Peak flow velocities in the ascending aorta—real-time phase-contrast magnetic resonance imaging vs. cine magnetic resonance imaging and echocardiography
Introduction
Echocardiography plays an important role in routine diagnostics of valvular heart disease (1). Its advantages are widespread availability, lack of radiation and multi-local performance. On the other hand, limitations are due to the need of a suitable acoustic window and the dependence on observer experience. Moreover, in contrast to imaging modalities such as computed tomography and magnetic resonance imaging (MRI), echocardiography does not allow for the evaluation of widespread surrounding anatomic structures.
For more than a decade ECG-synchronized cine acquisitions of phase-contrast MRI sequences with selective encoding of through-plane flow are increasingly used for the assessment of cardiac outflow (2-5). In particular, cine phase-contrast MRI of cardiac flow has become the “gold standard” for functional evaluations of the left and right ventricle (6) and an ideal tool for the diagnosis of valvular diseases like stenosis, insufficiency or shunts (7).
Recently, technical advances in real-time MRI (8) have been applied to cardiac MRI (9,10) and further extended to quantitative phase-contrast MRI of through-plane flow (11,12). The real-time MRI technique applied here emerges as a promising new modality for measuring cardiovascular function and flow with high temporal and spatial resolution (13). It extends a variety of previous trials based on different combinations of echo-planar or spiral encoding strategies and parallel imaging techniques (14-20), which are restricted by limited spatiotemporal resolution and/or low image quality that so far precluded clinical acceptance.
The purpose of this pilot study under normal physiologic conditions was to assess the quality of real-time blood flow measurements (12) in the ascending aorta of healthy young volunteers and to compare the results to cine phase-contrast MRI and echocardiography.
Materials and methods
Subjects
Aortic blood flow of eight male healthy volunteers (24.9±2.0 years, range 22-28 years) was measured by MRI and echocardiography in a prospective single-center study. The study was approved by the institutional review board and written informed consent was obtained before MRI and echocardiography. Although one subject had a history of diabetes mellitus type 1, see Table 1, there were no contraindications for MRI or echocardiography for all volunteers. This study was in consent with the Declaration of Helsinki.
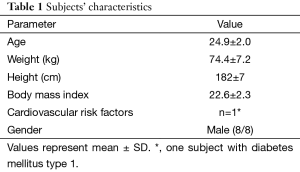
Full table
Magnetic resonance imaging (MRI)
MRI examinations were performed at 3T (Tim Trio, Siemens Healthcare, Erlangen, Germany) with use of a 32-element cardiac coil. MRI-compatible ECG recordings were used for cine phase-contrast MRI. For real-time MRI the ECG was co-registered and time stamps relative to the last R wave were assigned to respective images.
Real-time MRI was based on a highly undersampled radial gradient-echo sequence with image reconstruction by regularized nonlinear inversion (NLINV) (8). Modifications of the original method for anatomic MRI which are required for real-time phase-contrast flow MRI (11,12) refer to the use of phase-sensitive reconstructions of two series of differently flow-encoded images and the calculation of velocity-encoded phase-contrast maps without any temporal filter. Under these conditions, the temporal fidelity of the iteratively estimated images was experimentally validated with the use of a specially designed motion phantom covering a range of velocities and image acquisition times (21). Excellent spatiotemporal acuity even of small and fast moving objects was obtained for NLINV reconstructions without post-processing temporal filter.
Through-plane flow encoding was accomplished in a sequential manner using a bipolar flow-encoding gradient in every other image acquisition. The radial fast low-angle shot (FLASH) images were obtained from only seven spokes (base resolution 144) yielding a total acquisition time of 40 ms for one pair of images with and without flow-encoding gradient. T1-weighted images were acquired with repetition time (TR) 2.86 ms, echo time (TE) 1.93 ms, flip angle 10°, field of view 192×192 mm2, nominal in-plane resolution 1.3×1.3 mm2, slice thickness 6 mm, and VENC 200 cm s−1.
