Optimization and comparison of two practical dual-tuned birdcage configurations for quantitative assessment of articular cartilage with sodium magnetic resonance imaging
Introduction
Osteoarthritis (OA) is a degenerative joint disease with a high prevalence in the aging population. The early stages of OA can be associated with a reduction in glycosaminoglycan (GAG) concentration, changes in the size and organization of collagen fibers, and increased water content (1-3). When the disease progresses, morphologic changes like thinning and lesions of the cartilage occur that can be captured by radiography and conventional magnetic resonance imaging (MRI). To maximize the effectiveness of therapeutic approaches to OA, there is high demand for imaging techniques that are sensitive to the early stages of OA. Recently, more advanced MRI techniques have been developed to detect and quantify the early molecular changes that precede the morphological changes of the cartilage. The most popular quantitative MRI techniques of this kind are delayed gadolinium-enhanced magnetic resonance imaging contrast (dGEMRIC) (4,5), T1ρ relaxation mapping (6) and sodium MRI (7-9). Although sodium MR imaging has been shown to strongly correlate with the GAG concentration in the cartilage, its implementation is still challenging because of the low sensitivity of the 23Na nucleus, low in vivo concentrations, fast transverse relaxation times and the requirement for x-nuclei compatible scanner hardware and RF coils. Dual-tuned coils provide heteronuclear images and anatomical hydrogen background images without repositioning the subject thus avoiding co-registration errors. Furthermore the hydrogen channel can be used for efficient B0 shimming, which is often required in heteronuclear imaging applications.
Due to the anatomy of the human leg, the birdcage with its cylindrical structure is well suited to perform imaging of the knee joint. Several approaches to achieve dual tuning of a birdcage structure have been proposed over the years: double tuning LC circuits on rungs (10), filters on rungs (11,12), concentric birdcages (13), four-ring birdcages (14) and birdcages with alternating low and high frequency tuned rungs (15,16). All of these designs have their advantages and weaknesses in regard to B1 field homogeneity, coil efficiency, complexity and geometric requirements. Generally, inserting tank circuits or filters into the coil structure to facilitate dual tuning is cumbersome and leads to additional losses which might be unacceptable at the low gamma nucleus. In contrast two designs, the four-ring birdcage (FRB) (14) and the alternating rungs birdcage (ARB) (15) are especially attractive under practical considerations since they combine both frequency modes in one easy-to-build structure, but don’t require additional lossy dual tuning circuits. Thus the efficiency of the low gamma nucleus is only slightly degraded compared to a single-tuned coil. To find a practical and well performing coil for future sodium and hydrogen MRI studies of the human knee, a FRB and ARB coil were designed and then characterized using workbench and MRI measurements.
Methods
Coil structures
Four-ring birdcage (FRB)
The 1.5 T 23Na/1H dual-tuned FRB coil consists of three connected, low-pass birdcages with 16 legs (Figure 1A,B). The inner 23Na birdcage is tuned at 16.8 MHz with the central capacitors Clow and acts very similar to a single-tuned low-pass birdcage. The outer birdcages are tuned with capacitors Chigh and couple through space and through the rungs to provide together a homogenous 1H mode at 63.6 MHz. A drawback often encountered with FRB structures is the increase of the axial coil length due to the outer birdcage structures. The diameter of the human thigh is considerable bigger than the diameter of the knee and the calf, thus a long coil makes it difficult to place the knee in the center of the coil without increasing the diameter of the coil. This would be detrimental to the coil efficiency due to a decreased filling factor. Additionally the increased length might cause patient discomfort. Duan et al. (17) computationally optimized the lengths of the FRB structure while taking these geometric considerations, field homogeneity and coil efficiency of both nuclei into account. Considering these simulations, the lengths of the inner and outer birdcage were set to 15 cm (linner) and 2.5 cm (louter) for the 18 cm diameter coil built in this study.
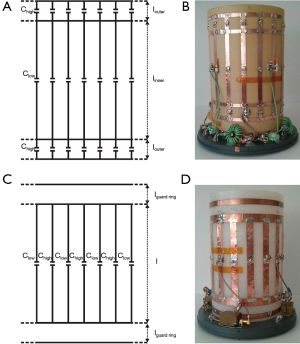
Alternating rungs birdcage (ARB)
The 1.5 T 23Na/1H dual-tuned ARB coil consists of a low-pass birdcage with 16 rungs which are alternatingly populated with capacitors Clow and Chigh to resonate the coil at 16.8 MHz and 63.6 MHz (Figure 1C,D). The length l of 15 cm and the diameter of 18 cm of the ARB were chosen to provide the same 23Na FOV as the FRB.
