Recent advances in surgical planning & navigation for tumor biopsy and resection
Introduction
Cancer is among the leading causes of morbidity and mortality worldwide, with approximately 14 million new cases and 8.2 million cancer related deaths in 2012 (1). Surgical operation is one of the cornerstones of curative treatment of cancer. However, majority of surgeries were only conducted when surgical planning showed a reasonable chance of complete removal of cancer tissues. As tumors could bury deep inside the brain or near crucial vessel or organ which is hard to define, precise surgical planning and navigation system for tumor biopsy and resection play an important role in surgical processes.
Current imaging techniques such as fluoroscopy, computed tomography (CT), and magnetic resonance imaging (MRI), are used for preoperative surgical planning and/or intraoperative surgical guidance. Regardless, single modality imaging has certain limitations in clearly identifying the actual tumor margin which is crucial for surgeons to make correct intra-procedural decisions (2). Intense efforts have therefore been given to the combination of multimodality imaging techniques, including functional imaging, for preoperative plan under a precise brain functional connectivity map. In addition, with the development of resting-state functional MRI, this non-task based functional imaging can be widely used in preoperative planning when patient is sleeping or unconsciousness (3-5). In addition to pre-surgical operation planning, the intra-surgical navigation technology also plays a crucial role in overcoming the limitations of human eye through intra-surgical visualization (6). The essence of all these pre-surgical and intra-surgical techniques are fused and results in the state-of-art Advanced Multimodal Image-Guided Operation (AMIGO) suite (7), which unites the multi-model imaging with operation room and guides the future development of imaging technology.
Moreover, three-dimensional (3D) printing and cloud computing technologies are fast growing areas of development in healthcare which worth further exploration of their usage in the surgical realm. Due to the fact that 3D-printing can turn a 3D medical image dataset into a 3D object in patient’s actual size, it can largely improve the precision of modern surgical planning by combining with other preoperative plan and intra-operative navigation techniques. In addition, it has been demonstrated that the utilization of cloud computing architecture can improve cost-efficiency, flexibility and clinical workflow (8,9).
To provide a better understanding of recent advanced technology in surgical planning and navigation, this review will focus on the innovative fields of multimodality imaging pre-operative planning, intra-operative imaging navigation and the application of 3D printing technique.
Multimodality imaging for precise surgical plan
Recently, increased attention has been given to the establishment of multimodality imaging techniques, especially functional imaging, for the application of precise preoperative plan. Functional magnetic resonance imaging (fMRI) and diffusion tensor imaging (DTI) can be used to determine the spatial relationships between tumor tissue and eloquent brain areas based on the structural MRI or CT image and this can be applied in the neurosurgery field for tumor resection planning (10). Mickleborough et al. (11) published a report concerning a patient with a large cavernous malformation, near the left basal ganglia, using pre-surgical planning fMRI localization, showing that both reading and attention-processing tasks revealed consistent activation of the left superior parietal lobule, part of the attentional control network, and the site of the planned surgical access. Surgical access was set to avoid interference with the attentional control network. In comparison with conventional MRI techniques which provide only anatomical information, DTI offers data on central nervous system (CNS) connectivity by enabling visualization of crucial white matter tracts in the brain and provide guidance on resection operation (12). Kleiser et al. (13) found that DTI results were more accurate than those using anatomical landmarks alone, in which fMRI results were used to localize start points for DTI tract reconstruction. Also, in previous studies of Romano et al. (14,15), they found that the utilization of DTI/fMRI in corticotomy led to a change of the surgical approach for resection in 16-21% of cases, furthermore, Rasmussen et al. found that this preoperative approach is more effective in all their cases examined (16). More recent studies by Cao et al. (17) found that in their retrospective analysis, preoperative planning using DTI enabled the surgeon to investigate individual patient specific surgical approach and can significantly improve the surgical outcome. Preoperative estimation of damage was shown to be possible using fMRI/DTI data in a recent study (18). Moreover, DTI tractography can provide important information to reduce the visual field defects by visualizing Meyer’s loop (19). This information is crucial to the epilepsy surgery team during pre-operative counseling.
