Comparison between tumour metabolism derived from 18F-FDG PET/CT and accurate cytogenetic stratification in newly diagnosed multiple myeloma patients
Introduction
Multiple myeloma (MM) is characterized by a monoclonal plasma cell disorder which represents 1% of all malignancies and 10% of hematologic malignancies and was responsible for 13,000 deaths over 2017 (1,2). The median age at diagnosis is 66–70 years old (yo). The disease is rare before 40 yo and slightly more frequent in men. Its main feature is its heterogeneity (clinical characteristics, genetics, survival, treatment response), revealing a complex pathophysiology. During the past two decades, significant therapeutic improvements have been made but this disease remains incurable (2). In this context, it appears worthwhile to perform early prognostic stratification to guide therapeutic strategies.
Over the last decade, there has been a growing interest in 18F-fluorodeoxyglucose positron emission tomography integrated with computed tomography (18F-FDG PET/CT) in MM. Indeed, it provides satisfying sensibility for lesion detection in symptomatic MM and allows an entire body examination with the possibility to find out extramedullary lesions (3-7). Furthermore, it has a prognostic value in smoldering myeloma, in symptomatic MM at baseline or in MM under treatment at each time of evaluation (3,4,8-19). Prognostic factors at baseline include: the presence of extramedullary disease (EMD), the number of focal lesions (FLs; >3), 18F-FDG avidity, total metabolic tumour volume (TMTV) and total lesion glycolysis (TLG) (4,10,11,14,20-22). In 2006, a revised version of the Durie and Salmon classification included 18F-FDG PET/CT and magnetic resonance imaging (MRI) of the spine and pelvis (23). Moreover, a correlation between PET and clinical/laboratory data such as medullary plasma cell burden, β2microglobulinemia and CD38/138 expression has been demonstrated (3,10,24,25).
Cytogenetic abnormalities are among the most powerful predictive factors of outcome in MM. Apart from the well-known deletion 17p (del17p) and translocation (4;14) [t(4;14)] mutations, which are not sufficient to explain myeloma outcomes heterogeneity, several studies tempted to demonstrate the impact of other anomalies on patient prognosis, with sometimes contradictory results (26-28). Recent studies, based on large patient series, have shown an additional effect of other mutations and an independent impact of trisomy’s (29). Whereas trisomy’s 3 and 5 are associated to a better prognosis, trisomy 21 negatively impacts survival, similarly to del1p32 or even 1q gain (30-32). Recently, a combined cytogenetic scoring system established in Toulouse demonstrated a better prognostic stratification at baseline, weighing the impact of six different abnormalities showed to be independently significant in terms of survival [t(4;14), del17p, trisomy 5, trisomy 21, del1p32, and 1q gain], adding them into the called linear predictor (LP) score, allowed patient classification in three distinct groups (33). A link between other genetic data and PET has been shown (24). A potential complementarity with PET data has also been suggested. Indeed, 18F-FDG PET/CT and genetic profile are independent prognostic factors, but positivity in both is linked to an even poorer prognosis (10,15,21).
The aim of our study was to examine the correlation between the new LP score and PET data among patients newly diagnosed for MM or secreting plasmacytoma, in particular through TMTV and/or TLG calculation, and their potential complementary role for prognostic stratification at baseline.
Methods
Patients
In this monocentric study, we retrospectively analysed 57 patients with a first diagnosis of MM or secreting plasmacytoma with minimal bone marrow involvement according to the updated diagnostic criteria of the IMWG in 2014, referred to our tertiary University Hospital from March 2012 to March 2019, who have undergone 18F-FDG PET/CT evaluation and bone marrow biopsy before treatment beginning, for whom EANM Research Ltd. (EARL) reconstructions were available (acquisitions on two different PET/CT systems). They all benefited of the standard treatment of MM: induction chemotherapy including Bortezomib, autologous stem cell transplantation (ASCT), consolidation therapy. Thirty-two of the patients underwent another 18F-FDG PET/CT after completion of induction chemotherapy. MM treatment indication was confirmed according to the International Myeloma Work Group (IMWG) criteria revised in 2014 [i.e., “CRAB” criteria (hypercalcemia, renal failure, anaemia, bone lesions) or at least one of the following criteria: more than one lesion ≥5 mm in MRI, bone marrow plasma cell burden >60% or serum free light chains (sFLC) ratio >100] (34). Positive diagnosis of secreting plasmacytoma was obtained by lesion biopsy as well as blood and bone marrow sampling. The study was conducted in accordance with the Declaration of Helsinki (as revised in 2013). According to institutional policy, approval from our Institutional Review Board was not required owing the retrospective nature of the study and individual consent for this retrospective analysis was waived.
