Mean apparent diffusion coefficient values in defining radiotherapy planning target volumes in glioblastoma
Background
Glioblastoma (GBM) is the most common and deadly primary brain tumor (1). Accounting for 20% of all intracranial tumors, the average life expectancy after diagnosis remains 14 months despite intense research and significant advances in therapy and imaging (1,2) The current standard of care in patients with newly diagnosed GBM involves surgical resection, oral temozolomide chemotherapy and targeted radiation therapy (2,3). Nevertheless, GBM often recurs or progresses following adjuvant therapy because of the topographically diffuse and infiltrative nature of the disease (2,4).
Magnetic resonance (MR) of the brain has evolved into an essential tool with a multi-parametric approach that allows for noninvasive characterization and surveillance of these aggressive tumors. Pre- and post-radiotherapy MR is a standard means of assessing for tumor recurrence and determining radiation planning target volumes (1). Pre-treatment radiation planning is primarily based on contoured volumes generated on the MR T1-weighted post contrast and T2/fluid-attenuated inversion recovery (FLAIR) sequences with a small margin around the abnormal region (1).
A major challenge in treating GBM is distinguishing the extent of tumor from other inflammatory processes such as peritumoral edema, treatment-induced pseudoprogression or radiation necrosis (2,5,6). Conventional T1-weighted post contrast and T2/FLAIR MR sequences are widely utilized to assess tumor extent and define the radiation target volume for radiotherapy. Pseudoprogression, like tumor recurrence, can appear as abnormally increased T1-weighted post gadolinium signal on MR sequences within 2 months of radiotherapy but in contrast to new tumor typically recovers or stabilizes spontaneously over serial exams (2,7). Similarly, radiation necrosis can appear as late enhancement 3-12 months after radiotherapy and can be confused with new tumor recurrence, but reflects treatment-induced cell death of brain tissue and may not resolve over time (2). Since suspected peritumoral edema on T2/FLAIR sequences is not always included in the radiation planning target volumes, it is critically important to correctly identify tumor from other similar appearing but benign signal abnormalities.
Several physiology-based MR imaging methods show promise in discriminating between tumor and benign altered tissue. Diffusion-weighted imaging (DWI) utilizes the motion of water protons in tissues, and can generate quantitative measures including the apparent diffusion coefficient (ADC) to differentiate tumor from surrounding tissue based on the microstructure and cellular integrity of the tissue (5,8). The DWI sequence with ADC maps is almost universally acquired on MR brain exams but these are not widely used to define radiation target volumes for radiotherapy. Necrosis, whether caused by chemoradiotherapy or tumor growth, degrades normal cellular structure to varying degrees and increases the ADC of tissue (9). Several prior studies have reported ADC values in neoplastic tissue, peritumoral edema or post-treatment change, and normal head and neck tissue (8,10-12), and these studies have generally found on post-treatment MR exams that mean ADC values of tumor recurrence fall between the mean ADC values of normal brain tissue and peritumoral or post-treatment tissue changes (Figure 1).
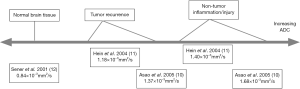
However, few studies have evaluated ADC values of the same tissue in relation to the pre- and post-treatment MR exams. In this investigation, we hypothesized that the brain tissue in regions of future tumor recurrence have pre-treatment ADC values different from those of other similar regional tissue despite a lack of visible differences on post gadolinium T1-weighted MR sequences. Our aim was to determine whether ADC values on pre-treatment MR exams could be associated with sites of future tumor recurrence, which would have significant implications for the incorporation of ADC mapping into pre-radiation planning and the current treatment of GBM.
Methods
Patient population
All patients with pathology proven GBM who received radiation therapy and had tumor recurrence on follow up MR exams at our institution between January 1, 2009 and May 31, 2012 were included in this single-center retrospective cohort study. Institutional Review Board approval was obtained and waiver of consent was granted. Data was acquired in compliance with all applicable Health Insurance Portability and Accountability Act regulations. A database of patients who had MR brain exams was generated from our institution’s Picture Archiving and Communications System (PACS), General Electric Centricity database and cross-referenced with a pathology database for all patients with histopathology confirmed diagnosis of GBM according to the World Health Organization classification system. Medical records were reviewed to identify patients who received standard external beam radiation therapy at our institution and had baseline (post-surgical, pre-radiotherapy) MR and a minimum of 2 follow up MR scans at least 6 months after radiation therapy. Patients were excluded who did not receive radiation treatment for GBM at our institution or who did not have adequate post-treatment follow up MR imaging.