Nevertheless, task-based fMRI which has traditionally been used to locate the exact key regions of the brain may not suitable for the patient who is unconsciousness or under anesthesia. That limits the application of the real-time surgical planning. Compared with this task-based method, resting state functional MRI (rsfMRI), which is a non-task based functional brain imaging technique, can be accustomed to evaluate the influence of brain tumor to brain function. Previous studies of predicting verbal fluency outcome following resective surgery for temporal lobe epilepsy had emphasized that the rsfMRI can detect the outcome following the lobectomy for the intractable temporal lobe epilepsy, and stated that this technique can be used effectively (20). In addition, this can be applied to locate specific brain resting state brain networks (RSNs) like sensorimotor regions using a seed-based approach for pre-operative planning (21). By comparing the pre- and post-tumor resection image it was shown that edema will affect the blood oxygenation level dependent (BOLD) signal response on ipsilateral side (22). The reliability and reproducibility of rsfMRI have been tested by previous studies, which suggest that RSNs can be detected reliably across imaging sessions (23,24) and across different subjects (24,25), though there may have some inter-subject variability (23).
Intraoperative imaging for surgery navigation
The aim of intra-operative imaging navigation is to develop high-precision interventions by improving current surgical and diagnostic techniques using intra-operative image guidance to facilitate successful lesion identification and resection. It is essential to establish a safe and optimal procedural workflow for combining image guidance with real-time surgery. It is also paramountly important to determine the pre-operative outcomes including successful lesion localization and to complete excision of the lesion. Traditional intraoperative surgery guidance systems are usually based on ultrasound, fluoroscopic imaging, structural MR, or CT imaging, which are basically single modality or bi-modality imaging intra-surgical navigation. However, these kinds of image-guided therapy (IGT) possess a number of limitations in functional imaging.
The concept of modern IGT, which dedicates not only to improve the localization and targeting of diseased tissue but to monitor and control the operation through imaging, has been featured in recent researches. Besides advanced medical instruments, it also requires a multidisciplinary team to assist the clinical procedure. This team requires a variety of expertise such as surgeons, radiologists, physicists, imaging specialists, biomedical engineers and computer scientists to work together and deliver a safe and precise surgical operation to patients (26). One of the most broadly used IGT type for intra-operation guidance is X-ray hybrid modalities. Housden et al. (27) presented a real-time imaging guidance system combined with trans-esophageal echo volume registered to and overlaid on an X-ray projection image in real-time by using X-ray fluoroscopy and 3D ultrasound imaging. The average alignment error is 2.9 mm tested in 11 clinical data sets. More advanced imaging-guided techniques have been present by other research teams. Patil et al. (28) developed a bedside external ventricular drain imaging-guided system. A 3D patient specific geometry model from CT imaging has been reconstructed in 3D Slicer. Meanwhile, electromagnetic (EM) tracking system recorded the position data and obtained registration with the 3D model in the Smart Stylet Computer.
The AMIGO suite at Brigham and Women’s Hospital is a state of art in modern IGT and designed to offer real-time intra-procedural multimodality imaging during surgical operation. This modern IGT suite system combined cross-sectional digital imaging like CT and 3.0T MRI, functional imaging like positron emission tomography (PET), and other real-time anatomical imaging like fluoroscopy and ultrasound together in a 3 sterile procedure rooms include an operating room (OR) (7). The intention of AMIGO is to provide a multimodality intra-procedural imaging during surgery for a precise definition of the tumor’s morphology and size to ensure the safety and accuracy of the operation of tumor biopsy, resection, or in situ destruction. Numbers of operations in different procedures have been done on this AMIGO suite, Golshan et al. (29) performed a pilot study on breast cancer surgery, and reported this advanced suite is feasible and able to reduce re-excision in therapy. Many more operations outcome have proven that there is a significant improvement in operation accuracy by using this modern IGT suite for neurosurgery and prostate cancer biopsy and resection (7,30-32).
