has not yet been systematically assessed in vivo.
The purpose of the study was to investigate the feasibility of quantitative UTE-MRI sequences (UTE-MT, UTE-adiabatic T1ρ, and UTE-T2* mapping) for the assessment of early cartilage degeneration in vivo and to evaluate the diagnostic performance of these different UTE techniques. We present the following article in accordance with the STARD reporting checklist (available at https://qims.amegroups.com/article/view/10.21037/qims-21-1181/rc).
Methods
Participants
A total of 46 OA patients, including 26 males (age range: 30 to 71 years; 48.3±11.1 years) and 20 females (age range: 37 to 72 years; 51.0±7.9 years) with complaints of knee joint discomfort and no contraindication to MRI examination were recruited within a year. Among them, 5 patients were scanned bilaterally. The exclusion criteria included previous knee surgery or traumatic knee injury, tumor, or infectious lesion. The study was conducted in accordance with the Declaration of Helsinki (as revised in 2013). The study was approved by the Ethics Committee at the Shanghai Tenth People’s Hospital of Tongji University (approval number: shsy-iec-ky-3964). All participants provided written informed consent before the examination.
MRI protocol
The whole knee joint was scanned using a transmit/receive 8-channel knee coil (Chenguang Medical Technologies, Shanghai, China) on a 3.0 T clinical magnetic resonance (MR) scanner (MR750; GE Healthcare, Milwaukee, WI, USA). The following 4 imaging protocols were continuously performed without any clinical interventions: (A) proton density (PD) fat-suppressed (FS): repetition time (TR) =3,000 ms, TE =32 ms, scan time =3.37 min; (B) 3D UTE-MT: off-resonance frequency =2 kHz, saturation power =750 degrees, TR/TE =100/0.032 ms, scan time =6.4 min; (C) 3D UTE-adiabatic T1ρ: spin-locking times =0, 24, 48, and 96 ms, scan times =16.53 min; (D) UTE-T2* mapping: TEs =0.032, 4.9, 9.8, and 14.7 ms, TR =30.9 ms, scan time =5.2 min. Other imaging parameters included: field of view (FOV) =16 cm2, acquisition matrix =256×256 pixels, slice thickness =3 mm, and slices =32. The total scan time was 31.5 min.
Post-processing and image analysis
Digital Imaging and Communications in Medicine (DICOM) images obtained from the MR protocol mentioned above were analyzed in MatLab (Mathworks Inc., Natick, MA, USA). The UTE-T2* and UTE-adiabatic T1ρ values were calculated in MatLab (Mathworks Inc., USA) using the Levenberg–Marquardt method for nonlinear least-squares curve fitting problems, while the UTE-MTR was obtained using the 2-pool effect MT model with saturation powers of 750° (MT on) and 0° (MT off).
Regions of interests (ROIs) were manually drawn on the mid-sagittal section of the image of UTE-adiabatic T1ρ (TSL =0 ms) and then copied to each of the other sequence images. We mapped 3 ROIs on the medial and lateral femoral condyles and their corresponding medial and lateral tibial plateaus, respectively, as shown in Figure 1. Each ROI was measured in MatLab by one of the authors with a special interest in musculoskeletal radiology who was blinded to the MRI Osteoarthritis Knee Score (MOAKS) gradings, and this process was repeated in the same region 3 times, with the average signal intensity used for statistical analysis. All ROIs were graded according to the MOAKS by 2 musculoskeletal radiologists (each with 6 and 10 years of work, respectively) based on the sagittal (Sag)-proton density-weighted imaging (PDWI)/fat saturation (FS) sequence, as shown in Figure 2: normal cartilage (MOAKS 0); mild degeneration (MOAKS 1) = <10% defect of regional cartilage surface area; moderate degeneration (MOAKS 2) =10–75% defect of regional cartilage surface area; and severe degeneration (MOAKS 3) = >75% defect of regional cartilage surface area. The ROIs for which 2 musculoskeletal radiologists held different opinions were excluded. Since the main purpose of this study was to investigate the feasibility of UTE techniques for the diagnosis of OA at an early stage in vivo, ROIs with severe degeneration (MOAKS 3) were excluded. A total of 561 ROIs were finally included in this study.