During the study period 110 adult patients with pathology proven GBM were identified. Of these, 20 patients met inclusion criteria (Figure 2). Fourty-six patients were excluded because they did not receive radiation treatment at our institution. Additionally, 16 patients were excluded who received radiation therapy but did not have adequate post-treatment follow up imaging, while 20 patients had no evidence of tumor recurrence on serial exams until the end of the study period. Eight patients were excluded because they had regression of residual tumor on follow up imaging, rather than tumor recurrence. Among the patients who met inclusion criteria, a review of medical charts was conducted to obtain clinical information and pre- and post-treatment imaging exams were analyzed. The 20 included patients underwent MRI within 24 hours following surgery for initial baseline postoperative evaluation. This exam was utilized as the pre-radiotherapy baseline MRI in this study. The included patients had radiation therapy 30±11 days after surgical resection.
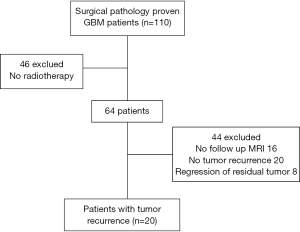
For this study, a patient was deemed to have tumor recurrence on MR exams if there was nodular enhancement on post radiotherapy T1-weighted post contrast sequence which was new or increased by greater than 25% on the 6 months follow up MR exam with continued increase on an additional follow up exam. Histologic sampling to prove recurrence was not available in these subjects. Therefore, serial follow up exams showing continued increase in enhancing tumor outside the expected timeframe for pseudo-progression, allowed diagnosis of recurrence over treatment related changes. In addition, each of these patients was reviewed at a multidisciplinary tumor board and underwent a consensus change in clinical management based on radiologic evidence of recurrence. Increased non-enhancing T2/FLAIR lesions were not selected as sites for ADC comparison given that ADC is often used to confirm this tissue as tumor. Each of the 20 patients in this study had enhancing lesions that progressed on serial follow-up exams. None of the excluded patients had increased non enhancing tumor on follow-up exams. Although the 1990 MacDonald criteria defined tumor progression as new or increasing enhancement on serial imaging, the updated response criteria recommended by the Response Assessment in Neuro-Oncology (RANO) Working Group stated progression could only be defined with new enhancement if the imaging exam was greater than 12 weeks after completion of chemoradiotherapy (13,14). Given the possibility of early confounding treatment related changes, we chose to include only patients who had a minimum of two follow up MR exams at least 6 months post-treatment. Serial imaging and chart review also confirmed that no corresponding clinical acute or subacute stroke was present that could explain the low ADC lesions.
MR and data acquisition
All MR examinations were performed on a 1.5-T imaging system (General Electric Medical Systems; Siemens Medical Solutions) or a 3.0-T imaging system (Siemens Medical Solutions) using standard pulse sequences. Conventional imaging sequences (model parameters noted with similar scan parameters between machines), including T1-weighted spin echo [TE/TR 14/366, slice thickness =1.6 mm, number of excitations (NEX) =6, matrix size =256×192, and field of view (FOV) =22 cm], T2-weighted fast spin-echo (TE/TR 110/3,400, slice thickness =1.6 mm, NEX =6, matrix size =256×192, and FOV =22 cm), FLAIR (TI =2,250 ms, TE/TR 144/11,000, slice thickness =3 mm, NEX =6, matrix size =256×192, and FOV =22 cm), and DW images (single-shot echo-planar, TE/TR 90/3,600, slice thickness =5 mm, matrix size =128×128, FOV =22 cm, b-value =1,000 s/mm2, one volume with no diffusion weighting followed by three volumes with diffusion weighting along one of the three orthogonal axes) were obtained during each examination. ADC maps were generated by the scanner by estimating the diffusion coefficient along each of the three orthogonal directions and averaging these values.
Sites of tumor recurrence on post-radiotherapy T1-weighted post contrast sequences were transferred to corresponding sites on pre-radiotherapy T1-weighted post contrast and ADC sequences utilizing the AW workstation Integrated Registration tool (General Electric Healthcare, Waukesha, WI, USA). Sites of ROI measurement were determined independently on T1-weighted post contrast sequences and transferred to the ADC maps to minimize introduction of bias. Quantitative ADC, T1, and T2 values were recorded on this pre-radiotherapy exam.