18F-FDG PET/CT acquisitions
Whole body 18F-FDG PET/CT was performed sequentially at baseline for each patient using a dedicated PET/CT system (GEMINI TF, Philips Medical Systems, Eindhoven, The Netherlands or DISCOVERY MI, GE Healthcare, Milwaukee, USA). CT scan was used for attenuation correction and anatomic lesion location. PET data corrections were performed for dead time losses, subtraction of scattered coincidences and attenuation. Reconstructions were performed via the EARL harmonization program to ensure reproducibility of measures obtained on both systems (35). PET emission data were acquired in three-dimensional (3D) mode with a 19×192 matrix with 90 seconds (s) per bed position (DISCOVERY), and with a 144×144 matrix with 90 to 120 s per bed position (GEMINI TF). FDG was injected intravenously at the dose of 3 MBq/kg (Philips) or 2 MBq/kg (GE), after at least 6 hours of fasting and only if glycaemia assessed by the hexokinase method was lower than 120 mg/dL. Time delay between injection and acquisition was 60±10 min.
Image analysis
Criteria to define a significant lesion were as follows:
- Visually hypermetabolic focus exceeding the surrounding background intensity, irrespective of the mediastinal blood pool or liver background (with the exception of liver lesions) or underlying osteolysis presence;
- Visible on at least two consecutive slices, irrespective of their intensity or size;
- EMD if biopsy proven diagnosis was obtained or if there was no other plausible alternative diagnosis;
- Paramedullary disease (PMD) in case of extra-osseous extension of a primary bone lesion;
- Exclusion of joints, sites of metallic implants/artefacts, kyphoplasty, post-biopsy remodelling, fractures (possible inflammation that could be a confounding factor);
- Diffuse bone marrow involvement (DBI) in case of homogenous osteomedullary metabolism exceeding liver background intensity or heterogeneous osteomedullary metabolism partially exceeding liver background intensity, solely visually assessed.
All scans were read by two physicians, a consensus was established when conclusions were not exactly the same.
Calculation of TMTV and TLG
TMTV threshold was chosen upon a set of six thresholds (inclusion of voxels with intensity above the mediastinal blood pool background, hepatic background, spleen background, bone marrow background, voxels above 2.5 of the maximum standardized uptake value (SUVmax), or above 41% of the hot point of the lesion) and compared to the progression-free survival (PFS) (data and results not shown). Liver background intensity was retained [SUVmean in a 3-cm diameter volume of interest (VOI) at the junction of segments V and VIII or in healthy liver where appropriate]. Given the high proportion of patients with a zero-TMTV/TLG, we then decided to separate the cohort into three groups (zero-TMTV/TLG, positive TMTV/TLG but under median value, and positive TMTV/TLG above median value) to assess secondary endpoints. TMTV and TLG calculation was carried out via the free Beth Israel plugin for FIJI (Image J distribution), already used for metabolic tumour volume (MTV) calculation in Hodgkin lymphoma by Kanoun et al. (36,37). VOI were drawn on each lesion achieving positivity criteria and all pixels with intensity above the threshold were included in TMTV calculation, TMTV was defined by the sum of the volumes taken into account in each lesion (Figure 1). Physicians took care not to include normal physiological background pixels in measures. Several VOI drawings were allowed for a single lesion if there was a heterogeneous uptake (i.e., high local uptake), so as not to exclude significant pixels. TLG was defined by the sum of the glycolyses of each lesion, each lesion glycolyse being defined by the product of metabolic volume with the SUVmean within significant voxels of a single lesion.
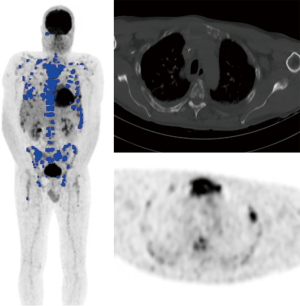
It is important to note that DBI was visually assessed and not included in TMTV/TLG calculation, including the possibility of patients with DBI but zero-TMTV/TLG: DBI reflects the extent of bone marrow infiltration, in contradiction with the capacity of plasma cells to agglutinate into a FL. These two pathological patterns theoretically don’t have the same signification in terms of prognosis (21), so we decided to separate them, not including DBI into TMTV/TLG calculation (neither in case of homogeneous uptake nor in case of heterogeneous uptake partially above hepatic background). Moreover, including DBI into TMTV/TLG calculation would have significate considering the whole red marrow volume, and FLs represent areas only filled with plasma cells whereas DBI reflects a variable percentage of malignant plasma cells among normal bone marrow cells, this is partially explained by FDG ability to show superimposed hypermetabolic foci on a visual aspect of DBI.
LP score determination
Bone marrow samples were all collected before treatment initiation and were transported overnight to a central laboratory. Mononuclear bone marrow cells were sorted with the RoboSep system (StemCell Technologies, Vancouver, BC, Canada). Analysis were validated only on samples with a rate higher than 70% of plasma cells after sorting (samples which were not achieving this rate because of insufficient plasma cell number were excluded from the analysis). Cytogenetic analyses were performed on sorted CD138+ magnetic-activated cells (Miltenyi Biotec, Paris, France), by fluorescence in situ hybridization (FISH) (Abbott, Paris, France) ± single nucleotide polymorphism (SNP) arrays (Affymetrix, Santa Clara, CA, USA), using the Cytoscan HD array kit (Affymetrix).