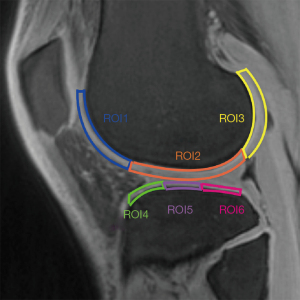
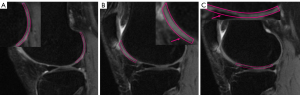
Statistical analysis
The software SPSS 20.0 (IBM Corp, Armonk, NY, USA) was used for the statistical analysis. Quantitative UTE-MRI values were normally distributed using the Kolmogorov–Smirnov normality test. The intra-class correlation coefficient (ICC) was used to describe the reliability of the measurements of UTE-MRI values. One-way analysis of variance (ANOVA) was used to compare the differences of different groups based on MOAKS scores, and the Tamhane-T2 test was performed for post hoc multiple comparisons when a significant difference existed. Spearman’s correlation analysis was used to test the correlation between different quantitative UTE-MRI values and MOAKS scores. Finally, receiver operating characteristic (ROC) curves were used to compare the diagnostic efficacy of different quantitative UTE-MRI techniques for mild cartilage degeneration (MOAKS 1). The area under the curve (AUC) values of different UTE-MRI values for mild cartilage degeneration (MOAKS 1) were calculated according to the ROC curves. A P value less than 0.05 was considered statistically significant.
Results
Comparison of quantitative UTE-MRI values among different groups of cartilage degeneration
The quantitative results of different UTE-biomarkers are shown in Table 1 and Figure 3. The UTE-MTR and UTE-T2* values in the normal group (MOAKS 0) were significantly higher than those in both the mild degeneration group (MOAKS 1) and moderate degeneration group (MOAKS 2) (P<0.001, P<0.001 and P=0.002, P<0.001, respectively). The UTE-adiabatic T1ρ values in the normal group (MOAKS 0) were significantly lower than those in the mild degeneration group (MOAKS 1) and moderate degeneration group (MOAKS 2) (P<0.001). Meanwhile, the UTE-MTR values in the mild degeneration group (MOAKS 1) were significantly higher than those in the moderate degeneration group (MOAKS 2) (P<0.001), and the UTE-adiabatic T1ρ values in the mild degeneration group (MOAKS 1) were significantly lower than those in the moderate degeneration group (MOAKS 2) (P<0.001). The UTE-adiabatic T1ρ [ICC =0.984, 95% confidence interval (CI): 0.937 to 0.996], UTE-MTR (ICC =0.977, 95% CI: 0.912 to 0.994), and UTE-T2* values (ICC =0.988, 95% CI: 0.952 to 0.997) all showed good reliability and reproducibility.
Table 1
Quantitative UTE-MRI sequences | MOAKS Grading | N | Mean ± SD | 95% CI | P value | Spearman correlation coefficient | |||
---|---|---|---|---|---|---|---|---|---|
vs. grade 1 | vs. grade 2 | r | P value | ||||||
T1ρ (ms) | 0 | 175 | 30.43±6.26 | 29.50–31.36 | <0.001 | <0.001 | 0.531 | <0.001 | |
1 | 283 | 35.05±8.78 | 34.03–36.07 | <0.001 | |||||
2 | 103 | 45.98±4.75 | 45.06–46.90 | ||||||
MTR (%) | 0 | 175 | 21.09±3.03 | 20.64–21.54 | <0.001 | <0.001 | −0.523 | <0.001 | |
1 | 283 | 17.30±3.22 | 16.23–18.37 | <0.001 | |||||
2 | 103 | 14.64±3.34 | 14.00–15.29 | ||||||
T2* (ms) | 0 | 175 | 21.49±3.96 | 20.76–22.23 | 0.002 | <0.001 | −0.396 | <0.001 | |
1 | 283 | 19.86±3.08 | 19.27–20.45 | <0.001 | |||||
2 | 103 | 15.15±2.84 | 14.60–15.70 |
SD, standard deviation; CI, confidence interval; UTE-MRI, ultrashort echo time magnetic resonance imaging; MOAKS, MRI Osteoarthritis Knee Score; MTR, magnetization transfer ratio; T2*, UTE-T2*mapping value.