Surrounding background sites that did not give rise to future tumor were chosen to match the type of tissue which gave rise to future tumor [i.e., T2 hyperintensity, normal appearing white matter (WM), or normal appearing gray matter (GM)]. The AW workstation quantitative thresholding tool (General Electric Healthcare, Waukesha, WI, USA) was utilized to select a region with T1-weighted pre-contrast and T2-weighted signal intensity values within 10% of those recorded for sites of future tumor. This technique yielded regions with targeted signal intensities, and the site closest to the tumor recurrence and also located within the same radiation dosage region (60 Gy, 46–60 Gy, or outside 46 Gy) was selected. These sites were targeted on the post therapy T2/FLAIR sequence with similar registration and cross referencing, and ADC, T1, and T2 quantitative measurements were recorded on pre-radiotherapy exam and these values were compared to values obtained at future tumor and sites.
Future tumor site selection and mean ADC measurements were performed by two experienced radiologists following the above methods. The mean ADC measurements for sites of future tumor recurrence and T1/T2 signal intensity matched background tissue were compared for interobserver variability.
Radiation planning target volume determination utilized T1-weighted post contrast and T2/FLAIR MR images on post-surgical/pre-radiation exams. The 46 Gy volume included T2/FLAIR abnormal regions with a 2 cm clinical target volume and 0.3–0.5 cm expansion. The 60 Gy volumes included the resection cavity, residual enhancement, a 2 cm clinical target volume, and 0.3–0.5 cm expansion. Recurrence was considered outside of the planning target volume if there was definitive tumor located completely outside of the 60 Gy isodense line on post radiation follow up MR exam, which implied the site of recurrence did not receive the full 60 Gy dose.
Data analysis and statistical methods
The sample size was predetermined by the number of subjects meeting the inclusion criteria who had MR performed and available in our institution’s PACS database. The primary endpoint used for this study was the difference in the mean ADC values of tissue at sites of future tumor recurrence on pre-radiation MR exams compared to mean ADC values of T1- and T2-signal intensity-matched tissue that did not go on to tumor recurrence. A two-sided Wilcoxon signed-rank test was used to compare for significant differences between the continuous variables for nonparametric dependent samples. Intraclass correlation was used to compare for interobserver variability. SPSS statistical software package, version 20 (IBM, Chicago, IL, USA), was used for calculations and box plots. A P<0.05 was considered statistically significant.
Results
Baseline patient characteristics and demographics for the twenty patients included in this study are listed in Table 1. The mean age (± standard deviation) was 56.9±13.2 years, and 8/20 patients (40%) were male. The locations of the patients’ primary GBM lesion varied, consistent with the topographically diffuse nature of the disease. Four of the 20 patients (20%) had tumor recurrence outside of the 60 Gy isodense line. Twelve had recurrence within previous regions of T2 hyperintensity, seven had recurrence within previously normal appearing WM, and one had recurrence in previously normal GM (Tables 2,3). Representative tumor recurrences on pre- and post-radiotherapy MR exams outside the planning target volume (Figure 3) are shown.
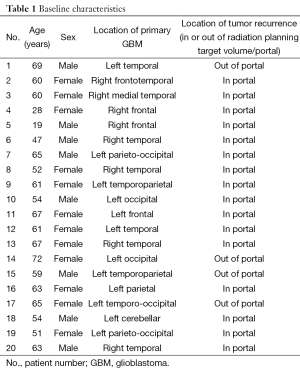
Full table
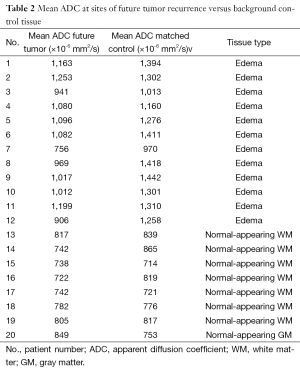
Full table
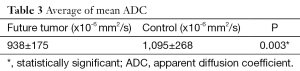
Full table
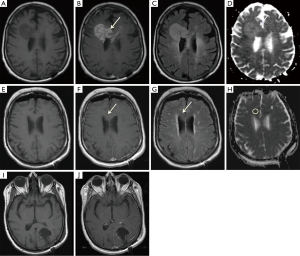
All twenty patients included in this study underwent surgical resection of their GBM prior to the initiation of radiation treatment. Upon review of medical records, surgeons reported a total gross resection for 12 of the 20 patients (60%). In the eight subjects with subtotal resection, the surgeon reported complete or near complete resection of visible tumor in each of these cases. Early postoperative MR in the subtotal resection group showed small amounts of nodular enhancement around the resection cavity in all eight and thick linear enhancement also present in 3/8 cases which were compatible with residual tumor.