First, t(4;14) was evaluated by FISH with specific probes. Del17p positivity was defined by the presence of the mutation in at least 60% of isolated plasma cells, demonstrated by FISH or SNP arrays, with additional FISH analysis to quantify plasma cells involved with a specific probe TP53/CEP17 (Cytocell AmpliTech, Compiègne, France). The other significant baseline cytogenetic analyses (determination of the mutational status for trisomy 5, trisomy 21, 1q gain, del1p32) were determined by SNP-arrays. LP score is a 6-marker based weighted score, defined by the following algorithm: LP score = 0.4 ×t(4;14) + 1.2 × del17p – 0.3 × tri5 + 0.3 × tri21 + 0.5 × 1q gain + 0.8 × del1p32.
A score of ≤0 defined the group with a favourable cytogenetic prognosis (5-year relative survival >75%). A score [0;1] defined the group with intermediate prognosis (5-year relative survival 50–75%). A score >1 defined the high-risk cytogenetic group (5-year relative survival <50%).
Statistical analysis
Concerning primary endpoint analysis, correlation between TMTV or TLG and LP score was assessed by a Kruskal-Wallis test, as well as the evaluation of the correlation between TMTV or TLG and each of the mutations included in LP score calculation and the correlation between the number of FLs and LP score. A Chi-squared test was performed to assess the link between DBI and LP score. Concerning secondary endpoints, PFS evaluation according to baseline metabolic parameters, their variation post-induction, LP score or each of the mutations included in its calculation was performed. After determination of time to progression for each patient, Kaplan-Meier analysis and log-rank tests were performed. A Chi-squared test was used to assess of the potential link between DBI and PFS. Statistical analyses were performed on MedCalc software.
Results
Patient characteristics
General characteristics
Population characteristics are listed in Table 1. The 57 patients of the cohort, diagnosed between March 2012 and March 2019, were included for primary endpoint analysis, two of them were diagnosed with secreting plasmacytomas (bone locations only). According to first line treatment, only three patients didn’t receive Bortezomib in the first line. Mean follow-up was 36.9 (range, 10.7–86.4) months. Eighteen patients presented a progression during follow-up (32%) and one died of MM at 9.9 months of follow-up. The mean time to progression was 30.4 (range, 11.8–77.0) months.
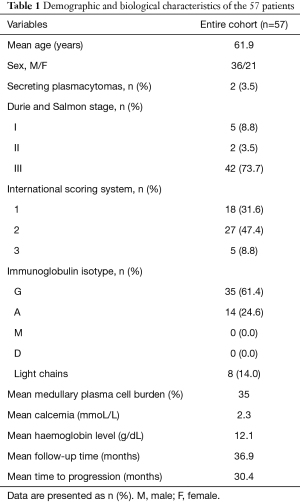
Full table
Distribution of volumetric and other 18F-FDG PET/CT data
Usual metabolic data are listed in Table 2. Among the 57 patients included in the primary endpoint analysis, 36 presented hypermetabolic FL (63.2%), with mean SUVmax at 7.53 (ranging from 2.41 to 36.02). Five patients (8.7%) presented PMD (4 with only 1 lesion, 1 with 3 lesions), 2 (3.5%) had EMD (1 and 3 lesions respectively). Twenty-seven patients had DBI suspicion (47.4%, bone marrow SUVmax ranging from 3.40 to 6.54). Twenty-one patients had a zero-TMTV/TLG, among which 8 had DBI (vs. 19 among the 36 patients with positive TMTV), 3 had solely hypermetabolic fractures and 2 had non-FDG-avid osteolysis (most probably due to MM). The number of visually negative (neither DBI nor FL) 18F-FDG PETs was 10 (17.5%). Median TMTV was 12.1 cc (mean, 59.3 cc), and median TLG was 26.4 g (mean, 187 g). Considering only patients with positive TMTV/TLG, median TMTV was 42.9 cc (mean, 93.9 cc, ranging from 0.51 to 945 cc), and median TLG was 143 g (mean, 297 g, ranging from 1.19 to 2,660 g).
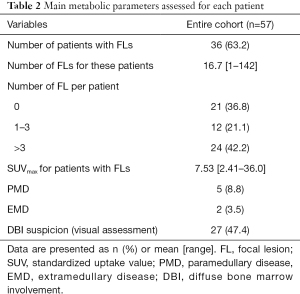
Full table
Cytogenetic data
Thirty-three patients had a LP score ≤0 (57.9%), 19 (33.3%) had a LP score [0;1] and the 5 others (8.8%) had a LP score ≥1. LP scores ranged from -0.3 to 1.7 (median, 0; mean, 0.3). Fifty patients (87.7%) presented at least one of the six anomalies included in LP score calculation. Trisomy 5 was found in 22 patients (38.6%), trisomy 21 in 16 (28.1%), t(4;14) in 2 (3.5%), 1q gain in 18 (31.6%), del1p32 in 5 (8.8%) and del17p in 3 (5.3%) patients.
Primary endpoint or association between metabolic parameters and LP score
The distribution of TMTV and TLG values between the three categories of LP-scores was almost stochastic, with no significant correlation (P=0.70 for each variable) (Figure 2).