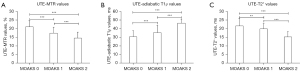
Correlation between different quantitative UTE-MRI values and MOAKS gradings
There was a moderate positive correlation between UTE-adiabatic T1ρ values and MOAKS gradings (r=0.531; P<0.001). The UTE-MTR values showed a moderate negative correlation with MOAKS gradings (r=−0.523; P<0.001), and UTE-T2* values showed a weak negative correlation with MOAKS gradings (r=−0.396; P<0.001).
Diagnostic efficacy of 3 quantitative UTE-MRI techniques using the ROC curve
As shown in Table 2 and Figure 4, the AUC values of UTE-MTR (AUC =0.794, 95% CI: 0.751 to 0.837; P<0.001) and UTE-adiabatic T1ρ (AUC =0.732, 95% CI: 0.685 to 0.779; P<0.001) were higher than that of UTE-T2* (AUC =0.651, 95% CI: 0.600 to 0.702; P<0.002). Of these, UTE-MTR exhibited the highest diagnostic sensitivity (0.754), and UTE-adiabatic T1ρ exhibited the highest diagnostic specificity (0.903).
Table 2
UTE-MRI sequences | AUC | 95% CI | P value | Sensitivity | Specificity |
---|---|---|---|---|---|
UTE-MT | 0.794 | 0.751–0.837 | 0.001 | 0.754 | 0.732 |
UTE-adiabaticT1ρ | 0.732 | 0.685–0.779 | 0.001 | 0.522 | 0.903 |
UTE-T2* mapping | 0.651 | 0.600–0.702 | 0.002 | 0.746 | 0.403 |
UTE-MRI, ultrashort echo time magnetic resonance imaging; MOAKS, MRI Osteoarthritis Knee Score; AUC, area under the curve; CI, confidence interval.
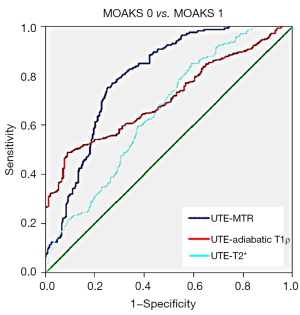
Discussion
This study systematically investigated the feasibility and potential of 3 different UTE-MRI techniques (UTE-MT, UTE-adiabatic T1ρ, and UTE-T2* mapping) for detecting early cartilage degeneration in vivo. It showed that UTE-MT and UTE-adiabatic T1ρ have a moderate correlation with MOAKS grading. Meanwhile, both techniques have higher diagnostic efficacy than UTE-T2* mapping, which indicates that UTE-MT and UTE-adiabatic T1ρ may have more potential for clinical applications than UTE-T2* mapping.
Williams et al. used UTE-T2* mapping to scan the tibial plateaus of human cadaveric specimens and suggested that UTE-T2* mapping has the sensitivity required to detect changes in the sub-surface matrix microstructure of articular cartilage (22,23). Wan et al. supported this by demonstrating the potential of 3D UTE-MT and UTE-adiabatic T1ρ to reflect matrix changes corresponding to early cartilage degeneration in an in vitro study (24). However, the variation of values may result from tissue degeneration after a freeze-thaw cycle, potentially impacting tissue MR properties and hydration as well as the temperature of samples during imaging (25). Besides, measurements taken in the controlled conditions of studies performed in vitro (e.g., extracellular pH, at room temperature) are not the same as the measurements taken in vivo in the real physiological environment, which may impact tissue MR properties (25,26). Williams et al. (27) demonstrated the feasibility and intersession repeatability of UTE-T2* mapping of articular cartilage in vivo, and Xue et al. (28) investigated the feasibility of 3D UTE-MT-derived macromolecular fraction (MMF) by scanning volunteers with and without OA. In the current study, the feasibility of 3 different quantitative UTE-MRI sequences for detecting early cartilage degeneration was investigated in vivo, thus promoting the clinical application of quantitative UTE-MRI techniques and facilitating the early detection and intervention of OA.