Eighteen of the 20 patients (90%) also received initial chemotherapy with oral temozolomide. One patient did not receive chemotherapy secondary to liver failure. Another patient did not receive chemotherapy nor complete radiation treatment secondary to acute hospitalizations. Seventeen of 20 (85%) completed standard radiation treatment with 60 Gy. Mortality data and second line chemotherapy data were not collected for this investigation.
The mean ADC value of pre-radiotherapy brain tissue in regions of future tumor recurrence was significantly lower than mean ADC in control regions matched for T1- and T2-weighted signal intensity (938±175×10−6 mm2/s vs. 1,095±268×10−6 mm2/s, P=0.003), despite the lack of any visible abnormalities on post gadolinium T1-weighted MR sequences (Tables 2,3). There was no significant difference in T1- or T2-weighted signal intensity values (P=0.50 and P=0.10, respectively) between the regions that went on to tumor recurrence versus background sites that did not. Figure 4 shows box and whisker plots of the mean ADC values for the two sets of ROIs. While the differences are statistically significant, there is substantial overlap of mean ADC values between the two groups when all patients are considered together (Figure 4A); however, the overlap is clearly lessened by categorizing the patients according to the type of tissue from where the tumor recurred, i.e., from T2-hyperintense or normal appearing WM (Figure 4B,C) Specifically, the mean ADC differences was substantially lower when recurrent tumor arose from T2-hyperintense WM, which was a majority of cases (60%), P=0.002. No significant mean ADC difference was seen when recurrent tumor arose from background normal appearing WM.
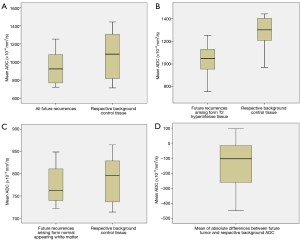
There was strong interobserver agreement with intraclass correlation of 0.867 and P<0.0001 for the pretreatment mean ADC measurements performed independently by two radiologists.
Radiation dosage for sites of future tumor recurrence were recorded as within the 60 Gy volume (n=16), outside 60 Gy but within the 46 Gy volume (n=2), or outside 46 Gy volume (n=2). Corresponding background sites were selected within the same radiation dosage region for each patient.
Discussion
Our study showed a statistically significant difference in pre-radiotherapy mean ADC between brain tissue that goes on to tumor recurrence and other, similar-appearing tissue that does not. To our knowledge, this is the first GBM study that quantitatively compares pre-treatment mean ADC values at sites that gave rise to new tumor to similar-appearing tissue not giving rise to tumor. This data coincides with results from previous studies which compared ADC values of different tissues on post-radiotherapy treatment MR exams and found reduced ADC measures in areas of tumor recurrence (5,10,11).
Although RANO criteria also account for the T2 non-enhancing component of tumor, in this study, only new enhancing tumor that serially increased from a site of T2 hyperintensity were selected as sites of recurrence. ADC is inherent to defining the presence of non-enhancing tumor and therefore these sites were not chosen as targets for this study.
A recent study by Elson et al. (15) showed that regions of ADC hypointensity on pre-radiotherapy exams correlated with sites of gross tumor recurrence on post-therapy exams in 88% of their cases. Their study also reported that pre-radiation ADC hypointensity regions were 95% covered within the 60 Gy isodense line assigned on pre-radiation exam (15). Their study qualitatively evaluated ADC hypointensity and qualitatively assessed T1 post-contrast and T2 signal abnormalities for correlating enhancement and T2 shine-through. Our study complements their findings by reporting quantitative ADC measurements on each of our 20 subjects and comparing ADC values to that of surrounding background tissue. In our study, ADC values were compared to similar surrounding tissue without significant differences in T1 pre-contrast or T2 values. This suggests that ADC can be abnormal even without gross T1 and T2 quantitative disturbances.