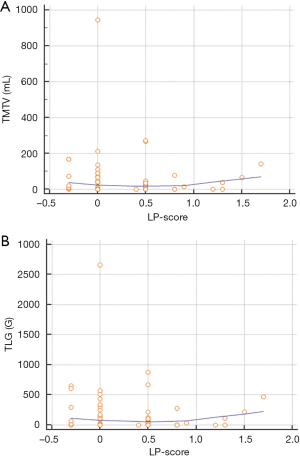
No significant correlation between TMTV or TLG and any of the anomalies included in LP score calculation was observed. A significant association between high suspicion of DBI and LP score was observed (P=0.036) (Table 3 and Figure 3). Moreover, there was a significant association between high suspicion of DBI and the presence of 1q gain (P=0.049), but not with the others mutations included in LP score calculation.
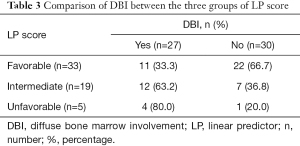
Full table
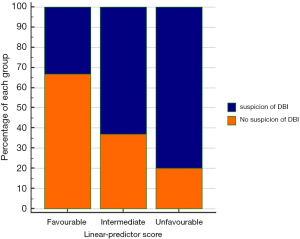
Secondary endpoints
No correlation was found between the presence of DBI and PFS (P=0.16). We didn’t observe any significant correlation between PFS and any quantitative metabolic parameters (TMTV, TLG, SUVmax, FLs at baseline, after induction treatment, and their variation). We didn’t obtain signification when comparing LP score stratification and PFS (P=0.44), probably because of the absence of progression among the patients of the high-risk group. Mean follow-up was 38.3, 36.3, and 29.2 months, respectively, for low, intermediate and high-risk subgroups of LP score. Considering the comparison between metabolic parameters and biological response after induction, ASCT or consolidation treatment, we found significant association between post-consolidation treatment biological response (i.e., at least very good partial response according to IMWG criteria) and TMTV, TLG and SUVmax at baseline (P=0.025, 0.039 and 0.03, respectively).
Discussion
18F-FDG PET/CT is one of the most interesting imaging modalities to assess baseline tumour burden in treatment-naïve MM patients and certainly the most valuable technique in treatment evaluation in routine. Furthermore, the Italian myeloma workgroup proposed promising standardized interpretation criteria, taking into consideration some elements until then neglected, such as fractures or Deauville scale (13).
Two previous studies evaluated the prognostic impact of metabolic tumour volume determined with FDG PET data (21,22). Additionally, possible link between genetics and tumour metabolism was studied, with results suggesting a complementarity between the two approaches (21). In their study, Fonti et al. didn’t include patients with no tumour metabolism (zero-TMTV/TLG), inducing a potential selection bias (22). As in McDonald study, we have chosen to include these patients to be representative of the general patient population in clinical practice (21). We found relatively similar patient percentages with zero-TMTV/TLG (36.8% vs. 32% for McDonald et al.) (21). Furthermore, distribution in terms of number of FLs in our study was slightly different compared to that of McDonald’s (21% vs. 31% of patients with 1 to 3 FLs, and 42 vs. 36% with more than 3 FLs, considering the whole cohort). Our population included all stages of Durie Salmon classification, as in McDonald et al. contrary to Fonti et al. study (only stages IIIA) (21,22).
We intentionally included patients with secreting plasmacytomas with minimal bone marrow involvement because of the relatively similar pathophysiology and because they could represent a particular form of MM even they presented no sign of systemic disease. This hypothesis is underpinned by the fact that patients presenting multiple secreting plasmacytomas could be at risk if treated only locally. Moreover, systemic treatment is similar to that of classic MM and MM arising from transformation of secreting plasmacytomas, as patients do not present a poorer prognosis when compared to primitive MM (38).
We decided not to include in TMTV/TLG assessment fracture sites, to consider only metabolism due to myelomatous lesion, consequently excluding post-traumatic reactive metabolism due to fracture consolidation. Vertebral fractures are seen in up to 70% of patients during MM evolution and are often difficult to interpret (39). Most of the fractures encountered in our series were costal lesions, with relatively small hypermetabolic foci. Basically, causes of false positives in FDG PET are metallic implants, inflammation/infection, post-biopsy remodelling, artefacts, fractures, high dose steroids and growth factors. There were only three patients with only fracture sites as hypermetabolic foci in our cohort.