The onset of OA is mainly characterized by the loss of macromolecules, including PG and collagen, followed by the structural destruction of collagen fibers, reduction of bound water, and increase of free water (29-31). Therefore, the early detection of PG and collagen loss is important for identifying early cartilage degeneration and timely clinical intervention. Grey et al. showed reduced MTR values in trypsin-degraded bovine cartilage, suggesting that MTR values can reflect the reduction in the content of matrix macromolecules (e.g., collagen, PG) during cartilage degeneration (32). Fang et al. measured the UTE-MTR and UTE-T2* values of tendon in runners at different times and believed that UTE-MTR values can reflect the biochemical changes of the Achilles tendons (33). In this study, we found that the UTE-MTR values decreased with the increase of MOAKS gradings, which has a similar tendency to the results of our group’s previous in vitro study in which UTE-MTR values decreased with the degree of cartilage degeneration (34,35). The technology of UTE-MT is proving to be promising in a clinical setting, although there are still some further optimizations needed, such as the selection of frequency offsets and the MT powers.
Shen et al. found that T1ρ values increased with PG loss in the femoral condylar cartilage of OA rabbits (36). However, Menezes et al. found no direct correlation between changes of glycosaminoglycan (GAG) content and T1ρ values in mature human tissues at 8.5 T (37). The results of our study demonstrated that UTE-adiabatic T1ρ values of normal cartilage were significantly lower than those of the mildly and moderately degenerated cartilage groups, and there was a moderate positive correlation between UTE-adiabatic T1ρ values and MOAKS grading, which is consistent with the results of the study conducted by Shen et al. (36). This may relate to the magic angle-insensitivity of the UTE-adiabatic T1ρ sequence and its sensitivity in detecting short T2 tissues, allowing better observation of the deep cartilage and calcified layers that are first affected during early cartilage degeneration (10,16).
Previous in vitro studies have confirmed that UTE-T2* mapping is a potential method for evaluating the quality of cartilage, as it is sensitive to the destruction of deep cartilage (12,38,39). Williams et al. (22) claimed that UTE-T2* mapping has lower values in severely degraded cartilage compared to healthy cartilage. However, Chu et al. (40) found that the medial femoral condyle (MFC) deep cartilage MRI UTE-T2* value of anterior cruciate ligament (ACL)-injured patients was elevated compared to that of uninjured controls and UTE-T2* mapping can be used for the follow-up of clinical disease states. That may result from subchondral edema, an acute phase change after ACL injury, which leads to the increase of the long T2* (free water) components in cartilage (41). In this study, we found that UTE-T2* values of normal cartilage were significantly higher than those of the mild and moderate degenerated cartilage groups, which was consistent with our previous studies (34). However, UTE-T2* values have a weak negative correlation with MOAKS grading.
The diagnostic efficacy of UTE-MT and UTE-adiabatic T1ρ for detecting early cartilage degeneration is higher than that of UTE-T2* mapping. The variability in diagnostic efficacy of different UTE-MRI sequences may be due to the different biochemical basis of cartilage. The loss of PG and collagen content occurs before the changes of collagen fiber structure and water content (42). Loss of collagen and PG leads to a decrease of bound water, causing a decrease in T2* values, while collagen fiber destruction increases the exposed surface area of collagen, which may also lead to an increase of bound water (43). The simultaneous presence of both pathophysiological processes which cause contrary change of bound water certainly will affect the diagnostic efficacy of UTE-T2* values. In addition, UTE-T2* values are more susceptible to magic angle effects and magnetic field inhomogeneities than UTE-MT and UTE-adiabatic T1ρ, which can lead to an excessive increase in measured values (44).