Methylation of the DNA repair enzyme O6-methylguanine-DNA methyltransferase (MGMT) is a significant biomarker in GBM which can support the presence of true recurrence over pseudoprogression (16). However, all cases in this study were determined to be tumor recurrence 6 months post therapy with available serial MR exam data by two independent reviewers. Therefore, the role for MGMT methylation status in evaluation for pseudoprogression was limited in this study.
ADC differentiates tissues based on the rate of water movement and cellular integrity of tissue, and research has shown that ADC correlates well with the cellularity of tumors seen on histologic examinations (2,17). It is physiologically plausible that the cellular integrity of a tumor cell will differ with that of normal brain cells, post-operative edema or post-chemoradiotherapy pseudoprogression, or post-treatment cells undergoing necrosis. Malignant cells are hypercellular with enlarged nuclei and hyperchromatism, all affecting the ADC measure (8).
Our data may be explained by decreased mean ADC values representing non-enhancing tumor prior to gross recurrence. The large overlap in our whole group ADC values is significantly reduced when only recurrence arising from T2 hyperintensity (n=12/20) is compared to similar background. A previous study showed that isolated restricted diffusion can precede development of enhancing tumor in a subset of patients with GBM (18). It is possible that these sites in our study represent isolated non-enhancing T2 hyperintense tumor with restricted diffusion that developed enhancement on follow up exams.
An alternative explanation for our results may be related to treatment related tissue hypoxia and necrosis. Tissues at the periphery of tumors demonstrating low ADC values on post therapy exams have been previously shown to represent tissue hypoxia related to response to therapy (19). A study comparing DWI and PET/CT utilizing a hypoxia agent has shown that hypoxia within brain tumor tissues correlate with low ADC values (20). In the available cases from our study, no decreased cerebral blood flows or cerebral blood volumes were identified at future recurrence sites that would suggest hypoxia alone accounted for lowered ADC values. Further research may elucidate the mechanism of low ADC values on pre-treatment exams as increased cellularity versus hypoxia within tumor or a combination of both phenomena.
Multiple strategies have been employed to assess ADC data in GBM evaluation. Mean ADC values have been well documented as important MR data to support the presence of GBM tumor or recurrence on a given exam (10,11,21). Although earlier studies demonstrated the use of minimum ADC as an important predictor in overall survival in treated GBM (22), a more recent study showed that minimum ADC correlates poorly with the most aggressive component of high grade gliomas, possibly due to factors such as tissue compression or ischemia (23). Gradients of ADC values that increased with distance away from enhancing tumor allow differentiation of GBM from metastatic lesions (24). Metastatic lesions were shown to have homogenous ADC gradient values with increasing distance from enhancing tumor whereas GBM had increasing ADC values (24). ADC histograms can be generated by plotting ADC values of individual voxels within segmented volumes which offers a more complete analysis of the ADC spectrum within a region of interest. Studies have shown ADC histogram analysis to be an important predictor of patient survival with treatment of bevacizumab (9,25-27).
Previous studies have concluded that advanced MR imaging techniques may help differentiate tissue on post-treatment MR exams, which can prevent delays in treatment of tumor recurrence, improve prognosis and avoid continuation of ineffective treatment (7,11). Our study suggests that ADC values may be helpful even before the initiation of radiotherapy in the stages of radiation target volume determination. As noted, tumor recurrence occurred outside the radiation planning target volume in 20% of our study patients. A prior investigation that examined pre-treatment ADC values concluded that ADC analysis could stratify progression-free survival in recurrent GBM (9). Our study suggests that the use of ADC values in conjunction with conventional T1 and T2/FLAIR MR sequences could better identify an appropriate radiation planning target volume for radiation therapy. Moreover, ADC maps are commonly available on routine MR brain exams and can be easily incorporated into potential treatment planning.
There were several limitations to this investigation. The study sample size was limited by the number of subjects who received their treatment and had the appropriate MR imaging performed and available in our institution’s PACS database. From the initial 110 patients screened for the study, only twenty were included. Most notably, mortality data was not collected for the initial 110 patients screened. Given the average life expectancy of 14 months, it is possible that the twenty patients who survived long enough to meet the inclusion criteria were healthier than the patients who were excluded. Given the small sample size and retrospective nature of this investigation, validating our findings in a larger sample size with adequate power or through a future prospective study would be useful. A larger study could suggest a pretherapy mean ADC threshold value that prospectively predicts future tumor recurrence.