Other imaging techniques have also been used in image guided therapy or intra-operative image-to-patient registration. Optical molecular imaging like fluorescence molecular imaging (FMI) has been used as a meticulous surgical guiding during the tumor resection (33-36). Unlike the IGT listed above, this FMI technique is using fluorescent dye to label the tumor tissue under an image-guided navigation system (37). Surgeons, traditionally determine the mass margin for biopsy or resection only by experience on pathological anatomy, nowadays can distinguish the malignant tissue by the previous fluorescent marker and perform a precise operation. This FMI optical imaging technology highly relies on the performance of the intra-operative imaging navigation system due to the fact that it is crucial to obtain an accurate depth information inside the patient’s body (37). Compare to the single-modal imaging systems, the multi-modal imaging guided system (such as intraoperative CT, MRI and/or 3D ultrasonography) can provide more precise solution for the fluorescence procedure. Some preclinical results using the near infrared imaging (NIR) technique unite with other imaging methods like ultrasonography (38), MRI (39) and X-ray CT (40) compensate for the depth issues and have already demonstrated the possibility of using the FMI guided multi-modality method to precisely excise tumors. Still, the intra-operative guided system supplying 3D precise detection of tumor margin is not yet commercial available and requires future development efforts.
Patient-to-image intraoperative registration is an important bridge which connects imaging navigation with patient. This is a critical step to give surgeons precise guidance information. The traditional methods for patient-to-image registration use marker based point-surface matching registration method (41-43). The limitation of this method is that it can only be used to registration a limited area near the point cloud center (44). One recent study by Fan et al. (45) uses portable 3D scanner to acquire a markerless patient-to-image registration. This lightweight, portable 3D scanner based intra-operative registration technique greatly facilitates the preoperative registration procedure. To unify this registration technique into modern IGT platform will be a promising future.
3D-printing technology for patient specific surgical plan
Recently the technology of 3D-printing is spreading fast not only in the industry and business area, but also in clinical application. Due to this technology can turn a digital 3D model into a 3D replicated object; it has the capabilities to largely improve the precision of modern surgical plan by combining with other preoperative plan and intraoperative navigation techniques. The basic workflow of 3D printing is showed in Figure 1, indicating that this advanced technology has broad applications not only in clinical field, but also in biomedical engineering field.
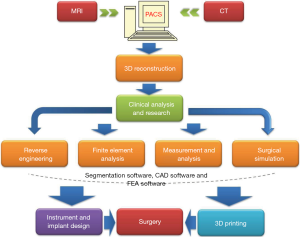
Careful pre-operative planning
For the resection of tumor with an intricate anatomical pathology such as like pelvic bone tumor, it is indispensable to perform a preoperative pathological anatomy analysis and rehearsal before the real operation (46). The patient 3D-printed replica can provide surgeons opportunities to optimize the approach during pre-operation meetings (47). Silberstein et al. (48) printed a patient specific 3D renal unit model with enhancing renal lesion identified on imaging. This 3D printed model solves the disadvantage of 3D imaging which is limited in depth appreciation displayed on 2D screen.
Accurate intra-operative execution
As the 3D printing technology can produce a patient specific anatomical model, it also can be used to design a personalized surgical implant jig or prosthesis by using computer aided design (CAD) or Solidworks. It’s a “bridge” to link the preoperative plan to the intraoperative execution and quantify any corrections. Qiao et al. (49) combined 3D printing with a computer-assisted reduction technique to design a patient specific external fixator of treating tibial fractures and limb function recovery. The results of reduction were obtained from three tibial fractures with an average lateral displacement of 2.04 mm (±1.53 mm) and an angulation of 2.54° (±1.33°). Kusaka et al. (50) also reported that they adopted an inkjet 3D printer to produce a 3D organ model for the surgical planning and intra-operative guidance.
Cloud computing for fast surgical planning and navigation
As reviewed in the previous sections of this article, the advancement of surgical planning and navigation techniques comes with higher requirements on technical support, equipment and computational power. Cutting edge surgical techniques are therefore restricted to institutions with sophisticate equipment and specific technical supports. Previous studies of graphics processing unit (GPU)-based medical image computing techniques had emphasized that with the rapid development of GPU, the parallel GPU computation technique will greatly enhance the medical imaging processing performance (51). However, this parallel acceleration computation may largely rely on the hardware performance of the computing machine. Such limitations are solvable through employing cloud technologies. It is crucial to analyses the feasibility to migrate surgical planning and navigation techniques from traditional platforms to clouds with a cost-efficient and user-friendly yet secure fashion.
Comparing to other medical fields, cloud computing is relatively new in the field of surgical planning and navigation, only limited previous literatures are available related to the topic. The majority of them were pioneer studies without actual implementation discussing whether cloud-computing is advantageous in clinical uses (52,53). Two of the recent studies related to cloud surgical planning have been reviewed which contain relevant reference value to any computational-power-dependent surgery techniques.