Magnitude images and phase-contrast maps were reconstructed online with the use of a fully integrated “bypass” computer to the host of the MRI system (sysGen/TYAN Octuple-GPU, 2x Intel Westmere E5620 processor, 48GB RAM, Sysgen, Bremen, Germany) equipped with 2×4 graphical processing units (GPUs, GeForce GTX 580, NVIDIA, Santa Clara, California, USA). Real-time phase-contrast MRI data were acquired during free breathing for 15 s corresponding to about 12 to 15 heartbeats.
Cine phase-contrast MRI with conventional Cartesian phase- and frequency-encoding was performed during breath-holding with the following parameters: TR 46.90 ms, TE 1.92 ms, flip angle 25°, rectangular FOV of 320×220 mm2, 1 average, 20 phases, 5 segments, in-plane resolution 1.7 mm, slice thickness 6 mm, and VENC 200 cm s−1. GRAPPA was applied with an acceleration factor of 2 and 24 central auto-calibration lines. The measuring time for cine MRI with breath-holding was 13 s.
MRI flow analyses
Peak flow velocities (PFV) were determined with a commercially available software package QFlow (Medis, Leiden, The Netherlands). For the analysis of real-time image series a prototype version of QFlow supports a separate treatment of data from multiple heartbeats. Real-time evaluations were based on 30 cardiac cycles (three scans each providing ten consecutive cardiac cycles) using both magnitude images and phase-contrast maps. As shown in Figure 1 segmentation of the aorta was accomplished by a semi-automatic definition of contours by two observers with 3 and 2 years of cardiac MRI experience (A.A.J. and J.T.K.). If necessary, contours were manually corrected and ambiguities were resolved by consensus.
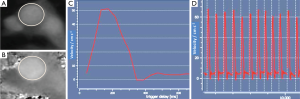
Echocardiography
Echocardiography was performed by one cardiologist with 28 years of experience (C.U.B.) using a clinically established ultrasound system (iE33, Philips, The Netherlands) with trans-thoracic CW (Continuous-Wave) Doppler technique. The frequency was about 1.8 MHz, and 2D images were used for general anatomic orientation and localization of the correct measurement. In 7 of 8 volunteers echocardiography involved an apical five-chamber view, while one subject required a suprasternal view with focus on the aortic valve for flow measurement. CW-Doppler velocities were analyzed offline using 2D Cardiac Performance Analysis (TomTec Imaging System, Munich, Germany). Three measurements with three repetitions each resulted in mean values for a total of nine heart cycles.
Statistics
Real-time flow MRI measurements were correlated with the results of cine MRI and echocardiography using Bland-Altman plots (22,23).
Results and discussion
In this study of flow in the ascending aorta of healthy young adults, real-time phase-contrast MRI at 40 ms temporal resolution resulted in PFV that quantitatively agree with values obtained by echocardiography and cine MRI. The PFV for real-time and cine phase-contrast MRI as well as echocardiography are summarized in Table 2. The respective mean values are virtually identical for the group of healthy young adults studied here. A visual representation of this finding is demonstrated in Figure 2.
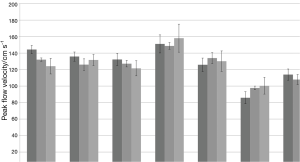
On an individual basis, deviations between real-time phase-contrast MRI and echocardiography varied between –20 and +14 cm s−1 which corresponds to –14 to +16%. Respective correlations and Bland-Altman plots are depicted in Figure 3. However, such individual deviations are only slightly larger than for cine phase-contrast MRI (–12 to +12 cm s−1 corresponding to –8 to +14%) and in most cases show the same positive or negative trend as demonstrated in Table 2 and Figure 3. These observations again pose methodologic questions that are difficult to answer. This is mainly because of the absence of a true gold standard for in vivo flow (i.e., velocity) measurements as most techniques have their specific drawbacks and pitfalls. For example, the need for a proper acoustic window in echocardiography may lower the quality of examinations to such a degree that no reliable quantitative values are obtainable. With respect to MRI, ECG-synchronized cine acquisitions depend on a robust periodicity of cardiac cycles. As a consequence, contributions from irregular heartbeats are likely to cause phase differences which upon averaging during retrospective synthesis of a single-cycle representation would affect the velocity-encoded phase information. Similar problems may occur when instead of a breath hold acquisition the retrospective sorting and averaging of data extends over many more heartbeats for a free breathing cine phase-contrast method.