This study was also limited in that tumor recurrence was not defined by histologic evidence. However, tumor recurrence was determined by MR evidence on two follow up exams at least 6 months post radiotherapy in addition to consensus change in clinical management based on multidisciplinary tumor board review. Similar evidence for tumor recurrence has been used by previous authors when histologic evidence was unavailable (15,28).
The subject group in this study was heterogeneous in composition (12/20 gross total resections and 8/20 subtotal resections) which could affect the baseline ADC values at sites of residual disease. However, the recurrence sites selected for quantitative comparison in this study were separate from the nodular or thick linear enhancing tissue which could be considered residual disease in order to limit the effects of residual disease on ADC analysis. In addition, ADC values are also affected by the postoperative resection cavity and sites of associated hemorrhage. Sites of recurrence were not selected in regions of possible hemorrhage, within the resection cavity, or within a thin rim of enhancement at the periphery of the resection cavity.
Previous studies have shown that nodular and thick linear enhancement patterns and higher residual tumor volume on early post-operative MR have been associated with increased recurrence rates and lower survival (29-31). However, all 20 in our cohort had evidence of new nodular enhancing tumor on serial imaging at previous sites without enhancing tumor, regardless of post-operative residual tumor status. Future studies with a larger sample size could elucidate whether similar tumor recurrence after subtotal vs. gross total resection yields differences in prognosis.
A technical limitation involves the ADC region of interest selection. Currently, there is no standardized way to determine the region of interest from which to acquire ADC measurements, although our methods were similar to a previous study examining ADC values in GBM (18). A future study with automated segmentation of low ADC regions for analysis would decrease the possibility of bias in ROI selection and would increase sensitivity in detecting clinically relevant regions on pre-therapy exams.
Images included in this study were collected from different MR scanners. However, ADC should not depend on field strength or differences in the pulse sequences, so this should not have significantly affected the outcome of this study. In addition, ADC values were only compared to values within the same exam and analyzed as paired data which further reduces variability of utilizing multiple scanners.
Conclusions
In conclusion, our study showed a significant association between mean ADC values on pre-treatment MR exams and sites of future tumor recurrence. Pre-radiotherapy mean ADC at sites of future tumor recurrence were significantly lower than the mean ADC in T1 and T2 matched control tissue not progressing to tumor. Future investigations with larger sample sizes could prospectively correlate sites of low ADC on pre-therapy exams with the development of tumor recurrence on follow up evaluations. In addition, automated segmentation of low ADC signal could reduce manual ROI selection bias and increase prospective applicability of these results. Increasing the clinical integration of ADC measurements in radiotherapy planning may alter and advance the treatment of GBM.
Acknowledgements
None.
Footnote
Conflicts of Interest: The Research described in this was primarily performed at Department of Diagnostic Radiology, Rush University Medical Center, 1653 W. Congress Parkway, Chicago, IL 60612, USA.
References
- Van Meir EG, Hadjipanayis CG, Norden AD, Shu HK, Wen PY, Olson JJ. Exciting new advances in neuro-oncology: the avenue to a cure for malignant glioma. CA Cancer J Clin 2010;60:166-93. [PubMed]
- Yang I, Aghi MK. New advances that enable identification of glioblastoma recurrence. Nat Rev Clin Oncol 2009;6:648-57. [PubMed]
