The use of isodose levels to interpret radiation induced lung injury: a quantitative analysis of computed tomography changes
Introduction
Stereotactic body radiation therapy (SBRT), also known as stereotactic ablative radiotherapy (SABR), has been adopted as an effective method for treating lung cancer, including early stage lung cancer in inoperable patients (1) and patients with lung oligo metastases (2). The use of SBRT will likely increase over time, both due to the increasing cancer burden worldwide, as well as the efficacy, low toxicity profile, cost effectiveness, and ease of compliance with SBRT (3). High local control rates have been reported in numerous prospective and retrospective series. In a phase II trial of 59 patients, Timmerman et al. (4) demonstrated a 3-year primary tumor control rate, local control rate, and local-regional control rate of 97.6%, 90.6% and 87.2%, respectively. Other studies have found similar high local control rates for both primary lung cancers and lung oligo metastases (5-7).
The diagnosis of responsive, stable, or progressive disease is based on radiographic findings; therefore it is imperative that the radiologist be able to differentiate expected radiation-induced lung injury (RILI) progression from tumor re-occurrence. Computed tomography (CT) is the typical method for following patients. Alternatives include PET/CT and chest radiography (8). Ebright et al., have advocated that PET and diagnostic CT can enhance detection of recurrence (9). The tumor response to therapy is generally evaluated using the RECIST 1.1 guidelines (10,11). The guidelines state, however, that lesions in a previously irradiated area may not be considered “measurable” unless there is actual progression.
In addition to following the tumor response after therapy, it is widely known that normal lung tissue also exhibits changes (12). When followed with CT imaging after SBRT, >90% of patients are observed to have radiographic evidence of pulmonary injury in the irradiated lung (13). This occurs in normal lung tissue that was not specifically targeted for cancer therapy, and may have received radiation doses as little as 16 Gy (14). The distribution of lung changes after lung radiation (whether conventional radiation or SBRT) has been reported to vary between homogenous, patchy, or discrete consolidation; these areas may or may not appear to conform to the radiation portal shape (14,15). Other reports have indicated that lung injury conforms to and correlates with the high-dose regions (16,17).
Attempts at clarifying the predictability of radiation induced RILI is made difficult by the fact that the direction of radiation portals used in SBRT is more complicated than those used in conventional external beam radiation therapy (EBRT). It is often impossible to distinguish between the initially treated primary tumor and the surrounding RILI that develops (14,16). The RILI itself may appear as a mass-like shape which can resemble recurrent disease (13,16); however, the majority of these suspicious opacities are ultimately not biopsy-proven as recurrences (18). The RILI does not follow an anatomic pattern but rather is specifically related to the therapeutic details, and as such, detailed information regarding the treatment portals is necessary for accurate interpretation (12). CT changes can continue to progress even two years beyond treatment cessation (13); therefore, there are numerous opportunities during this interval for misinterpretation that may lead to unnecessary worry and biopsies.
Palma et al. (17) showed that lung density changes surrounding the tumor, as measured in Hounsfield Units, significantly correlated with radiographic evidence of pneumonitis. These density changes were most accurately measured using deformable registration, as rigid registration cannot account for lung volume and tumor position changes on follow up (FU) scans. This issue is compounded by the steep dose fall-off when treating with SBRT. They advocated for using deformable registration to compare new radiological findings on FU CT to previous dose distributions. The “peri-tumoral” region, within which CT changes may be found, has not been well defined. We aimed to define and quantify whether treatment isodose levels can reliably correlate with CT lung changes.
Methods and materials
We performed a retrospective review of all patients treated with lung SBRT in our department from 2010–2012, who underwent routine CT scan FU surveillance. Four-dimensional (4D) CT scans were acquired in our department for treatment planning use. A 4D CT scan is a 3-dimensional CT scan wherein extra individual scans are also taken throughout the respiratory cycle, so that the tumor can be targeted in all phases of respiration. FU diagnostic CT scans were performed at the facility of the patient’s choice. Multi-slice CT scanners were utilized and axial images were reviewed on lung window settings window 1,500 and level −500.