We tested a potential correlation between cytogenetics and metabolic data. To our knowledge this has not been previously analysed as primary endpoint. Interestingly, we found no correlation between genetics and quantitative metabolic parameters, with a stochastic distribution of TMTV and TLG values among LP scores. It has been demonstrated a correlation between TMTV and TLG and survival in two studies, and between cytogenetics and survival in several studies. We wondered if genetic and metabolic data were linked or alternatively if complementarity was observed between the two types of data. Results obtained could suggest that cytogenetic analyses performed on iliac crest bone marrow samples are not predictive of high measurable tumour burden at baseline. To support this hypothesis, the study of Rasche et al. evaluating multisite biopsies among a cohort mainly composed of treatment-naïve MM patients, found a very poor concordance of mutational load between all samples of a single patient (40). Oncogenic drivers activated during MM evolution could explain such a variability in a pathologic plasma cell genome. As an example, it is possible that our results could have been totally different if cytogenetic analysis had been performed on a biopsy of the largest or the most FDG-avid lesion. There is a consensus regarding the non-feasibility of FL biopsy (often CT-guided) before treatment onset. Taking this into account, our work began to demonstrate the complementarity between PET-CT and LP-score: whereas LP-score indicates the “basal” mutational load on random bone marrow sample (iliac crest, which is at the moment the most profitable way to know genetic stratification), PET-CT is able to warn the clinician on the possibility of mutations all the more unfavourable within FLs (higher metabolism).
Moreover, the IMAJEM study showed a poor concordance between PET negativation and minimal residual disease assessment under treatment (4), supporting the hypothesis that FLs could represent containers of cells predisposing to relapse, even in the absence of circulating monoclonal component, in a particular micro-environment and thus particular selective pressure. Indeed, in a study performed in 2014 by Caers et al., 23% of patients reaching a classical biological complete response had hypermetabolic lesions yet, with a PFS significantly poorer when compared to patients without hypermetabolic lesions (12). All these different characteristics further complicate the understanding of MM physiopathology. In contrast, we found a significant association when comparing visual high suspicion of DBI and LP score (P=0.036). Indeed, visual determination of DBI represents a global assessment of medullary metabolic activity and LP score is calculated upon a systematic iliac crest medullogram (not focused on a FL), which could potentially explain our results.
Considering the distribution of each of the 6 genetic anomalies included in LP score calculation, our population was rather representative of the original study (33), except for the percentage of t(4;14), lower in our study (3.5% vs. 15%). This could have induced a selection bias in secondary endpoints analysis due to the fact that t(4;14) is the second most important mutation to lead to unfavourable prognostic according to LP score. Considering the proportion of each of LP score subgroups, our population seemed to be slightly different from the population in which the LP score was established (57.9% of favourable, 33.3% of intermediate and 8.8% of unfavourable prognosis, vs. respectively 40.2, 41.4 and 18.4%). As secondary endpoints, we evaluated the correlation between metabolic data and survival, and cytogenetic data and survival. Unfortunately, our cohort didn’t allow us to demonstrate any significant result. Indeed, mean follow-up was short (approximately 3 years, vs. more than 8 years for McDonald et al.), as well as the number of events (18 upon 57 patients, vs. nearly a half for McDonald et al.) (22). Ten patients were included with a follow-up shorter than 18 months, inducing selection bias.
There was no significant association between metabolic parameters and PFS. To our knowledge, there is no SUV cut-off for DBI established in literature, and considering hepatic background, as in our study, can probably induce an over-diagnosis of this parameter, making it no longer discriminant for prognostic stratification. Work is in progress in our department to quantify total medullary metabolic activity via bone segmentation on the CT part of our exams, in order to compare it to PFS. Unfortunately, we didn’t find any significant association between cytogenetics and PFS. It’s worth emphasizing that mean follow-up time was 38.3 months for patients with LP score ≤0, vs. 36.3 and 29.2 months respectively for patients with LP score [0;1] and >1, and that mean time to progression upon the whole cohort was 25.6 months.
Our study has some limitations. The main limitations of this study, in addition to the already-cited short follow-up, were the relatively low number of patients, the severe inclusion criteria and the retrospective nature of the study. Indeed, 18F-FDG PET/CT had to be done before treatment initiation and it is well known that some MM patients need to be treated urgently because of the life-threatening conditions. Moreover, PET has been recommended in initial MM evaluation relatively recently (since 2018) and most patients newly diagnosed in our centre since 2012 had conventional imaging modalities at baseline (5). Another difficulty we encountered with inclusion criteria was the need for EARL reconstruction DICOMs, which had not been saved systematically for all patients diagnosed with MM in our centre. We have lost at least a dozen patients due to the retrospective nature of this study. To our knowledge, this is the first time that such a calibration is performed during a study dealing with MM, to ensure measurements reproducibility between DICOMs obtained on more than a single device. EARL harmonization reconstruction program significantly improves delineation reproducibility in MTVs and has allowed a variability in SUV measurements lower than 10% between devices (35).
Conclusions
Our study showed a significant association between visual suspicion of DBI and LP score, presumably because of a genetic assessment based on systematic iliac crest bone marrow sample. On the contrary, quantitative metabolic data coming from 18F-FDG PET/CT, especially volumetric measurements, do not seem to be associated to cytogenetic data, suggesting a potential complementarity of these biomarkers for baseline MM stratification. However, none of the markers tested as secondary endpoints were significantly associated to survival, suggesting that this cohort should be enlarged and a longer follow-up time should be assessed.
Acknowledgments
Funding: None.
Footnote
Conflicts of Interest: All authors have completed the ICMJE uniform disclosure form (available at http://dx.doi.org/10.21037/qims-21-85). Dr. RL serves as an unpaid deputy editor of Quantitative Imaging in Medicine and Surgery. AC serves as an unpaid editorial board member of Quantitative Imaging in Medicine and Surgery. The other authors have no conflicts of interest to declare.