Cloud-based preoperative digital templating
Maratt and colleagues performed a research against the accuracy, efficiency and compliance of cloud-based surgery planning in 2012 (54). They showed that preoperative planning for total hip arthroplasty on cloud-based software produce comparable result in terms of accuracy with traditional acetate overlay templating with more than 2-fold efficiency. They further stated that cloud-based digital templating provide additional benefits of cost saving, efficiency and workflow improvement for total hip arthroplasty (54). In their specific case of total hip arthroplasty, digital surgical planning was a newly available, cloud-based system particularly for this purpose during the time. Knowing that, Maratt et al. stressed in their study that for other medical applications or research, regulatory changes must be made before the advantages of cloud technologies can be realized.
Cloud-based surgical planning architecture
Similar effort had been made by Schoenhagen, Zimmermann and Falkner who reported the application of cloud computing in clinical workflows of trans-catheter aortic valve replacement treatment in 2013 (8). They are motivated to deploy such system due to trans-catheter aortic valve planning require intensive 3D modeling and produce large volume of image data which results in limited sharing ability. This leads to the necessity to have a “reading room” that has more delicate workstations to handle the computation, not restricted to trans-catheter aortic valve, in majority of hospitals or clinics for clinicians to review the images. In attempts to resolve this counter-productive system, Schoenhagen et al. adopt a cloud architecture where processing work and data storing are centralized to powerful cloud server. The resultant image data can be accessed by multiple less expensive computer clients. Their model, however, was limited by network speed because of intense traffic between the clients and the central cloud server generated from image exchanges between clients and database (8).
The above discussed cases demonstrated how cloud architecture can assist in preoperative planning through centralizing computational steps to cloud servers. With increasingly computational-intense planning methodology being proposed and implemented, the importance of cloud computing will gradually emerge. It is believed that exploration in the direction of preoperative application of cloud computation can accelerate the process of surgical planning in individual hospitals.
Conclusions and perspectives
With the help of the development of multimodality imaging, especially the fusion of functional imaging in pre-operative planning and modern image guided therapy in intra-operative navigating, surgeons are now able to operate a portion of extremely risky procedures with both high level of safety and accuracy. However, the current use of multimodal MRI imagining for tumor surgical planning and navigation is largely restricted to a few institutions with strong technical support from physicists and imaging and image post processing specialists to maintain this entire preoperative planning system. As the development of medical imaging technology advanced, the increasing number of imaging processing systems and intra-operative imaging guided platforms became powerful but also complicated. Surgeons tend to like a reliable, stable, and user-friendly platform. Unfortunately, due to the fact that the commercial imaging platforms are closed source, standardization of surgical procedures can hardly be realized by surgeons. The advancement of automatic, robust and easy-to-use multimodal MRI/CT analysis and fusion tool for surgeons to perform the pre-operative plan and intra-operative navigation is highly desirable.
Given that surgical planning and navigation of tumor biopsy and resection are highly dependent on digital imaging and registration, adopting cloud architecture can improve clinical workflow. Nevertheless, it is stressed that the aforesaid advantages are only the most obvious benefits of cloud computing, previous researchers focused mainly on hardware cost-efficiency improvements and yet to further explore other potential benefits brought by cloud technologies.
More importantly, cloud-computing can enable better collaboration among people because its usage is not restricted by the physical locations of the users and the server. For example, a cloud surgical planning application can be implemented such that multiple surgeons can work on the same case simultaneously and cross reference each other’s work and perfect the plan. Experienced individuals from all over the world can be invited to be involved in the planning and enable other surgeons to learn from their invaluable experience such as accurate identification of tumor mass region. Under suitable circumstances, surgeons can also allow the patients to receive updates of their surgical planning without much additional effort and hence, allowing them to gain better understanding of the risks and be able to make relevant decisions regarding the surgery.
Nonetheless, it is also necessary to recognize the limitation of cloud computation before deciding whether it is suitable for the intended applications. For any cloud-base model in the medical field, the most challenges lies in security and privacy issues. Since researcher must pass the data to cloud server hosts in order to utilize the cloud, if such host is not within trusty domains, it posts a security threat to all the data being computed or processed on the server and, thus, violate privacy regulations. It is, however, quite expensive and time-consuming to host a private cloud for projects of smaller scale (55). Fortunately, we can expect this issue to be resolved in the near future with the maturation of fully homomorphic encryption schemes (9,56), which allows one to compute encrypted data directly and produce correct encrypted results without knowing the true content of the data itself, preventing third parties from accessing patients’ or subjects’ personal information yet allow research efforts to carry on.