- Bloch O, Han SJ, Cha S, Sun MZ, Aghi MK, McDermott MW, Berger MS, Parsa AT. Impact of extent of resection for recurrent glioblastoma on overall survival: clinical article. J Neurosurg 2012;117:1032-8. [PubMed]
- Holland EC. Glioblastoma multiforme: the terminator. Proc Natl Acad Sci U S A 2000;97:6242-4. [PubMed]
- Al Sayyari A, Buckley R, McHenery C, Pannek K, Coulthard A, Rose S. Distinguishing recurrent primary brain tumor from radiation injury: a preliminary study using a susceptibility-weighted MR imaging-guided apparent diffusion coefficient analysis strategy. AJNR Am J Neuroradiol 2010;31:1049-54. [PubMed]
- Kleijn A, Chen JW, Buhrman JS, Wojtkiewicz GR, Iwamoto Y, Lamfers ML, Stemmer-Rachamimov AO, Rabkin SD, Weissleder R, Martuza RL, Fulci G. Distinguishing inflammation from tumor and peritumoral edema by myeloperoxidase magnetic resonance imaging. Clin Cancer Res 2011;17:4484-93. [PubMed]
- Gahramanov S, Muldoon LL, Varallyay CG, Li X, Kraemer DF, Fu R, Hamilton BE, Rooney WD, Neuwelt EA. Pseudoprogression of glioblastoma after chemo- and radiation therapy: diagnosis by using dynamic susceptibility-weighted contrast-enhanced perfusion MR imaging with ferumoxytol versus gadoteridol and correlation with survival. Radiology 2013;266:842-52. [PubMed]
- Abdel Razek AA, Kandeel AY, Soliman N, El-shenshawy HM, Kamel Y, Nada N, Denewar A. Role of diffusion-weighted echo-planar MR imaging in differentiation of residual or recurrent head and neck tumors and posttreatment changes. AJNR Am J Neuroradiol 2007;28:1146-52. [PubMed]
- Pope WB, Kim HJ, Huo J, Alger J, Brown MS, Gjertson D, Sai V, Young JR, Tekchandani L, Cloughesy T, Mischel PS, Lai A, Nghiemphu P, Rahmanuddin S, Goldin J. Recurrent glioblastoma multiforme: ADC histogram analysis predicts response to bevacizumab treatment. Radiology 2009;252:182-9. [PubMed]
- Asao C, Korogi Y, Kitajima M, Hirai T, Baba Y, Makino K, Kochi M, Morishita S, Yamashita Y. Diffusion-weighted imaging of radiation-induced brain injury for differentiation from tumor recurrence. AJNR Am J Neuroradiol 2005;26:1455-60. [PubMed]
- Hein PA, Eskey CJ, Dunn JF, Hug EB. Diffusion-weighted imaging in the follow-up of treated high-grade gliomas: tumor recurrence versus radiation injury. AJNR Am J Neuroradiol 2004;25:201-9. [PubMed]
- Sener RN. Diffusion MRI: apparent diffusion coefficient (ADC) values in the normal brain and a classification of brain disorders based on ADC values. Comput Med Imaging Graph 2001;25:299-326. [PubMed]
- Macdonald DR, Cascino TL, Schold SC Jr, Cairncross JG. Response criteria for phase II studies of supratentorial malignant glioma. J Clin Oncol 1990;8:1277-80. [PubMed]
- Wen PY, Macdonald DR, Reardon DA, Cloughesy TF, Sorensen AG, Galanis E, Degroot J, Wick W, Gilbert MR, Lassman AB, Tsien C, Mikkelsen T, Wong ET, Chamberlain MC, Stupp R, Lamborn KR, Vogelbaum MA, van den Bent MJ, Chang SM. Updated response assessment criteria for high-grade gliomas: response assessment in neuro-oncology working group. J Clin Oncol 2010;28:1963-72. [PubMed]
- Elson A, Bovi J, Siker M, Schultz C, Paulson E. Evaluation of absolute and normalized apparent diffusion coefficient (ADC) values within the post-operative T2/FLAIR volume as adverse prognostic indicators in glioblastoma. J Neurooncol 2015;122:549-58. [PubMed]
- Hegi ME, Liu L, Herman JG, Stupp R, Wick W, Weller M, Mehta MP, Gilbert MR. Correlation of O6-methylguanine methyltransferase (MGMT) promoter methylation with clinical outcomes in glioblastoma and clinical strategies to modulate MGMT activity. J Clin Oncol 2008;26:4189-99. [PubMed]
- Lee EJ, terBrugge K, Mikulis D, Choi DS, Bae JM, Lee SK, Moon SY. Diagnostic value of peritumoral minimum apparent diffusion coefficient for differentiation of glioblastoma multiforme from solitary metastatic lesions. AJR Am J Roentgenol 2011;196:71-6. [PubMed]