Ethical Statement: The authors are accountable for all aspects of the work in ensuring that questions related to the accuracy or integrity of any part of the work are appropriately investigated and resolved. The study was conducted in accordance with the Declaration of Helsinki (as revised in 2013). According to institutional policy, approval from our Institutional Review Board was not required owing the retrospective nature of the study and individual consent for this retrospective analysis was waived.
Open Access Statement: This is an Open Access article distributed in accordance with the Creative Commons Attribution-NonCommercial-NoDerivs 4.0 International License (CC BY-NC-ND 4.0), which permits the non-commercial replication and distribution of the article with the strict proviso that no changes or edits are made and the original work is properly cited (including links to both the formal publication through the relevant DOI and the license). See: https://creativecommons.org/licenses/by-nc-nd/4.0/.
References
- Hathi DK, DeLassus EN, Achilefu S, McConathy J, Shokeen M. Imaging melphalan therapy response in preclinical extramedullary multiple myeloma with 18F-FDOPA and 18F-FDG PET. J Nucl Med 2018;59:1551-7. [Crossref] [PubMed]
- Kazandjian D. Multiple myeloma epidemiology and survival: a unique malignancy. Semin Oncol 2016;43:676-81. [Crossref] [PubMed]
- Sager S, Ergül N, Ciftci H, Cetin G, Güner Sİ, Cermik TF. The value of FDG PET/CT in the initial staging and bone marrow involvement of patients with multiple myeloma. Skeletal Radiol 2011;40:843-7. [Crossref] [PubMed]
- Moreau P, Attal M, Caillot D, Macro M, Karlin L, Garderet L, et al. Prospective evaluation of magnetic resonance imaging and 18F-Fluorodeoxyglucose positron emission tomography-computed tomography at diagnosis and before maintenance therapy in symptomatic patients with multiple myeloma included in the IFM/DFCI 2009 trial: Results of the IMAJEM study. J Clin Oncol 2017;35:2911-8. [Crossref] [PubMed]
- Cavo M, Terpos E, Nanni C, Moreau P, Lentzsch S, Zweegman S, et al. Role of 18F-FDG PET/CT in the diagnosis and management of multiple myeloma and other plasma cell disorders: a consensus statement by the International Myeloma Working Group. Lancet Oncol 2017;18:e206-17. [Crossref] [PubMed]
- Batsukh K, Lee SE, Min GJ, Park SS, Jeon YW, Yoon JH, Cho BS, Eom KS, Kim YJ, Kim HJ, Lee S, Cho SG, Kim DW, Lee JW, Min WS, Min CK. Distinct clinical outcomes between paramedullary and extramedullary lesions in newly diagnosed multiple myeloma. Immune Netw 2017;17:250-60. [Crossref] [PubMed]
- Usmani SZ, Heuck C, Mitchell A, Szymonifka J, Nair B, Hoering A, Alsayed Y, Waheed S, Haider S, Restrepo A, Van Rhee F, Crowley J, Barlogie B. Extramedullary disease portends poor prognosis in multiple myeloma and is over-represented in high-risk disease even in the era of novel agents. Haematologica 2012;97:1761-7. [Crossref] [PubMed]
- Zamagni E, Nanni C, Gay F, Pezzi A, Patriarca F, Bellò M, et al. 18F-FDG PET/CT focal, but not osteolytic, lesions predict the progression of smoldering myeloma to active disease. Leukemia 2016;30:417-22. [Crossref] [PubMed]
- Siontis B, Kumar S, Dispenzieri A, Drake MT, Lacy MQ, Buadi F, Dingli D, Kapoor P, Gonsalves W, Gertz MA, Rajkumar SV. Positron emission tomography-computed tomography in the diagnostic evaluation of smoldering multiple myeloma: Identification of patients needing therapy. Blood Cancer J 2015;5:e364 [Crossref] [PubMed]
- Bartel TB, Haessler J, Brown TLY, Shaughnessy JD, van Rhee F, Anaissie E, Alpe T, Angtuaco E, Walker R, Epstein J, Crowley J, Barlogie B. F18-fluorodeoxyglucose positron emission tomography in the context of other imaging techniques and prognostic factors in multiple myeloma. Blood 2009;114:2068-76. [Crossref] [PubMed]
- Zamagni E, Patriarca F, Nanni C, Zannetti B, Englaro E, Pezzi A, Tacchetti P, Buttignol S, Perrone G, Brioli A, Pantani L, Terragna C, Carobolante F, Baccarani M, Fanin R, Fanti S, Cavo M. Prognostic relevance of 18-F FDG PET/CT in newly diagnosed multiple myeloma patients treated with up-front autologous transplantation. Blood 2011;118:5989-95. [Crossref] [PubMed]