Acknowledgements
The authors would like to acknowledge the insightful discussions with the members in surgical planning laboratory at Brigham and Women Hospital during the summer visit in 2015.
Funding: This study was partially supported by Knowledge Transfer Fund at CUHK (Project Code: TBF14MED008).
Footnote
Conflicts of Interest: The authors have no conflicts of interest to declare.
References
- Stewart BW, Wild CP, editors. World Cancer Report 2014. Geneva: WHO Press, 2014.
- Gering DT, Nabavi A, Kikinis R, Grimson WE, Hata N, Everett P, Jolesz F, Wells WM. An Integrated Visualization System for Surgical Planning and Guidance Using Image Fusion and Interventional Imaging. In: Taylor C, Colchester A, editors. Medical Image Computing and Computer-Assisted Intervention – MICCAI’99. Berlin: Springer Berlin Heidelberg, 1999:809-19.
- Liu H, Buckner RL, Talukdar T, Tanaka N, Madsen JR, Stufflebeam SM. Task-free presurgical mapping using functional magnetic resonance imaging intrinsic activity. J Neurosurg 2009;111:746-54. [PubMed]
- Kokkonen SM, Nikkinen J, Remes J, Kantola J, Starck T, Haapea M, Tuominen J, Tervonen O, Kiviniemi V. Preoperative localization of the sensorimotor area using independent component analysis of resting-state fMRI. Magn Reson Imaging 2009;27:733-40. [PubMed]
- Shimony JS, Zhang D, Johnston JM, Fox MD, Roy A, Leuthardt EC. Resting-state spontaneous fluctuations in brain activity: a new paradigm for presurgical planning using fMRI. Acad Radiol 2009;16:578-83. [PubMed]
- Wang D, Tewfik AH. Real time 3D visualization of intraoperative organ deformations using structured dictionary. IEEE Trans Med Imaging 2012;31:924-37. [PubMed]
- Tempany CM, Jayender J, Kapur T, Bueno R, Golby A, Agar N, Jolesz FA. Multimodal imaging for improved diagnosis and treatment of cancers. Cancer 2015;121:817-27. [PubMed]
- Schoenhagen P, Zimmermann M, Falkner J. Advanced 3-D analysis, client-server systems, and cloud computing-Integration of cardiovascular imaging data into clinical workflows of transcatheter aortic valve replacement. Cardiovasc Diagn Ther 2013;3:80-92. [PubMed]
- Gentry CB, inventor; International Business Machines Corporation, assignee. Fully homomorphic encryption method based on a bootstrappable encryption scheme, computer program and apparatus. United States patent US 20110110525 A1. 2011 May 12.
- Dimou S, Battisti RA, Hermens DF, Lagopoulos J. A systematic review of functional magnetic resonance imaging and diffusion tensor imaging modalities used in presurgical planning of brain tumour resection. Neurosurg Rev 2013;36:205-14; discussion 214. [PubMed]
- Mickleborough MJ, Kelly ME, Gould L, Ekstrand C, Lorentz E, Ellchuk T, Babyn P, Borowsky R. Inclusion of attentional networks in the pre-surgical neuroimaging assessment of a large deep hemispheric cavernous malformation: an FMRI case report. Cerebrovasc Dis 2015;39:202-8. [PubMed]
- Abdullah KG, Lubelski D, Nucifora PG, Brem S. Use of diffusion tensor imaging in glioma resection. Neurosurg Focus 2013;34:E1. [PubMed]
- Kleiser R, Staempfli P, Valavanis A, Boesiger P, Kollias S. Impact of fMRI-guided advanced DTI fiber tracking techniques on their clinical applications in patients with brain tumors. Neuroradiology 2010;52:37-46. [PubMed]
- Romano A, D’Andrea G, Minniti G, Mastronardi L, Ferrante L, Fantozzi LM, Bozzao A. Pre-surgical planning and MR-tractography utility in brain tumour resection. Eur Radiol 2009;19:2798-808. [PubMed]