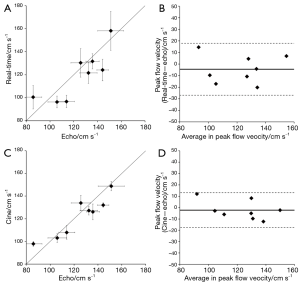
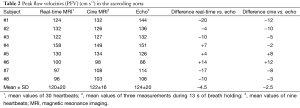
Full table
In addition to the aforementioned problems, comparisons between MRI and ultrasound techniques are further complicated by other differences such as, for example, the common choice of a position for echocardiography close to the aortic valve, whereas MRI studies are typically performed in a slightly more ventral section at the level of the pulmonary artery. On the other hand, any situation that enhances the degree of or exposure to turbulent flow would affect all phase-dependent MRI techniques by a partial loss of phase coherence which in turn translates into lower velocities. This unfortunate situation also applies to many literature studies which deal with methodologic comparisons for specific groups of patients or pathologies. It therefore remains to be seen whether real-time phase-contrast MRI still behaves similar to echocardiography when, for example, studying blood flow in patients with aortic valve stenosis.
Conclusions
Real-time phase-contrast MRI of through-plane flow in the ascending aorta resulted in a quantitative agreement with both conventional cine phase-contrast MRI and echocardiography. While real-time PFV in a group of healthy young adults were lower to echocardiography by only about 3% when averaged across subjects, maximum differences in individual subjects were up to ±15%. The present work demonstrates that real-time phase-contrast MRI promises robust measurements of cardiac outflow even in situations where echocardiography suffers from an improper acoustic window or ECG-synchronized cine MRI is compromised by irregular heartbeats. In fact, real-time phase-contrast MRI offers access to flow in individual cardiac cycles and therefore may be applied to monitor the immediate physiologic responses to stress or exercise. Extended patient studies are now warranted to investigate its performance for different vascular or structural pathologies, in particular for situations with increased turbulent flow.
Acknowledgements
Funding: This work was supported by the DFG, project number LO 1773/1-1. We thank Medis, Leiden, The Netherlands, for providing a prototype version of QFlow software which allows for the analysis of multiple consecutive heartbeats.
Footnote
Conflicts of Interest: Jens Frahm holds a patent about the real-time MRI acquisition and reconstruction technique used here. All other authors declare that they have no competing interest.
References
- Gillebert TC, De Pauw M, Timmermans F. Echo-Doppler assessment of diastole: flow, function and haemodynamics. Heart 2013;99:55-64. [PubMed]
- Kondo C, Caputo GR, Semelka R, Foster E, Shimakawa A, Higgins CB. Right and left ventricular stroke volume measurements with velocity-encoded cine MR imaging: in vitro and in vivo validation. AJR Am J Roentgenol 1991;157:9-16. [PubMed]
- Hoskins PR. Accuracy of maximum velocity estimates made using Doppler ultrasound systems. Br J Radiol 1996;69:172-7. [PubMed]
- Lee VS, Spritzer CE, Carroll BA, Pool LG, Bernstein MA, Heinle SK, MacFall JR. Flow quantification using fast cine phase-contrast MR imaging, conventional cine phase-contrast MR imaging, and Doppler sonography: in vitro and in vivo validation. AJR Am J Roentgenol 1997;169:1125-31. [PubMed]
- Lotz J, Meier C, Leppert A, Galanski M. Cardiovascular flow measurement with phase-contrast MR imaging: basic facts and implementation. Radiographics 2002;22:651-71. [PubMed]