There were several limitations to this study. Firstly, we did not perform scans on healthy volunteers comprising a control group. The normal quantitative UTE-MRI measured in the OA patients may not have been accurate. However, in the current study, both the trend of UTE-MRI values with increasing cartilage degeneration and the correlation between UTE-MRI values and cartilage degeneration were similar to previous studies (22,24,34), which means our results still have some degree of credibility. Secondly, the scan time was longer than the PDWI (3.37 min), especially for the 3D UTE-adiabatic T1ρ sequence (16.53 min), and this could be shortened by reducing the number of spin-lock times or advanced image reconstruction techniques, such as parallel imaging and compressed sensing (45). Thirdly, MOAKS scores instead of pathological histology were used as grouping criteria. The relationship between different quantitative UTE-MRI values and pathological histology will be further investigated in our future work, which might be beneficial for demonstrating the intrinsic mechanisms at play. Fourthly, although we enlarged the sample size by expanding the number of ROIs, the sample size was relatively small. Furthermore, the longest echo acquired in this experiment was only 14.7 ms, and long T2 components, such as free water, may not have been well-captured by this sequence; hence, the measured profile values may have been underestimated.
Conclusions
Quantitative UTE-MRI techniques (especially UTE-adiabatic T1ρ and UTE-MT) have the potential for detecting the degeneration of articular cartilage at an early stage in vivo. The diagnostic performance of UTE-based biomarkers, including UTE-adiabatic T1ρ, UTE-MT, and UTE-T2* mapping, for the assessment of mild degeneration of cartilage is good; thus, these UTE-based biomarkers may facilitate the noninvasive diagnosis of early OA in clinical practice.
Acknowledgments
Funding: This work was supported by grants from the National Natural Science Foundation of China (No. 81801656), Science and Technology Innovation Action Project of STCS (No. 20Y11911800), Scientific and Technology Innovation Plan of Chongming STCS (No. CKY2021-41), and Science and Technology Innovation Action Plan of STCS (No. 22YF1434000).
Footnote
Reporting Checklist: The authors have completed the STARD reporting checklist. Available at https://qims.amegroups.com/article/view/10.21037/qims-21-1181/rc
Conflicts of Interest: All authors have completed the ICMJE uniform disclosure form (available at https://qims.amegroups.com/article/view/10.21037/qims-21-1181/coif). The authors have no conflicts of interest to declare.
Ethical Statement: The authors are accountable for all aspects of the work in ensuring that questions related to the accuracy or integrity of any part of the work are appropriately investigated and resolved. The study was conducted in accordance with the Declaration of Helsinki (as revised in 2013). The study was approved by the Ethics Committee at the Shanghai Tenth People’s Hospital of Tongji University (approval number: shsy-iec-ky-3964), and written informed consent was provided by all participants.
Open Access Statement: This is an Open Access article distributed in accordance with the Creative Commons Attribution-NonCommercial-NoDerivs 4.0 International License (CC BY-NC-ND 4.0), which permits the non-commercial replication and distribution of the article with the strict proviso that no changes or edits are made and the original work is properly cited (including links to both the formal publication through the relevant DOI and the license). See: https://creativecommons.org/licenses/by-nc-nd/4.0/.