- Romano A, Ferrante M, Cipriani V, Fasoli F, Ferrante L, D’Andrea G, Fantozzi LM, Bozzao A. Role of magnetic resonance tractography in the preoperative planning and intraoperative assessment of patients with intra-axial brain tumours. Radiol Med 2007;112:906-920. [PubMed]
- Rasmussen IA Jr, Lindseth F, Rygh OM, Berntsen EM, Selbekk T, Xu J, Nagelhus Hernes TA, Harg E, Håberg A, Unsgaard G. Functional neuronavigation combined with intra-operative 3D ultrasound: initial experiences during surgical resections close to eloquent brain areas and future directions in automatic brain shift compensation of preoperative data. Acta Neurochir (Wien) 2007;149:365-78. [PubMed]
- Cao Z, Lv J, Wei X, Quan W. Appliance of preoperative diffusion tensor imaging and fiber tractography in patients with brainstem lesions. Neurol India 2010;58:886-90. [PubMed]
- Fernandez-Miranda JC, Engh JA, Pathak SK, Madhok R, Boada FE, Schneider W, Kassam AB. High-definition fiber tracking guidance for intraparenchymal endoscopic port surgery. J Neurosurg 2010;113:990-9. [PubMed]
- Lilja Y, Nilsson DT. Strengths and limitations of tractography methods to identify the optic radiation for epilepsy surgery. Quant Imaging Med Surg 2015;5:288-99. [PubMed]
- Osipowicz K, Sperling MR, Sharan AD, Tracy JI. Functional MRI, resting state fMRI, and DTI for predicting verbal fluency outcome following resective surgery for temporal lobe epilepsy. J Neurosurg 2015.1-9. [Epub ahead of print]. [PubMed]
- Zhang D, Johnston JM, Fox MD, Leuthardt EC, Grubb RL, Chicoine MR, Smyth MD, Snyder AZ, Raichle ME, Shimony JS. Preoperative sensorimotor mapping in brain tumor patients using spontaneous fluctuations in neuronal activity imaged with functional magnetic resonance imaging: initial experience. Neurosurgery 2009;65:226-36. [PubMed]
- Kokkonen SM, Kiviniemi V, Mäkiranta M, Yrjänä S, Koivukangas J, Tervonen O. Effect of brain surgery on auditory and motor cortex activation: a preliminary functional magnetic resonance imaging study. Neurosurgery 2005;57:249-56; discussion 249-56. [PubMed]
- Biswal BB, Mennes M, Zuo XN, Gohel S, Kelly C, Smith SM, Beckmann CF, Adelstein JS, Buckner RL, Colcombe S, Dogonowski AM, Ernst M, Fair D, Hampson M, Hoptman MJ, Hyde JS, Kiviniemi VJ, Kötter R, Li SJ, Lin CP, Lowe MJ, Mackay C, Madden DJ, Madsen KH, Margulies DS, Mayberg HS, McMahon K, Monk CS, Mostofsky SH, Nagel BJ, Pekar JJ, Peltier SJ, Petersen SE, Riedl V, Rombouts SA, Rypma B, Schlaggar BL, Schmidt S, Seidler RD, Siegle GJ, Sorg C, Teng GJ, Veijola J, Villringer A, Walter M, Wang L, Weng XC, Whitfield-Gabrieli S, Williamson P, Windischberger C, Zang YF, Zhang HY, Castellanos FX, Milham MP. Toward discovery science of human brain function. Proc Natl Acad Sci U S A 2010;107:4734-9. [PubMed]
- Shehzad Z, Kelly AM, Reiss PT, Gee DG, Gotimer K, Uddin LQ, Lee SH, Margulies DS, Roy AK, Biswal BB, Petkova E, Castellanos FX, Milham MP. The resting brain: unconstrained yet reliable. Cereb Cortex 2009;19:2209-29. [PubMed]
- Damoiseaux JS, Rombouts SA, Barkhof F, Scheltens P, Stam CJ, Smith SM, Beckmann CF. Consistent resting-state networks across healthy subjects. Proc Natl Acad Sci U S A 2006;103:13848-53. [PubMed]
- Jolesz FA. History of Image-Guided Therapy at Brigham and Women’s Hospital. In: Jolesz FA, editor. Intraoperative Imaging and Image-Guided Therapy. New York: Springer-Verlag New York, 2014:25-45.