A radiologist (M.S.) and radiation oncologist (M.K.) collaboratively contoured the total amount of RILI surrounding the treated tumor using standard lung window levels for optimal viewing. RILI was defined based on radiographic loss of air content of normal lung tissue or patchy or dense infiltration in the area of lung treated with radiation. The total volume of radiation-induced RILI (RILI) was calculated as the volume of the contoured structure containing the area of RILI.
As most of the treated patients were former or current smokers, we reviewed the pre-treatment CTs were for evidence of emphysema. Each patient’s baseline smoking history was recorded. Patients were scored by number of pulmonary lobes with emphysema and severity of emphysema in each lobe (mild, moderate or severe). Mild emphysema was defined as: 0–25% of the lobe affected, moderate was defined as: 25–75% of the lobe affected, and severe was defined as: >75% of the lobe affected.
We performed image fusion between each patient’s pre- and post-treatment scans, to limit error based on different patient positioning between scans. Interval lung scans differed slightly based upon changes in the patient’s weight, anatomy, breathing pattern, or positioning for the scan including whether arms up or down. For example, during the simulation scan, patients’ arms are placed over their head so that their arms will receive less radiation during their planned treatment. During most of the FU scans, the patients’ arms were down by their sides. A two-step deformable image registration process was applied to fuse the studies. First the rigid body registration was applied to align two scans in treatment area and then a B-spline multi-pass deformable registration algorithm (19,20) was applied to fuse two image sets. This demon based B-spline deformable registration algorithm gave a better visual result compared to the intensity-based free-form deformable algorithm in the low density area. The intensity-based algorithm created significant swirls artifacts inside the lung due to the signal noise.
After the pre- and post-treatment image registration was performed, the treatment selected levels of isodose curves were overlaid onto the post-treatment scan (Figure 1). To describe and quantify the spatial orientation of the RILI in relation to each isodose line (isodose 5 Gy − isodose 40 Gy in 5 Gy increments), we measured both the total amount of RILI and the amount of RILI extending outside a given isodose curve. We defined a RILI extension index [fibrosis extension index (FEI)] to express the percentage of RILI extending outside a given isodose level relative to the total volume of RILI seen on follow-up CT (Figure 2). A FEI of 0% indicated that all of the RILI was included within a given isodose curve, while an FEI of 100% indicated that all of the observed RILI fell outside a given isodose curve. The FEI was plotted as a function of dose levels 5, 10, 15, 20, 25, 30, 35, and 40 Gy. We did not utilize a conformity index because our goal was to describe the peri-tumoral RILI zone surrounding the tumor, not its conformity to the shape of the treated tumor or isodose curves.
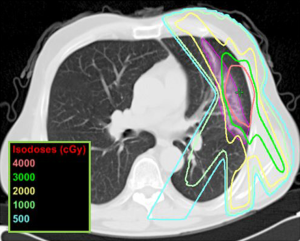
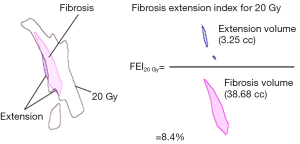
Statistical analysis was performed using Statistical Package of Social Sciences (SPSS version 15.0). Where appropriate, t test or Pearson’s correlation were used, with P=0.05 indicating statistical significance.
Results
We included 37 lesions in 32 patients who were treated between June 2010 and June 2012. These patients had undergone routine FU scans that were available for review. Of these patients, all thirty two underwent an initial FU scan, 26 patients underwent a second FU scan and six patients underwent a third FU scan. Patients were treated with varying fractionation schedules as clinically indicated, ranging from 4,500 cGy in 5 fractions to 5,400 cGy in 3 fractions (Table 1). The first, second, and third CT scans occurred at a median of 6 (range, 3–7), 10 (range, 8–13), and 16.5 (range, 14–19) months. The mean total RILI volume at 1st, 2nd, and 3rd FU CT’s was 69, 47, and 42 cc, respectively.