Materials
For both birdcage coils formers with 18 cm diameter were chosen to comfortably fit around a human knee. Some commercial knee coils can be split in two halves to allow easier patient access (18). This complicates the coil construction process and introduces additional connector losses and coil asymmetries. Thus it was decided to build the coils on closed cylinders. The diameter of 18 cm of the coils built for this study allows accessing the coils by slightly flexing the ankle which was found unproblematic. The coil structures were constructed with adhesive-back copper strips (3M, St. Paul, MN, USA) with a thickness of 35 μm. Coaxial HF-shields with a diameter of 24 cm were used to minimize the interaction of the coils with the scanner bore and avoid aliasing from the other leg. Non-magnetic capacitors (TEMEX Ceramics, Pessac, France) and variable trimmer capacitor (Voltronics, Denville, NJ, USA) were used to tune the coils.
Bench tests
An Agilent (Santa Clear, CA, USA) E5071B vector network analyzer was used to measure the S-parameters and resonance patterns.
Tuning
The capacitors required to resonate the FRB and ARB at the desired frequencies can be calculated by the equations provided in (14) and (15). In practice it is sufficient to start by calculating the capacitors needed for a single-tuned low pass birdcage at the 23Na frequency. The additional 1H tuning rungs with their low capacitance represent high impedance at the 23Na frequency and therefore have little influence on the tuning of the 23Na mode. In contrast, the capacitors of the 23Na structure represent low impedance, i.e., almost shorts at the 1H frequency. Thus, tuning of the 1H and 23Na modes is a largely independent procedure. The final capacitor values are listed in Table 1. To suppress gradient eddy currents, two equally spaced 100 nF bypass capacitors were added per end ring (19).
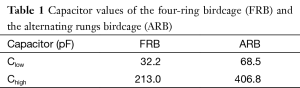
Full table
Matching and interfacing
Both coils were capacitively matched to 50 Ohm with symmetric networks. Both nuclei are driven in quadrature with custom-built quadrature hybrids. Cable traps and low/high-pass filters in the feed lines tuned to the appropriate frequencies were incorporated to ensure coil safety and stability.
MR experiments
All MR experiments were performed on a 1.5 T clinical scanner (Siemens Medical Solutions, Erlangen, Germany) with x-nuclei capability.
Acquisition of B1 maps
A cylindrical phantom (11 cm diameter) containing a saline water solution (5 g/L NaCl and 1.25 g/L NiSO4) was used to characterize the coils. B1 mapping was performed on both nuclei to assess the coil homogeneity and efficiency. To obtain high quality sodium B1 maps a phase-sensitive method (20,21) with a 3D gradient echo (GRE) readout (resolution 5×5×10 mm3, TE: 7 ms, TR: 100 ms, Bandwidth: 80 Hz/pixel, TA: 35:00 min) was used. The B1 field of the 1H channel was mapped with a 3D GRE sequence with a Bloch-Siegert shift (22) preparation (resolution 5×5×5 mm3, TE: 7 ms, TR: 20 ms, Bandwidth: 250 Hz/pixel, TA: 0:45 min).
Evaluation of the B1 field homogeneity, efficiency and signal-to-noise ratio (SNR)
To quantitatively evaluate the field homogeneity the relative uniformity (RU) within the central FOV (11 cm diameter, 8 cm length) of the B1 maps was calculated. RU represents the percentage of pixels inside the FOV whose relative deviation is not bigger than 10% of the mean B1 field Eq. [1] (23):
The B1 maps were also used to calculate the required voltage amplitude for a 180° block pulse of 1 ms length at the center of the coil. This voltage serves as a measure of the coil efficiency and SNR since B1 is direct proportional to the SNR (24).
In vivo imaging
In vivo data of a volunteer was acquired with the FRB to verify that the coil performance is sufficient to perform sodium and hydrogen imaging of the human knee. For 1H imaging a high resolution 3D MEDIC (multi-echo data imaging combination) sequence (resolution: 0.47×0.51×1.5 mm3, TR: 37 ms, TE: 20 ms, flip angle: 20°, TA: 8 min) was used. For 23Na imaging a 3D GRE dataset was acquired (resolution: 2.7×2.7×8 mm3, TR: 11.4 ms, TE: 4 ms, Bandwidth: 85 Hz/pixel, TA: 30 min). The in vivo SNR is given as the signal intensity of each pixel divided by the standard deviation of the noise of the background.