References
- Hawker GA. Osteoarthritis is a serious disease. Clin Exp Rheumatol 2019;37:3-6. [PubMed]
- Charlier E, Deroyer C, Ciregia F, Malaise O, Neuville S, Plener Z, Malaise M, de Seny D. Chondrocyte dedifferentiation and osteoarthritis (OA). Biochem Pharmacol 2019;165:49-65. [Crossref] [PubMed]
- Callahan LF, Ambrose KR, Albright AL, Altpeter M, Golightly YM, Huffman KF, Nelson AE, Weisner SE. Public Health Interventions for Osteoarthritis - updates on the Osteoarthritis Action Alliance's efforts to address the 2010 OA Public Health Agenda Recommendations. Clin Exp Rheumatol 2019;37:31-9. [PubMed]
- Siriwanarangsun P, Statum S, Biswas R, Bae WC, Chung CB. Ultrashort time to echo magnetic resonance techniques for the musculoskeletal system. Quant Imaging Med Surg 2016;6:731-43. [Crossref] [PubMed]
- Liu J, Wei Y, Ma YJ, Zhu YC, Zhou Q, Zhao YH. Magnetic resonance imaging of the zone of calcified cartilage in the knee joint using 3-dimensional ultrashort echo time cones sequences. Chin Med J (Engl) 2019;132:562-8. [Crossref] [PubMed]
- Geiger D, Bae WC, Statum S, Du J, Chung CB. Quantitative 3D ultrashort time-to-echo (UTE) MRI and micro-CT (μCT) evaluation of the temporomandibular joint (TMJ) condylar morphology. Skeletal Radiol 2014;43:19-25. [Crossref] [PubMed]
- Wang L, Regatte RR. Investigation of regional influence of magic-angle effect on t2 in human articular cartilage with osteoarthritis at 3 T. Acad Radiol 2015;22:87-92. [Crossref] [PubMed]
- Shao H, Pauli C, Li S, Ma Y, Tadros AS, Kavanaugh A, Chang EY, Tang G, Du J. Magic angle effect plays a major role in both T1rho and T2 relaxation in articular cartilage. Osteoarthritis Cartilage 2017;25:2022-30. [Crossref] [PubMed]
- Ma YJ, Shao H, Du J, Chang EY. Ultrashort echo time magnetization transfer (UTE-MT) imaging and modeling: magic angle independent biomarkers of tissue properties. NMR Biomed 2016;29:1546-52. [Crossref] [PubMed]
- Wu M, Ma YJ, Kasibhatla A, Chen M, Jang H, Jerban S, Chang EY, Du J. Convincing evidence for magic angle less-sensitive quantitative T1ρ imaging of articular cartilage using the 3D ultrashort echo time cones adiabatic T1ρ (3D UTE cones-AdiabT1ρ) sequence. Magn Reson Med 2020;84:2551-60. [Crossref] [PubMed]
- Pauli C, Bae WC, Lee M, Lotz M, Bydder GM, D'Lima DL, Chung CB, Du J. Ultrashort-echo time MR imaging of the patella with bicomponent analysis: correlation with histopathologic and polarized light microscopic findings. Radiology 2012;264:484-93. [Crossref] [PubMed]
- Tao H, Qiao Y, Hu Y, Xie Y, Lu R, Yan X, Chen S. Quantitative T2-Mapping and T2*-Mapping Evaluation of Changes in Cartilage Matrix after Acute Anterior Cruciate Ligament Rupture and the Correlation between the Results of Both Methods. Biomed Res Int 2018;2018:7985672. [Crossref] [PubMed]
- Foreman SC, Ashmeik W, Baal JD, Han M, Bahroos E, von Schacky CE, Carl M, Krug R, Joseph GB, Link TM. Patients with Type 2 Diabetes Exhibit a More Mineralized Deep Cartilage Layer Compared with Nondiabetic Controls: A Pilot Study. Cartilage 2021;13:428S-36S. [Crossref] [PubMed]
- Iwasaki K, Tafur M, Chang EY, Statum S, Biswas R, Tran B, Bae WC, Du J, Bydder GM, Chung CB. High-Resolution Qualitative and Quantitative Magnetic Resonance Evaluation of the Glenoid Labrum. J Comput Assist Tomogr 2015;39:936-44. [Crossref] [PubMed]
- Chang EY, Campos JC, Bae WC, Znamirowski R, Statum S, Du J, Chung CB. Ultrashort Echo Time T1ρ Is Sensitive to Enzymatic Degeneration of Human Menisci. J Comput Assist Tomogr 2015;39:637-42. [Crossref] [PubMed]