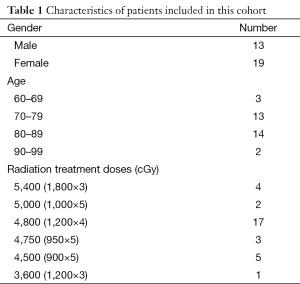
Full table
In Figure 1, a diagnostic CT scan was obtained 6 months after the completion of SBRT to a left lung lesion. The radiation-induced RILI was contoured and correlated with the various isodose levels that were overlaid onto the scan. Visual evaluation suggests that the 20 Gy isodose line has a high degree of correlation with the pattern of RILI.
Univariate analysis using Pearson’s correlation revealed that the planning target volume (PTV) was positively correlated with the volume of radiation induced RILI [correlation coefficient (CC) =0.628 and P<0.0001 at 1st FU; CC =0.401 and P=0.021 at 2nd FU; CC =0.265 and P=0.306 at 3rd FU]. PTV was also significantly negatively correlated with FEI, such that increasing PTV corresponded to a lower percentage of RILI extending outside the isodose curve, with the strongest correlation seen at the 40 Gy isodose level (CC =−0.361; P=0.016).
FEI 40 Gy at 1st FU was significantly positively correlated with FEI 40 Gy at subsequent FU’s (CC =0.689 and P=6.5×10−5 comparing 1st and 2nd FU; 0.901 and P=0.020 comparing 2nd and 3rd FUs. A similar trend was seen for FEI 20 Gy, FEI 30 Gy and FEI 35 Gy, where 1st FU positively correlated with 2nd FU and 2nd FU positively correlated with 3rd FU. Notably, 65% of patients were found to have a decrease in RILI at 2nd FU when compared to 1st FU. FEI was found to decrease with lower isodose levels, such that median FEI for isodose levels 40, 35, 25, 20, 15, 10 and 5 were 45%, 40%, 33%, 17%, 9%, 4%, 1%, and 0% respectively (Figure 3). FEI was not affected by fraction size (P=0.660).
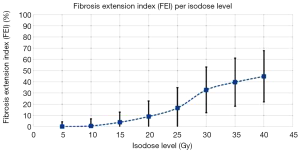
At the initial FU scan of 6 months, the median FEI at 20 Gy was 7.9% for primary lung cancers vs. 1.1% for lung metastases; however, only 6/32 of these patients had lung metastases. At the second FU scan of 10 months, the median FEI at 20 Gy was 6.8% for primary lung cancers vs. 5.2% for lung metastases; 4/23 of these patients had lung metastases.
One patient experienced grade III pneumonitis and required steroids with improvement. The FEI on her scans did not significantly differ from the remainder of the cohort. Another patient experienced grade I pneumonitis which was self-limited. All other patients were not observed to experience pneumonitis. In all our patients to date, we have observed one local recurrence (subsequently retreated with SBRT), one regional failure, two distant failures, and one concurrent regional/distant failure. The local failure was confirmed by biopsy.
The mean volume of RILI in patients with and without emphysema was 35.4 and 99.2 mm3, respectively. The presence and severity of emphysema was significantly inversely proportional to the total RILI (P=0.024 and P=0.003, respectively). Age was not significantly associated with RILI (P=0.441). The number of total lung lobes with emphysema was not significantly associated with RILI (P=0.276). Smoking status was also not significantly correlated with RILI, although only three patients were never-smokers (Figure 4).