Results
After building the structures of the FRB and the ARB the coils were tuned, matched and their coupling coefficients were determined with the cylindrical phantom. Figure 2 shows the S11 plots of the FRB and ARB for 1H (span 10 MHz) and 23Na (span 5 MHz) in dB after tuning and matching the coil. All plots show well-defined resonance modes with sufficient mode separation. The worst case coupling coefficients can be found in Table 2. The goal to sufficiently match and isolate the 23Na and 1H channels was achieved without complications. To further increase the isolation between the 1H and 23Na channels to at least 20 dB, filters were added to the 23Na feed lines on both coils and 23Na filters on the ARB. For the FRB the least inter nuclei coupling was observed when the 1H and 23Na channels were 45° apart.
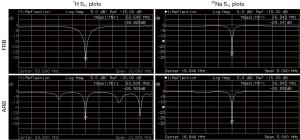
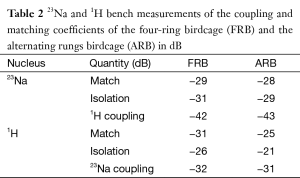
Full table
The acquired B1 maps (Figure 3) were used to calculate the RU and the 180° pulse amplitude (Table 3). The 23Na efficiencies of the two coils are almost identical (45.1 vs. 45.9 V) while the RU of the FRB is slightly higher (95.2% vs. 93.6%) than the ARB’s. Regarding the 1H performance the FRB outperforms the ARB at both efficiency (69.2 vs. 75.4 V) and field homogeneity (94.4% vs. 74.4%).
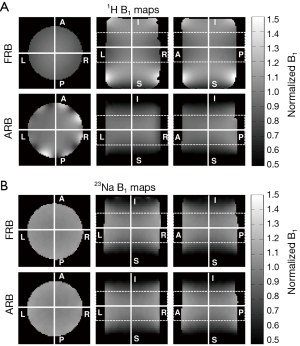
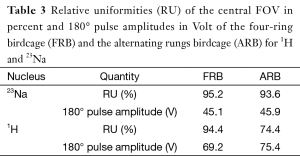
Full table
As results of the better performance in the phantom measurements, the FRB was chosen to acquire in vivo data of a volunteer. Figure 4 shows a sagittal slice of the acquired morphological 3D MEDIC data set and the 3D sodium data set. Additionally an overlay image of the segmented 23Na cartilage signal onto the 1H image was created. The 23Na SNR of the cartilage is in the range of 6 to 14.
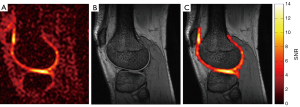
Discussion
Two practical dual-tuned quadrature birdcages for the human knee were designed and characterized. Both coils show well-defined resonance modes and the bench measurements of the matching and decoupling coefficients were well within the commonly accepted requirements (<−20 dB). Sodium and hydrogen B1 maps were acquired to investigate the efficiency and RF field homogeneity of the coils. As expected the 23Na efficiency of the FRB and the ARB is almost identical since the additional 1H rungs have little influence on the 23Na currents. The field homogeneity of a birdcage coil increases with its number of rungs. Correspondingly, the FRB with its 16 rungs is superior in field homogeneity to the ARB with its eight rungs contributing to the 23Na field as indicated by the lower RU. The imaging coverage of 1H and 23Na in the axial direction is identical for the ARB (Figure 3). Unlike, the FRB’s axial imaging coverage for 1H is considerably larger than for 23Na due to the longer length of the 1H structure (19 vs. 15 cm). This might be causing aliasing in the axial direction, if not addressed properly during image acquisition. The 23Na rungs of the ARB support counter rotating currents at the 1H frequency that have a negative impact on the 1H field homogeneity. This effect is visible as periodic B1 field attenuations near the rungs (Figure 3A). Accordingly, the FRB is superior to the ARB regarding the 1H field homogeneity and efficiency. The achieved in vivo resolution and SNR of the sodium images acquired with the FRB allow quantification of the sodium concentration of the cartilage as shown by others researchers (18). High resolution anatomical 1H images could be obtained without averaging.
Conclusions
Concluding, the ARB and FRB are both practical dual-tuned coils and deliver similar 23Na efficiency. Due to its superior 1H homogeneity and efficiency and its slightly better 23Na homogeneity, the FRB is the overall preferred coil for the given requirements of this study.
Acknowledgements
Funding: This work was funded by the EU as part of the Seventh Framework Program (FP7) in the Health project 241719 “ADIPOA”.
Footnote
Conflicts of Interest: The authors have no conflicts of interest to declare.