- Gupta A, Young RJ, Karimi S, Sood S, Zhang Z, Mo Q, Gutin PH, Holodny AI, Lassman AB. Isolated diffusion restriction precedes the development of enhancing tumor in a subset of patients with glioblastoma. AJNR Am J Neuroradiol 2011;32:1301-6. [PubMed]
- Morse DL, Galons JP, Payne CM, Jennings DL, Day S, Xia G, Gillies RJ. MRI-measured water mobility increases in response to chemotherapy via multiple cell-death mechanisms. NMR Biomed 2007;20:602-14. [PubMed]
- Hino-Shishikura A, Tateishi U, Shibata H, Yoneyama T, Nishii T, Torii I, Tateishi K, Ohtake M, Kawahara N, Inoue T. Tumor hypoxia and microscopic diffusion capacity in brain tumors: a comparison of (62)Cu-Diacetyl-Bis (N4-Methylthiosemicarbazone) PET/CT and diffusion-weighted MR imaging. Eur J Nucl Med Mol Imaging 2014;41:1419-27. [PubMed]
- Di Costanzo A, Scarabino T, Trojsi F, Popolizio T, Bonavita S, de Cristofaro M, Conforti R, Cristofano A, Colonnese C, Salvolini U, Tedeschi G. Recurrent glioblastoma multiforme versus radiation injury: a multiparametric 3-T MR approach. Radiol Med 2014;119:616-24. [PubMed]
- Yamasaki F, Sugiyama K, Ohtaki M, Takeshima Y, Abe N, Akiyama Y, Takaba J, Amatya VJ, Saito T, Kajiwara Y, Hanaya R, Kurisu K. Glioblastoma treated with postoperative radio-chemotherapy: prognostic value of apparent diffusion coefficient at MR imaging. Eur J Radiol 2010;73:532-7. [PubMed]
- Rose S, Fay M, Thomas P, Bourgeat P, Dowson N, Salvado O, Gal Y, Coulthard A, Crozier S. Correlation of MRI-derived apparent diffusion coefficients in newly diagnosed gliomas with [18F]-fluoro-L-dopa PET: what are we really measuring with minimum ADC? AJNR Am J Neuroradiol 2013;34:758-64. [PubMed]
- Lemercier P, Paz Maya S, Patrie JT, Flors L, Leiva-Salinas C. Gradient of apparent diffusion coefficient values in peritumoral edema helps in differentiation of glioblastoma from solitary metastatic lesions. AJR Am J Roentgenol 2014;203:163-9. [PubMed]
- Ellingson BM, Sahebjam S, Kim HJ, Pope WB, Harris RJ, Woodworth DC, Lai A, Nghiemphu PL, Mason WP, Cloughesy TF. Pretreatment ADC histogram analysis is a predictive imaging biomarker for bevacizumab treatment but not chemotherapy in recurrent glioblastoma. AJNR Am J Neuroradiol 2014;35:673-9. [PubMed]
- Pope WB, Qiao XJ, Kim HJ, Lai A, Nghiemphu P, Xue X, Ellingson BM, Schiff D, Aregawi D, Cha S, Puduvalli VK, Wu J, Yung WK, Young GS, Vredenburgh J, Barboriak D, Abrey LE, Mikkelsen T, Jain R, Paleologos NA, Rn PL, Prados M, Goldin J, Wen PY, Cloughesy T. Apparent diffusion coefficient histogram analysis stratifies progression-free and overall survival in patients with recurrent GBM treated with bevacizumab: a multi-center study. J Neurooncol 2012;108:491-8. [PubMed]
- Rahman R, Hamdan A, Zweifler R, Jiang H, Norden AD, Reardon DA, Mukundan S, Wen PY, Huang RY. Histogram analysis of apparent diffusion coefficient within enhancing and nonenhancing tumor volumes in recurrent glioblastoma patients treated with bevacizumab. J Neurooncol 2014;119:149-58. [PubMed]
- Elson A, Paulson E, Bovi J, Siker M, Schultz C, Laviolette PS. Evaluation of pre-radiotherapy apparent diffusion coefficient (ADC): patterns of recurrence and survival outcomes analysis in patients treated for glioblastoma multiforme. J Neurooncol 2015;123:179-88. [PubMed]
- Majós C, Cos M, Castañer S, Gil M, Plans G, Lucas A, Bruna J, Aguilera C. Early post-operative magnetic resonance imaging in glioblastoma: correlation among radiological findings and overall survival in 60 patients. Eur Radiol 2015. [Epub ahead of print]. [PubMed]
- Chaichana KL, Cabrera-Aldana EE, Jusue-Torres I, Wijesekera O, Olivi A, Rahman M, Quinones-Hinojosa A. When gross total resection of a glioblastoma is possible, how much resection should be achieved? World Neurosurg 2014;82:e257-65. [PubMed]
- Grabowski MM, Recinos PF, Nowacki AS, Schroeder JL, Angelov L, Barnett GH, Vogelbaum MA. Residual tumor volume versus extent of resection: predictors of survival after surgery for glioblastoma. J Neurosurg 2014;121:1115-23. [PubMed]