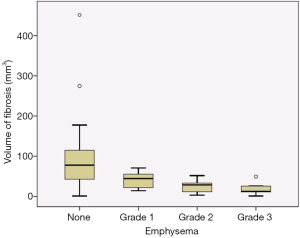
Discussion
This study describes the response of normal lung tissue to SBRT, as evident on CT imaging. As expected, the volume of irradiated lung receiving the PTV was significantly correlated with the radiation-induced RILI volume. We have found that a large portion of the total RILI occurs in a region receiving a moderate dose of radiation, i.e., not only within the tumor itself and not within the high dose area. This is described by the FEI measure, which gives a quantifiable percentage of the RILI that was found outside a given isodose level. The higher isodose levels (i.e., the area of the treated tumor) were found to have a high FEI, showing that much of the RILI extends outside the high dose region. In contrast, the lower isodose levels encompassed most, or all, of the RILI and therefore have a low FEI which limits their utility in interpreting subsequent CT scans. These findings are important because when clinicians and radiologists are analyzing FU scans, the only information readily available may be scans of the tumor itself prior to the radiation treatment. However, we have shown that the RILI frequently appears at a distance to the tumor, in a spatial orientation related to the specific treatment administered (i.e., as described by isodose levels). Therefore, treatment information is helpful for interpretation of CT FU scans.
Our results show that the 20-Gy isodose curve encompassed >90% of the RILI, and the 15-Gy isodose curve encompassed >95% of the RILI. This suggests that the 20 Gy isodose curve might be the most useful in determining the pattern of RILI that is not worrisome for recurrence. For example, in Figure 1, we can observe qualitatively that the 20 Gy isodose curve approximates the pattern and shape of the observed RILI. The 30 Gy isodose curve does not encompass the entire fibrotic region (i.e., it has a high FEI) and the 10 Gy isodose curve encompasses a large volume of unaffected lung. We also found that patients with radiographic evidence of emphysema had significantly decreased RILI on post-treatment CT, as compared patients without emphysema. Within the subset of patients with emphysema, those with increased severity had significant decreases in RILI.
In addition, FEI correlated over the course of the 1st, 2nd, and 3rd FU scans, demonstrating that the RILI was repeatedly seen within the low dose regions. The presence of a dose response in regional lung CT density changes following lung irradiation has been studied by Ma et al. (21), and minimal lung changes were found in areas receiving <20 Gy. Individual patient’s dose response curves were found to evolve over the course of FU. However, these changes were no longer significant after the 3 months FU period; scans from 6 months and after showed stable changes (21). In our study, the first FU occurred at a median of 6 months. Nearly two thirds of the patients had a decrease in total volume of RILI at the second FU period. At the same time, FEI was consistent between the FU intervals, supporting this measure as a useful tool for describing RILI. This is important because if a reliably ‘safe’ pattern of RILI can be established at a patient’s first FU CT, subsequent scans can be more readily interpreted.
Limitations to the study include the small number of patients evaluated. In addition, we utilized different fractionation schedules as clinically indicated, ranging from 4,500 cGy in 5 fractions to 5,400 cGy in 3 fractions. It has been demonstrated that decreasing the dose per fraction, especially for central tumors, does not alter local control despite differing biologically effective doses as computed using the linear quadratic formalism (22). As such, we felt it was reasonable to include these patients together for this analysis. There were also differences in patient positioning during pre- and post-treatment scans, which while unavoidable may not have been entirely corrected for by deformable registration. Other inaccuracies in FU imaging include respiratory motion, fibrotic retraction, and changes in patient anatomy over time. In addition, we used the pencil-beam algorithm to calculated dose and isodose curves, because this was our institution’s adopted method used for these patients’ actual treatment plans. However, other algorithms may have yielded slightly different results.
Currently there are no accepted criteria for predicting RILI (13,21,23). Our research has demonstrated that knowledge of isodose curves, and in particular the 20 Gy isodose curve, allows the Radiologist to better distinguish radiation induced lung injury from disease progression. Radiologist’s knowledge of individual patient’s isodose curves echoes the recommendations of Donaldson who writes, “the opportunities that cross-disciplinary partnerships afford include the excitement of developing innovative ideas with others, the challenges of building new research programs, and the satisfaction of creating new collegial relationships. These opportunities invigorate radiology and radiation oncology as well as each of us as individual subspecialist radiologists (24)”.