- Lotz J, Kivelitz D, Fischbach R, Beer M, Miller S. Recommendations for utilizing computerized tomography and magnetic resonance tomography in heart diagnosis. 2--Magnetic resonance tomography. Rofo 2009;181:800-14. [PubMed]
- Lotz J. Flow measurements in cardiac MRI. Radiologe 2007;47:333-41. [PubMed]
- Uecker M, Zhang S, Voit D, Karaus A, Merboldt KD, Frahm J. Real-time MRI at a resolution of 20 ms. NMR Biomed 2010;23:986-94. [PubMed]
- Zhang S, Uecker M, Voit D, Merboldt KD, Frahm J. Real-time cardiovascular magnetic resonance at high temporal resolution: radial FLASH with nonlinear inverse reconstruction. J Cardiovasc Magn Reson 2010;12:39. [PubMed]
- Voit D, Zhang S, Unterberg-Buchwald C, Sohns JM, Lotz J, Frahm J. Real-time cardiovascular magnetic resonance at 1.5 T using balanced SSFP and 40 ms resolution. J Cardiovasc Magn Reson 2013;15:79. [PubMed]
- Joseph AA, Merboldt KD, Voit D, Zhang S, Uecker M, Lotz J, Frahm J. Real-time phase-contrast MRI of cardiovascular blood flow using undersampled radial fast low-angle shot and nonlinear inverse reconstruction. NMR Biomed 2012;25:917-24. [PubMed]
- Joseph A, Kowallick JT, Merboldt KD, Voit D, Schaetz S, Zhang S, Sohns JM, Lotz J, Frahm J. Real-time flow MRI of the aorta at a resolution of 40 msec. J Magn Reson Imaging 2014;40:206-13. [PubMed]
- Zhang S, Joseph AA, Voit D, Schaetz S, Merboldt KD, Unterberg-Buchwald C, Hennemuth A, Lotz J, Frahm J. Real-time magnetic resonance imaging of cardiac function and flow-recent progress. Quant Imaging Med Surg 2014;4:313-29. [PubMed]
- Eichenberger AC, Schwitter J, McKinnon GC, Debatin JF, von Schulthess GK. Phase-contrast echo-planar MR imaging: real-time quantification of flow and velocity patterns in the thoracic vessels induced by Valsalva's maneuver. J Magn Reson Imaging 1995;5:648-55. [PubMed]
- Gatehouse PD, Firmin DN, Collins S, Longmore DB. Real time blood flow imaging by spiral scan phase velocity mapping. Magn Reson Med 1994;31:504-12. [PubMed]
- Nayak KS, Pauly JM, Kerr AB, Hu BS, Nishimura DG. Real-time color flow MRI. Magn Reson Med 2000;43:251-8. [PubMed]
- Klein C, Schalla S, Schnackenburg B, Bornstedt A, Fleck E, Nagel E. Magnetic resonance flow measurements in real time: comparison with a standard gradient-echo technique. J Magn Reson Imaging 2001;14:306-10. [PubMed]
- Körperich H, Gieseke J, Barth P, Hoogeveen R, Esdorn H, Peterschröder A, Meyer H, Beerbaum P. Flow volume and shunt quantification in pediatric congenital heart disease by real-time magnetic resonance velocity mapping: a validation study. Circulation 2004;109:1987-93. [PubMed]
- Nezafat R, Kellman P, Derbyshire JA, McVeigh ER. Real-time blood flow imaging using autocalibrated spiral sensitivity encoding. Magn Reson Med 2005;54:1557-61. [PubMed]
- Steeden JA, Atkinson D, Taylor AM, Muthurangu V. Assessing vascular response to exercise using a combination of real-time spiral phase contrast MR and noninvasive blood pressure measurements. J Magn Reson Imaging 2010;31:997-1003. [PubMed]
- Frahm J, Schätz S, Untenberger M, Zhang S, Voit D, Merboldt KD, Sohns JM, Lotz J, Uecker M. On the temporal fidelity of nonlinear inverse reconstructions for real-time MRI – The motion challenge. The Open Med Imaging J 2014;8:1-7.
- Bland JM, Altman DG. Statistical methods for assessing agreement between two methods of clinical measurement. Lancet 1986;1:307-10. [PubMed]
- Altman DG. Statistics in the medical literature: 3. Stat Med 1999;18:487-90. [PubMed]