- Caers J, Withofs N, Hillengass J, Simoni P, Zamagni E, Hustinx R, Beguin Y. The role of positron emission tomography-computed tomography and magnetic resonance imaging in diagnosis and follow up of multiple myeloma. Haematologica 2014;99:629-37. [Crossref] [PubMed]
- Nanni C, Versari A, Chauvie S, Bertone E, Bianchi A, Rensi M, Bellò M, Gallamini A, Patriarca F, Gay F, Gamberi B, Ghedini P, Cavo M, Fanti S, Zamagni E. Interpretation criteria for FDG PET/CT in multiple myeloma (IMPeTUs): final results. IMPeTUs (Italian myeloma criteria for PET USe). Eur J Nucl Med Mol Imaging 2018;45:712-9. [Crossref] [PubMed]
- Usmani SZ, Mitchell A, Waheed S, Crowley J, Hoering A, Petty N, Brown T, Bartel T, Anaissie E, van Rhee F, Barlogie B. Prognostic implications of serial 18-fluoro-deoxyglucose emission tomography in multiple myeloma treated with total therapy 3. Blood 2013;121:1819-23. [Crossref] [PubMed]
- Davies FE, Rosenthal A, Rasche L, Petty NM, McDonald JE, Ntambi JA, Steward DM, Panozzo SB, van Rhee F, Zangari M, Schinke CD, Thanendrarajan S, Walker B, Weinhold N, Barlogie B, Hoering A, Morgan GJ. Treatment to suppression of focal lesions on positron emission tomography-computed tomography is a therapeutic goal in newly diagnosed multiple myeloma. Haematologica 2018;103:1047-53. [Crossref] [PubMed]
- Stolzenburg A, Lückerath K, Samnick S, Speer M, Kneer K, Schmid JS, Grigoleit GU, Hofmann S, Beer AJ, Bunjes D, Knop S, Buck AK, Einsele H, Lapa C. Prognostic value of 18F-FDG-PET/CT in multiple myeloma patients before and after allogeneic hematopoietic cell transplantation. Eur J Nucl Med Mol Imaging 2018;45:1694-704. [Crossref] [PubMed]
- de Waal EGM, Glaudemans AWJM, Schröder CP, Vellenga E, Slart RHJA. Nuclear medicine imaging of multiple myeloma, particularly in the relapsed setting. Eur J Nucl Med Mol Imaging 2017;44:332-41. [Crossref] [PubMed]
- Nanni C, Zamagni E. Therapy assessment in multiple myeloma with PET. Eur J Nucl Med Mol Imaging 2017;44:111-7. [Crossref] [PubMed]
- Spinnato P, Bazzocchi A, Brioli A, Nanni C, Zamagni E, Albisinni U, Cavo M, Fanti S, Battista G, Salizzoni E. Contrast enhanced MRI and 18F-FDG PET-CT in the assessment of multiple myeloma: a comparison of results in different phases of the disease. Eur J Radiol 2012;81:4013-8. [Crossref] [PubMed]
- Tu H, He Y, Huang T, Choe S. lan X, Duan X, Sattar H, Li C, Zhou F. Predictive value of 18F-FDG PET/CT scanning in combination with clinical parameters in patients with newly diagnosed multiple myeloma. Eur J Haematol 2018;100:189-97. [Crossref] [PubMed]
- McDonald JE, Kessler MM, Gardner MW, Buros AF, Ntambi JA, Waheed S, van Rhee F, Zangari M, Heuck CJ, Petty N, Schinke C, Thanendrarajan S, Mitchell A, Hoering A, Barlogie B, Morgan GJ, Davies FE. Assessment of total lesion glycolysis by 18F FDG PET/CT significantly improves prognostic value of GEP and ISS in myeloma. Clin Cancer Res 2017;23:1981-7. [Crossref] [PubMed]
- Fonti R, Larobina M, Del Vecchio S, De Luca S, Fabbricini R, Catalano L, Pane F, Salvatore M, Pace L. Metabolic tumor volume assessed by 18F-FDG PET/CT for the prediction of outcome in patients with multiple myeloma. J Nucl Med 2012;53:1829-35. [Crossref] [PubMed]
- Durie BGM. The role of anatomic and functional staging in myeloma: description of Durie/Salmon plus staging system. Eur J Cancer 2006;42:1539-43. [Crossref] [PubMed]
- Waheed S, Mitchell A, Usmani S, Epstein J, Yaccoby S, Nair B, van Hemert R, Angtuaco E, Brown T, Bartel T, McDonald J, Anaissie E, van Rhee F, Crowley J, Barlogie B. Standard and novel imaging methods for multiple myeloma: correlates with prognostic laboratory variables including gene expression profiling data. Haematologica 2013;98:71-8. [Crossref] [PubMed]
- Elliott BM, Peti S, Osman K, Scigliano E, Lee D, Isola L, Kostakoglu L. Combining FDG-PET/CT with laboratory data yields superior results for prediction of relapse in multiple myeloma: FDG-PET/CT predicts relapse in MM. Eur J Haematol 2011;86:289-98. [Crossref] [PubMed]