Conclusion
We advocate an interdisciplinary approach to interpreting post-SBRT CT and for sharing treatment planning information with diagnostic radiologists to improve the accuracy of CT reporting.
Acknowledgements
None.
Footnote
Conflicts of Interest: The authors have no conflicts of interest to declare.
References
- Lagerwaard FJ, Haasbeek CJ, Smit EF, Slotman BJ, Senan S. Outcomes of risk-adapted fractionated stereotactic radiotherapy for stage I non-small-cell lung cancer. Int J Radiat Oncol Biol Phys 2008;70:685-92. [PubMed]
- Siva S, MacManus M, Ball D. Stereotactic radiotherapy for pulmonary oligometastases: a systematic review. J Thorac Oncol 2010;5:1091-9. [PubMed]
- Nagata Y, Wulf J, Lax I, Timmerman R, Zimmermann F, Stojkovski I, Jeremic B. Stereotactic radiotherapy of primary lung cancer and other targets: results of consultant meeting of the International Atomic Energy Agency. Int J Radiat Oncol Biol Phys 2011;79:660-9. [PubMed]
- Timmerman R, Paulus R, Galvin J, Michalski J, Straube W, Bradley J, Fakiris A, Bezjak A, Videtic G, Johnstone D, Fowler J, Gore E, Choy H. Stereotactic body radiation therapy for inoperable early stage lung cancer. JAMA 2010;303:1070-6. [PubMed]
- Ricardi U, Filippi AR, Guarneri A, Giglioli FR, Ciammella P, Franco P, Mantovani C, Borasio P, Scagliotti GV, Ragona R. Stereotactic body radiation therapy for early stage non-small cell lung cancer: results of a prospective trial. Lung Cancer 2010;68:72-7. [PubMed]
- Lo SS, Fakiris AJ, Teh BS, Cardenes HR, Henderson MA, Forquer JA, Papiez L, McGarry RC, Wang JZ, Li K, Mayr NA, Timmerman RD. Stereotactic body radiation therapy for oligometastases. Expert Rev Anticancer Ther 2009;9:621-35. [PubMed]
- Norihisa Y, Nagata Y, Takayama K, Matsuo Y, Sakamoto T, Sakamoto M, Mizowaki T, Yano S, Hiraoka M. Stereotactic body radiotherapy for oligometastatic lung tumors. Int J Radiat Oncol Biol Phys 2008;72:398-403. [PubMed]
- Henderson MA, Hoopes DJ, Fletcher JW, Lin PF, Tann M, Yiannoutsos CT, Williams MD, Fakiris AJ, McGarry RC, Timmerman RD. A pilot trial of serial 18F-fluorodeoxyglucose positron emission tomography in patients with medically inoperable stage I non-small-cell lung cancer treated with hypofractionated stereotactic body radiotherapy. Int J Radiat Oncol Biol Phys 2010;76:789-95. [PubMed]
- Ebright MI, Russo GA, Gupta A, Subramaniam RM, Fernando HC, Kachnic LA. Positron emission tomography combined with diagnostic chest computed tomography enhances detection of regional recurrence after stereotactic body radiation therapy for early stage non-small cell lung cancer. J Thorac Cardiovasc Surg 2013;145:709-15. [PubMed]
- Therasse P, Arbuck SG, Eisenhauer EA, Wanders J, Kaplan RS, Rubinstein L, Verweij J, Van Glabbeke M, van Oosterom AT, Christian MC, Gwyther SG. New guidelines to evaluate the response to treatment in solid tumors. European Organization for Research and Treatment of Cancer, National Cancer Institute of the United States, National Cancer Institute of Canada. J Natl Cancer Inst 2000;92:205-16. [PubMed]