- Ma YJ, Carl M, Shao H, Tadros AS, Chang EY, Du J. Three-dimensional ultrashort echo time cones T1ρ (3D UTE-cones-T1ρ) imaging. NMR Biomed 2017; [Crossref] [PubMed]
- Ma YJ, Jerban S, Carl M, Wan L, Guo T, Jang H, Bydder GM, Chang EY, Du J. Imaging of the region of the osteochondral junction (OCJ) using a 3D adiabatic inversion recovery prepared ultrashort echo time cones (3D IR-UTE-cones) sequence at 3 T. NMR Biomed 2019;32:e4080. [Crossref] [PubMed]
- Casula V, Nissi MJ, Podlipská J, Haapea M, Koski JM, Saarakkala S, Guermazi A, Lammentausta E, Nieminen MT. Elevated adiabatic T1ρ and T2ρ in articular cartilage are associated with cartilage and bone lesions in early osteoarthritis: A preliminary study. J Magn Reson Imaging 2017;46:678-89. [Crossref] [PubMed]
- Shinn RL, Pancotto TE, Stadler KL, Werre SR, Rossmeisl JH. Magnetization transfer and diffusion tensor imaging in dogs with intervertebral disk herniation. J Vet Intern Med 2020;34:2536-44. [Crossref] [PubMed]
- Jerban S, Ma Y, Namiranian B, Ashir A, Shirazian H, Wei Z, Le N, Wu M, Cai Z, Du J, Chang EY. Age-related decrease in collagen proton fraction in tibial tendons estimated by magnetization transfer modeling of ultrashort echo time magnetic resonance imaging (UTE-MRI). Sci Rep 2019;9:17974. [Crossref] [PubMed]
- Chang EY, Bae WC, Shao H, Biswas R, Li S, Chen J, Patil S, Healey R, D'Lima DD, Chung CB, Du J. Ultrashort echo time magnetization transfer (UTE-MT) imaging of cortical bone. NMR Biomed 2015;28:873-80. [Crossref] [PubMed]
- Williams A, Qian Y, Bear D, Chu CR. Assessing degeneration of human articular cartilage with ultra-short echo time (UTE) T2* mapping. Osteoarthritis Cartilage 2010;18:539-46. [Crossref] [PubMed]
- Titchenal MR, Williams AA, Chehab EF, Asay JL, Dragoo JL, Gold GE, McAdams TR, Andriacchi TP, Chu CR. Cartilage Subsurface Changes to Magnetic Resonance Imaging UTE-T2* 2 Years After Anterior Cruciate Ligament Reconstruction Correlate With Walking Mechanics Associated With Knee Osteoarthritis. Am J Sports Med 2018;46:565-72. [Crossref] [PubMed]
- Wan L, Cheng X, Searleman AC, Ma YJ, Wong JH, Meyer RS, Du J, Tang G, Chang EY. Evaluation of enzymatic proteoglycan loss and collagen degradation in human articular cartilage using ultrashort echo time-based biomarkers: A feasibility study. NMR Biomed 2022;35:e4664. [Crossref] [PubMed]
- Wright P, Jellus V, McGonagle D, Robson M, Ridgeway J, Hodgson R. Comparison of two ultrashort echo time sequences for the quantification of T1 within phantom and human Achilles tendon at 3 T. Magn Reson Med 2012;68:1279-84. [Crossref] [PubMed]
- Filho GH, Du J, Pak BC, Statum S, Znamorowski R, Haghighi P, Bydder G, Chung CB. Quantitative characterization of the Achilles tendon in cadaveric specimens: T1 and T2* measurements using ultrashort-TE MRI at 3 T. AJR Am J Roentgenol 2009;192:W117-24. [Crossref] [PubMed]
- Williams A, Qian Y, Chu CR. UTE-T2* mapping of human articular cartilage in vivo: a repeatability assessment. Osteoarthritis Cartilage 2011;19:84-8. [Crossref] [PubMed]
- Xue YP, Ma YJ, Wu M, Jerban S, Wei Z, Chang EY, Du J. Quantitative 3D Ultrashort Echo Time Magnetization Transfer Imaging for Evaluation of Knee Cartilage Degeneration In Vivo. J Magn Reson Imaging 2021;54:1294-302. [Crossref] [PubMed]
- Guermazi A, Alizai H, Crema MD, Trattnig S, Regatte RR, Roemer FW. Compositional MRI techniques for evaluation of cartilage degeneration in osteoarthritis. Osteoarthritis Cartilage 2015;23:1639-53. [Crossref] [PubMed]