- Moreau P, Cavo M, Sonneveld P, Rosinol L, Attal M, Pezzi A, et al. Combination of international scoring system 3, high lactate dehydrogenase, and t(4;14) and/or del(17p) identifies patients with multiple myeloma (MM) treated with front-line autologous stem-cell transplantation at high risk of early MM progression–related death. J Clin Oncol 2014;32:2173-80. [Crossref] [PubMed]
- Kumar S, Fonseca R, Ketterling RP, Dispenzieri A, Lacy MQ, Gertz MA, Hayman SR, Buadi FK, Dingli D, Knudson RA, Greenberg A, Russell SJ, Zeldenrust SR, Lust JA, Kyle RA, Bergsagel L, Rajkumar SV. Trisomies in multiple myeloma: Impact on survival in patients with high-risk cytogenetics. Blood 2012;119:2100-5. [Crossref] [PubMed]
- Pawlyn C, Melchor L, Murison A, Wardell CP, Brioli A, Boyle EM, Kaiser MF, Walker BA, Begum DB, Dahir NB, Proszek P, Gregory WM, Drayson MT, Jackson GH, Ross FM, Davies FE, Morgan GJ. Coexistent hyperdiploidy does not abrogate poor prognosis in myeloma with adverse cytogenetics and may precede IGH translocations. Blood 2015;125:831-40. [Crossref] [PubMed]
- Hebraud B, Magrangeas F, Cleynen A, Lauwers-Cances V, Chretien ML, Hulin C, et al. Role of additional chromosomal changes in the prognostic value of t(4;14) and del(17p) in multiple myeloma: The IFM experience. Blood 2015;125:2095-100. [Crossref] [PubMed]
- Chretien ML, Corre J, Lauwers-Cances V, Magrangeas F, Cleynen A, Yon E, et al. Understanding the role of hyperdiploidy in myeloma prognosis: Which trisomies really matter? Blood 2015;126:2713-9. [Crossref] [PubMed]
- Hebraud B, Leleu X, Lauwers-Cances V, Roussel M, Caillot D, Marit G, et al. Deletion of the 1p32 region is a major independent prognostic factor in young patients with myeloma: the IFM experience on 1195 patients. Leukemia 2014;28:675-9. [Crossref] [PubMed]
- Hanamura I. Frequent gain of chromosome band 1q21 in plasma-cell dyscrasias detected by fluorescence in situ hybridization: incidence increases from MGUS to relapsed myeloma and is related to prognosis and disease progression following tandem stem-cell transplantation. Blood 2006;108:1724-32. [Crossref] [PubMed]
- Perrot A, Lauwers-Cances V, Tournay E, Hulin C, Chretien ML, Royer B, et al. Development and validation of a cytogenetic prognostic index predicting survival in multiple myeloma. J Clin Oncol 2019;37:1657-65. [Crossref] [PubMed]
- Rajkumar SV, Dimopoulos MA, Palumbo A, Blade J, Merlini G, Mateos MV, et al. International Myeloma Working Group updated criteria for the diagnosis of multiple myeloma. Lancet Oncol 2014;15:e538-48. [Crossref] [PubMed]
- Houdu B, Lasnon C, Licaj I, Thomas G, Do P, Guizard AV, Desmonts C, Aide N. Why harmonization is needed when using FDG PET/CT as a prognosticator: Demonstration with EARL-compliant SUV as an independent prognostic factor in lung cancer. Eur J Nucl Med Mol Imaging 2019;46:421-8. [Crossref] [PubMed]
- Kanoun S, Tal I, Berriolo-Riedinger A, Rossi C, Riedinger JM, Vrigneaud JM, Legrand L, Humbert O, Casasnovas O, Brunotte F, Cochet A. Influence of software tool and methodological aspects of total metabolic tumor volume calculation on baseline 18F-FDG PET to predict survival in Hodgkin lymphoma. PLoS One 2015;10:e0140830 [Crossref] [PubMed]
- Kanoun S, Rossi C, Berriolo-Riedinger A, Dygai-Cochet I, Cochet A, Humbert O, Toubeau M, Ferrant E, Brunotte F, Casasnovas RO. Baseline metabolic tumour volume is an independent prognostic factor in Hodgkin lymphoma. Eur J Nucl Med Mol Imaging 2014;41:1735-43. [Crossref] [PubMed]
- Fouquet G, Guidez S, Herbaux C, Van de Wyngaert Z, Bonnet S, Beauvais D, Demarquette H, Adib S, Hivert B, Wemeau M, Berthon C, Terriou L, Coiteux V, Macro M, Decaux O, Facon T, Huglo D, Leleu X. Impact of initial FDG-PET/CT and serum-free light chain on transformation of conventionally defined solitary plasmacytoma to multiple myeloma. Clin Cancer Res 2014;20:3254-60. [Crossref] [PubMed]
- Mulligan M, Chirindel A, Karchevsky M. Characterizing and predicting pathologic spine fractures in myeloma patients with FDG PET/CT and MR imaging. Cancer Invest 2011;29:370-6. [Crossref] [PubMed]
- Rasche L, Chavan SS, Stephens OW, Patel PH, Tytarenko R, Ashby C, et al. Spatial genomic heterogeneity in multiple myeloma revealed by multi-region sequencing. Nat Commun 2017;8:268. [Crossref] [PubMed]