- Housden RJ, Arujuna A, Ma Y, Nijhof N, Gijsbers G, Bullens R, O’Neill M, Cooklin M, Rinaldi CA, Gill J, Kapetanakis S, Hancock J, Thomas M, Razavi R, Rhode KS. Evaluation of a real-time hybrid three-dimensional echo and X-ray imaging system for guidance of cardiac catheterisation procedures. Med Image Comput Comput Assist Interv 2012;15:25-32. [PubMed]
- Patil V, Gupta R, San José Estépar R, Lacson R, Cheung A, Wong JM, Popp AJ, Golby A, Ogilvy C, Vosburgh KG. Smart stylet: the development and use of a bedside external ventricular drain image-guidance system. Stereotact Funct Neurosurg 2015;93:50-8. [PubMed]
- Golshan M, Sagara Y, Wexelman B, Aydogan F, Desantis S, Elise Min H, Vosburgh K, Jagadeesan J, Caragacianu D, Gombos E, Jolesz FA. Pilot study to evaluate feasibility of image-guided breast-conserving therapy in the advanced multimodal image-guided operating (AMIGO) suite. Ann Surg Oncol 2014;21:3356-7. [PubMed]
- Agar NY, Golby AJ, Ligon KL, Norton I, Mohan V, Wiseman JM, Tannenbaum A, Jolesz FA. Development of stereotactic mass spectrometry for brain tumor surgery. Neurosurgery 2011;68:280-89; discussion 290. [PubMed]
- Stamatakis L, Siddiqui MM, Nix JW, Logan J, Rais-Bahrami S, Walton-Diaz A, Hoang AN, Vourganti S, Truong H, Shuch B, Parnes HL, Turkbey B, Choyke PL, Wood BJ, Simon RM, Pinto PA. Accuracy of multiparametric magnetic resonance imaging in confirming eligibility for active surveillance for men with prostate cancer. Cancer 2013;119:3359-66. [PubMed]
- Abd-Alazeez M, Ahmed HU, Arya M, Charman SC, Anastasiadis E, Freeman A, Emberton M, Kirkham A. The accuracy of multiparametric MRI in men with negative biopsy and elevated PSA level--can it rule out clinically significant prostate cancer? Urol Oncol 2014;32:45.e17-22.
- Nguyen QT, Tsien RY. Fluorescence-guided surgery with live molecular navigation--a new cutting edge. Nat Rev Cancer 2013;13:653-62. [PubMed]
- van Dam GM, Themelis G, Crane LM, Harlaar NJ, Pleijhuis RG, Kelder W, Sarantopoulos A, de Jong JS, Arts HJ, van der Zee AG, Bart J, Low PS, Ntziachristos V. Intraoperative tumor-specific fluorescence imaging in ovarian cancer by folate receptor-α targeting: first in-human results. Nat Med 2011;17:1315-9. [PubMed]
- van der Vorst JR, Schaafsma BE, Hutteman M, Verbeek FP, Liefers GJ, Hartgrink HH, Smit VT, Löwik CW, van de Velde CJ, Frangioni JV, Vahrmeijer AL. Near-infrared fluorescence-guided resection of colorectal liver metastases. Cancer 2013;119:3411-8. [PubMed]
- Sugie T, Sawada T, Tagaya N, Kinoshita T, Yamagami K, Suwa H, Ikeda T, Yoshimura K, Niimi M, Shimizu A, Toi M. Comparison of the indocyanine green fluorescence and blue dye methods in detection of sentinel lymph nodes in early-stage breast cancer. Ann Surg Oncol 2013;20:2213-8. [PubMed]
- Chi C, Du Y, Ye J, Kou D, Qiu J, Wang J, Tian J, Chen X. Intraoperative imaging-guided cancer surgery: from current fluorescence molecular imaging methods to future multi-modality imaging technology. Theranostics 2014;4:1072-84. [PubMed]
- Peloso A, Franchi E, Canepa MC, Barbieri L, Briani L, Ferrario J, Bianco C, Quaretti P, Brugnatelli S, Dionigi P, Maestri M. Combined use of intraoperative ultrasound and indocyanine green fluorescence imaging to detect liver metastases from colorectal cancer. HPB (Oxford) 2013;15:928-34. [PubMed]
- Chen YJ, Wu SC, Chen CY, Tzou SC, Cheng TL, Huang YF, Yuan SS, Wang YM. Peptide-based MRI contrast agent and near-infrared fluorescent probe for intratumoral legumain detection. Biomaterials 2014;35:304-15. [PubMed]