References
- Bollet AJ, Nance JL. Biochemical Findings in Normal and Osteoarthritic Articular Cartilage. II. Chondroitin Sulfate Concentration and Chain Length, Water, and Ash Content. J Clin Invest 1966;45:1170-7. [PubMed]
- Rizkalla G, Reiner A, Bogoch E, Poole AR. Studies of the articular cartilage proteoglycan aggrecan in health and osteoarthritis. Evidence for molecular heterogeneity and extensive molecular changes in disease. J Clin Invest 1992;90:2268-77. [PubMed]
- Pearle AD, Warren RF, Rodeo SA. Basic science of articular cartilage and osteoarthritis. Clin Sports Med 2005;24:1-12. [PubMed]
- Bashir A, Gray ML, Burstein D. Gd-DTPA2- as a measure of cartilage degradation. Magn Reson Med 1996;36:665-73. [PubMed]
- Bashir A, Gray ML, Boutin RD, Burstein D. Glycosaminoglycan in articular cartilage: in vivo assessment with delayed Gd(DTPA)(2-)-enhanced MR imaging. Radiology 1997;205:551-8. [PubMed]
- Duvvuri U, Reddy R, Patel SD, Kaufman JH, Kneeland JB, Leigh JS. T1rho-relaxation in articular cartilage: effects of enzymatic degradation. Magn Reson Med 1997;38:863-7. [PubMed]
- Reddy R, Insko EK, Noyszewski EA, Dandora R, Kneeland JB, Leigh JS. Sodium MRI of human articular cartilage in vivo. Magn Reson Med 1998;39:697-701. [PubMed]
- Shapiro EM, Borthakur A, Gougoutas A, Reddy R. 23Na MRI accurately measures fixed charge density in articular cartilage. Magn Reson Med 2002;47:284-91. [PubMed]
- Wheaton AJ, Borthakur A, Shapiro EM, Regatte RR, Akella SV, Kneeland JB, Reddy R. Proteoglycan loss in human knee cartilage: quantitation with sodium MR imaging--feasibility study. Radiology 2004;231:900-5. [PubMed]
- Isaac G, Schnall MD, Lenkinski RE, Vogele K. A design for a double tuned birdcage coil for use in an integrated MRI/MRS examination. J Magn Reson 1990;89:41-50.
- Rath AR. Design and performance of a double-tuned bird-cage coil. J Magn Reson 1990;86:488-95.
- Matson GB, Vermathen P, Hill TC. A practical double-tuned 1H/31P quadrature birdcage headcoil optimized for 31P operation. Magn Reson Med 1999;42:173-82. [PubMed]
- Fitzsimmons JR, Beck BL, Brooker HR. Double resonant quadrature birdcage. Magn Reson Med 1993;30:107-14. [PubMed]
- Murphy-Boesch J, Srinivasan R, Carvajal L, Brown TR. Two configurations of the four-ring birdcage coil for 1H imaging and 1H-decoupled 31P spectroscopy of the human head. J Magn Reson B 1994;103:103-14. [PubMed]
- Amari S, Uluğ AM, Bornemann J, van Zijl PC, Barker PB. Multiple tuning of birdcage resonators. Magn Reson Med 1997;37:243-51. [PubMed]
- Wang C, Li Y, Wu B, Xu D, Nelson SJ, Vigneron DB, Zhang X. A practical multinuclear transceiver volume coil for in vivo MRI/MRS at 7 T. Magn Reson Imaging 2012;30:78-84. [PubMed]
- Duan Y, Peterson BS, Liu F, Brown TR, Ibrahim TS, Kangarlu A. Computational and experimental optimization of a double-tuned (1)H/(31)P four-ring birdcage head coil for MRS at 3T. J Magn Reson Imaging 2009;29:13-22. [PubMed]
- Hani AF, Kumar D, Malik AS, Razak R. Physiological assessment of in vivo human knee articular cartilage using sodium MR imaging at 1.5 T. Magn Reson Imaging 2013;31:1059-67. [PubMed]
- Shen GX, Boada FE, Thulborn KR. Dual-frequency, dual-quadrature, birdcage RF coil design with identical B1 pattern for sodium and proton imaging of the human brain at 1.5 T. Magn Reson Med 1997;38:717-25. [PubMed]
- Morrell GR. A phase-sensitive method of flip angle mapping. Magn Reson Med 2008;60:889-94. [PubMed]
- Allen SP, Morrell GR, Peterson B, Park D, Gold GE, Kaggie JD, Bangerter NK. Phase-sensitive sodium B1 mapping. Magn Reson Med 2011;65:1125-30. [PubMed]
- Sacolick LI, Sun L, Vogel MW, Dixon WT, Hancu I. Fast radiofrequency flip angle calibration by Bloch-Siegert shift. Magn Reson Med 2011;66:1333-8. [PubMed]
- Garrido Salmon CE, Géa Vidoto EL, Martins MJ, et al. Optimization of saddle coils for magnetic resonance imaging. Braz J Phys 2006;36:4-8.
- Hoult DI, Richards RE. The signal-to-noise ratio of the nuclear magnetic resonance experiment. 1976. J Magn Reson (1969) 1976;24:71-85.