- Eisenhauer EA, Therasse P, Bogaerts J, Schwartz LH, Sargent D, Ford R, Dancey J, Arbuck S, Gwyther S, Mooney M, Rubinstein L, Shankar L, Dodd L, Kaplan R, Lacombe D, Verweij J. New response evaluation criteria in solid tumours: revised RECIST guideline (version 1.1). Eur J Cancer 2009;45:228-47. [PubMed]
- Park KJ, Chung JY, Chun MS, Suh JH. Radiation-induced lung disease and the impact of radiation methods on imaging features. Radiographics 2000;20:83-98. [PubMed]
- Huang K, Dahele M, Senan S, Guckenberger M, Rodrigues GB, Ward A, Boldt RG, Palma DA. Radiographic changes after lung stereotactic ablative radiotherapy (SABR)--can we distinguish recurrence from fibrosis? A systematic review of the literature. Radiother Oncol 2012;102:335-42. [PubMed]
- Aoki T, Nagata Y, Negoro Y, Takayama K, Mizowaki T, Kokubo M, Oya N, Mitsumori M, Hiraoka M. Evaluation of lung injury after three-dimensional conformal stereotactic radiation therapy for solitary lung tumors: CT appearance. Radiology 2004;230:101-8. [PubMed]
- Libshitz HI, Shuman LS. Radiation-induced pulmonary change: CT findings. J Comput Assist Tomogr 1984;8:15-9. [PubMed]
- Linda A, Trovo M, Bradley JD. Radiation injury of the lung after stereotactic body radiation therapy (SBRT) for lung cancer: a timeline and pattern of CT changes. Eur J Radiol 2011;79:147-54. [PubMed]
- Palma DA, van Sörnsen de Koste JR, Verbakel WF, Senan S. A new approach to quantifying lung damage after stereotactic body radiation therapy. Acta Oncol 2011;50:509-17. [PubMed]
- Takeda A, Kunieda E, Takeda T, Tanaka M, Sanuki N, Fujii H, Shigematsu N, Kubo A. Possible misinterpretation of demarcated solid patterns of radiation fibrosis on CT scans as tumor recurrence in patients receiving hypofractionated stereotactic radiotherapy for lung cancer. Int J Radiat Oncol Biol Phys 2008;70:1057-65. [PubMed]
- Thirion JP. Image matching as a diffusion process: an analogy with Maxwell's demons. Med Image Anal 1998;2:243-60. [PubMed]
- Wang H, Dong L, Lii MF, Lee AL, de Crevoisier R, Mohan R, Cox JD, Kuban DA, Cheung R. Implementation and validation of a three-dimensional deformable registration algorithm for targeted prostate cancer radiotherapy. Int J Radiat Oncol Biol Phys 2005;61:725-35. [PubMed]
- Ma J, Zhang J, Zhou S, Hubbs JL, Foltz RJ, Hollis DR, Light KL, Wong TZ, Kelsey CR, Marks LB. Regional lung density changes after radiation therapy for tumors in and around thorax. Int J Radiat Oncol Biol Phys 2010;76:116-22. [PubMed]
- Chi A, Tomé WA, Fowler J, Komaki R, Nguyen NP, Mehta MP, Welsh JS. Stereotactic body radiation therapy in non-small-cell lung cancer: linking radiobiological modeling and clinical outcome. Am J Clin Oncol 2011;34:432-41. [PubMed]
- Mattonen SA, Palma DA, Haasbeek CJ, Senan S, Ward AD. Distinguishing radiation fibrosis from tumour recurrence after stereotactic ablative radiotherapy (SABR) for lung cancer: a quantitative analysis of CT density changes. Acta Oncol 2013;52:910-8. [PubMed]
- Donaldson SS. The power of partnerships: a message for all radiologists. Radiology 2014;271:315-9. [PubMed]