- Luo T, Huang P, Gao G, Shen G, Fu S, Cui D, Zhou C, Ren Q. Mesoporous silica-coated gold nanorods with embedded indocyanine green for dual mode X-ray CT and NIR fluorescence imaging. Opt Express 2011;19:17030-9. [PubMed]
- Peters TM. Image-guidance for surgical procedures. Phys Med Biol 2006;51:R505-40. [PubMed]
- Siessegger M, Mischkowski RA, Schneider BT, Krug B, Klesper B, Zöller JE. Image guided surgical navigation for removal of foreign bodies in the head and neck. J Craniomaxillofac Surg 2001;29:321-5. [PubMed]
- Wang M, Song Z. Improving target registration accuracy in image-guided neurosurgery by optimizing the distribution of fiducial points. Int J Med Robot 2009;5:26-31. [PubMed]
- Wang MN, Song ZJ. Properties of the target registration error for surface matching in neuronavigation. Comput Aided Surg 2011;16:161-9. [PubMed]
- Fan Y, Jiang D, Wang M, Song Z. A new markerless patient-to-image registration method using a portable 3D scanner. Med Phys 2014;41:101910. [PubMed]
- Wong KC, Kumta SM, Geel NV, Demol J. One-step reconstruction with a 3D-printed, biomechanically evaluated custom implant after complex pelvic tumor resection. Comput Aided Surg 2015;20:14-23. [PubMed]
- Salloum C, Lim C, Fuentes L, Osseis M, Luciani A, Azoulay D. Fusion of Information from 3D Printing and Surgical Robot: An Innovative Minimally Technique Illustrated by the Resection of a Large Celiac Trunk Aneurysm. World J Surg 2015. [Epub ahead of print]. [PubMed]
- Silberstein JL, Maddox MM, Dorsey P, Feibus A, Thomas R, Lee BR. Physical models of renal malignancies using standard cross-sectional imaging and 3-dimensional printers: a pilot study. Urology 2014;84:268-72. [PubMed]
- Qiao F, Li D, Jin Z, Hao D, Liao Y, Gong S. A novel combination of computer-assisted reduction technique and three dimensional printed patient-specific external fixator for treatment of tibial fractures. Int Orthop 2015. [Epub ahead of print]. [PubMed]
- Kusaka M, Sugimoto M, Fukami N, Sasaki H, Takenaka M, Anraku T, Ito T, Kenmochi T, Shiroki R, Hoshinaga K. Initial experience with a tailor-made simulation and navigation program using a 3-D printer model of kidney transplantation surgery. Transplant Proc 2015;47:596-9. [PubMed]
- Shi L, Liu W, Zhang H, Xie Y, Wang D. A survey of GPU-based medical image computing techniques. Quant Imaging Med Surg 2012;2:188-206. [PubMed]
- Kagadis GC, Kloukinas C, Moore K, Philbin J, Papadimitroulas P, Alexakos C, Nagy PG, Visvikis D, Hendee WR. Cloud computing in medical imaging. Med Phys 2013;40:070901. [PubMed]
- Ohmann C, Canham S, Danielyan E, Robertshaw S, Legré Y, Clivio L, Demotes J. ’Cloud computing’ and clinical trials: report from an ECRIN workshop. Trials 2015;16:318. [PubMed]
- Maratt JD, Srinivasan RC, Dahl WJ, Schilling PL, Urquhart AG. Cloud-based preoperative planning for total hip arthroplasty: a study of accuracy, efficiency, and compliance. Orthopedics 2012;35:682-6. [PubMed]
- Yelick K, Coghlan S, Draney B, Canon RS. The Magellan Report on Cloud Computing for Science. U.S. Department of Energy, Office of Advanced Scientific Computing Research (ASCR), December, 2011. Available online: http://science.energy.gov/~/media/ascr/pdf/program-documents/docs/Magellan_Final_Report.pdf
- Brakerski Z, Gentry C, Vaikuntanathan V. Fully Homomorphic Encryption without Bootstrapping. ITCS '12 Proceedings of the 3rd Innovations in Theoretical Computer Science Conference. ACM New York, NY, USA, 2012:309-25.