- Deng B, Wang F, Yin L, Chen C, Guo L, Chen H, Gong X, Li Y, Yang L. Quantitative study on morphology of calcified cartilage zone in OARSI 0~4 cartilage from osteoarthritic knees. Curr Res Transl Med 2016;64:149-54. [Crossref] [PubMed]
- Ryd L, Brittberg M, Eriksson K, Jurvelin JS, Lindahl A, Marlovits S, Möller P, Richardson JB, Steinwachs M, Zenobi-Wong M. Pre-Osteoarthritis: Definition and Diagnosis of an Elusive Clinical Entity. Cartilage 2015;6:156-65. [Crossref] [PubMed]
- Gray ML, Burstein D, Lesperance LM, Gehrke L. Magnetization transfer in cartilage and its constituent macromolecules. Magn Reson Med 1995;34:319-25. [Crossref] [PubMed]
- Fang Y, Zhu D, Wu W, Yu W, Li S, Ma YJ. Assessment of Achilles Tendon Changes After Long-Distance Running Using Ultrashort Echo Time Magnetization Transfer MR Imaging. J Magn Reson Imaging 2022; [Epub ahead of print]. [Crossref] [PubMed]
- Yang J, Shao H, Ma Y, Wan L, Zhang Y, Jiang J, Du J, Tang G. Quantitative ultrashort echo time magnetization transfer (UTE-MT) for diagnosis of early cartilage degeneration: comparison with UTE-T2* and T2 mapping. Quant Imaging Med Surg 2020;10:171-83. [Crossref] [PubMed]
- Shao H, Yang J, Ma Y, Su X, Tang G, Jiang J, Du J, Liu J. Evaluation of cartilage degeneration using multiparametric quantitative ultrashort echo time-based MRI: an ex vivo study. Quant Imaging Med Surg 2022;12:1738-49. [Crossref] [PubMed]
- Shen S, Wang H, Zhang J, Wang F, Chen M. T1ρ magnetic resonance imaging quantification of early articular cartilage degeneration in a rabbit model. BMC Musculoskelet Disord 2015;16:361. [Crossref] [PubMed]
- Menezes NM, Gray ML, Hartke JR, Burstein D. T2 and T1rho MRI in articular cartilage systems. Magn Reson Med 2004;51:503-9. [Crossref] [PubMed]
- Wong TT, Quarterman P, Lynch TS, Rasiej MJ, Jaramillo D, Jambawalikar SR. Feasibility of ultrashort echo time (UTE) T2* cartilage mapping in the hip: a pilot study. Acta Radiol 2021; [Epub ahead of print]. [PubMed]
- Williams AA, Erhart-Hledik JC, Asay JL, Mahtani GB, Titchenal MR, Lutz AM, Andriacchi TP, Chu CR. Patient-Reported Outcomes and Knee Mechanics Correlate With Patellofemoral Deep Cartilage UTE-T2* 2 Years After Anterior Cruciate Ligament Reconstruction. Am J Sports Med 2021;49:675-83. [Crossref] [PubMed]
- Chu CR, Williams AA, West RV, Qian Y, Fu FH, Do BH, Bruno S. Quantitative Magnetic Resonance Imaging UTE-T2* Mapping of Cartilage and Meniscus Healing After Anatomic Anterior Cruciate Ligament Reconstruction. Am J Sports Med 2014;42:1847-56. [Crossref] [PubMed]
- Speer KP, Spritzer CE, Bassett FH 3rd, Feagin JA Jr, Garrett WE Jr. Osseous injury associated with acute tears of the anterior cruciate ligament. Am J Sports Med 1992;20:382-9. [Crossref] [PubMed]
- Link TM, Li X. Establishing compositional MRI of cartilage as a biomarker for clinical practice. Osteoarthritis Cartilage 2018;26:1137-9. [Crossref] [PubMed]
- Shao H, Chang EY, Pauli C, Zanganeh S, Bae W, Chung CB, Tang G, Du J. UTE bi-component analysis of T2* relaxation in articular cartilage. Osteoarthritis Cartilage 2016;24:364-73. [Crossref] [PubMed]
- Eagle S, Potter HG, Koff MF. Morphologic and quantitative magnetic resonance imaging of knee articular cartilage for the assessment of post-traumatic osteoarthritis. J Orthop Res 2017;35:412-23. [Crossref] [PubMed]
- Otazo R, Kim D, Axel L, Sodickson DK. Combination of compressed sensing and parallel imaging for highly accelerated first-pass cardiac perfusion MRI. Magn Reson Med 2010;64:767-76. [Crossref] [PubMed]