Surgical ligation level of the bronchial artery influences tissue oxygen saturation of the bronchus and the incidence of postoperative bronchofistula after pulmonary lobectomy
Introduction
Postoperative leakage of a surgical anastomosis, such as those in the intestinal tract or bronchus, is a major postoperative complication (1,2). Suture failure occurs when local blood flow in the affected tissue is reduced, which causes hypoxia in the sutured tissue and delays the healing process. Therefore, preserving locally distributed blood vessels to maintain blood flow to sutured tissue is crucial.
Bronchopleural fistula (BPF) is one of the most serious complications of lobectomy (2). The incidence of a BPF following a lobectomy is 0.5%, and the mortality rate of patients with a BPF is 27% (3-5). BPFs most often occur following right lower lobectomies and have a postoperative incidence rate of 1.1–1.7% (6). BPF is also common after pneumonectomy, and the incidence is higher (4.5%) than after lobectomy (5). Decreased local blood flow is generally the main cause of delayed healing or suture failure. BPF may be also caused by decreased blood flow through bronchial arteries at the bronchial stump.
Bronchial arteries branch right and left from the descending aorta and the intercostal arteries and feed the lungs and bronchi (7). From the right bronchial artery, multiple branches run into the periphery of the lower right lobe. One artery descends from the carina inside the bronchi. Another artery branches to the upper right lobe and then down to the middle trunk, exits the middle lobe bronchus, and finally courses into the lower right lobe bronchus (8-10).
The standard procedure for the curative resection of lung cancer in the right lower lobe is a right lower lobectomy and mediastinal lymph node dissection (11). In this procedure, the hilar lymphatic tissue that covers the bronchus is excised to remove the metastatic lymph nodes. For the lobectomy of the lower lobe, the lymphatic tissue on both the outside and inside of the inferior trunk of the bronchus is dissected during lymph node dissection, and the peripheral branches of the bronchial arteries are ligated along with the lymph nodes. Ligation of the bronchial arteries in the central part of the lobe results in reduced tissue oxygenation in the peripheral bronchus.
Indocyanine green (ICG) fluorescence has previously been used to evaluate blood flow during surgery. The ICG fluorescence technique evaluates blood flow by visualizing the fluorescence of ICG in the blood, as it is administered systemically from outside of the body. This visualization is possible with a near-infrared camera because the wavelength of the light fluorescence excitation is in the near-infrared region, and there is a high level of tissue permeability. This technique is safe for the human body and is often used to monitor coronary artery bypass blood flow and muscle valve blood flow (12-15). Blood flow in the bronchial arteries can be visualized with ICG fluorescence during thoracic surgery for lung cancer (Figure 1). However, this qualitative evaluation only determines the presence or absence of fluorescence. Quantitation of tissue oxygenation levels is difficult with this modality.
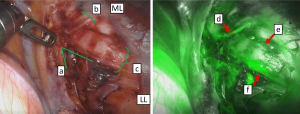
By contrast, the tissue oxygen saturation (TOS)-OR regional oxygen saturation (rSO2) monitor (Fujita Medical Instruments, Tokyo, Japan) (16) and the INVOS system (Medtronic, Tokyo, Japan) (17) have been used to quantitatively measure TOS levels using a non-invasive near-infrared spectroscopy technique. In this technique, the near-infrared light that has entered the tissue is absorbed by oxygenated and deoxygenated hemoglobin (Hb). The oxygen saturation can then be measured from the varying Hb absorption rates because oxygenated and deoxygenated Hb have different near-infrared light absorption characteristics (18).
The TOS-OR rSO2 monitor and INVOS system can measure oxygen saturation in tissue at depths of 2–3 cm or more (18). They are frequently used to monitor cerebral blood flow during general anesthesia surgery and evaluate blood flow in pedicled flaps in the field of plastic surgery. While these tools may be used to measure oxygen saturation in the deep parts of thick tissues, they cannot quantify oxygen saturation in thin tissues, such as the bronchus or intestinal tract, with a thickness of <1 cm.
In this study, we developed a new micro-TOS system that can semi-quantify oxygen saturation in thin tissues. Then, we used a biological pig lobectomy model to investigate the changes in blood flow at the bronchial stump, following lymph node dissection and bronchial artery amputation. Thus, this study aimed to clarify the influence of the level of the surgical ligation of the bronchial arteries on the reduction of TOS at the bronchial stump, allowing us to identify a surgical procedure that reduces the risk of a bronchopleural fistula following a right lower pulmonary lobectomy and the appropriate bronchial artery amputation site that could be used in future lobectomies.
Methods
Development of the micro-TOS system
A sensor compatible with the TOS-OR rSO2 monitor (Fujita Medical Instrument) was shaped into a 250-mm rod (Figure 2) for use in a minimally invasive endoscopic surgery. A light source and a sensor were built into the tip of the stainless-steel rod at a 30° angle. An ultra-small infrared light-emitting diode (LED) light source and two types of infrared light sensors were placed at the tip of the rod. The tips of the sensors were covered with black resin for measurement of the intended targets under light shielding. Three wavelengths of light (770, 805, and 870 nm) were irradiated from the LED light source for measurement. They were received for measurement by a sensor 6 mm away from the source. By attaching this custom sensor to the bronchial wall, TOS could be measured at a depth of 4–5 mm from the surface of the bronchus. The device captured 10 continuous time series data every second. Validation testing of the micro-TOS system was performed using the small intestine of a live porcine, as the organ has a simple anatomical structure and it is easy to surgically control the blood flow. While blood flow through the intestinal serosa was maintained, the rSO2 level was 80.7%±1.7%, but the rSO2 level decreased to 29.4%±2.5% in the ischemic state. The blood vessels of the intestinal tract that we wished to evaluate were ligated, and both sides of the intestinal tract were blocked with clamp forceps to inhibit mucosal blood flow. We were then able to accurately determine the TOS of the intestinal wall of several millimeters (figure not shown).
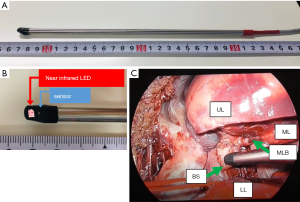
Animal experiment
Experiments were performed under a project license (No. 015-2019) granted by the institutional ethics board of the Foundation for Kobe International Medical Alliance, Kobe, Japan, in compliance with the institutional guidelines for the care and use of animals.
Specific pathogen-free porcine models (body weight 45±5 kg; male) were used for this experiment (n=2). The pre-carry-in treatment was as follows: A mixture of ketamine (10 mg/kg; 500 mg for intramuscular injection of Ketalal: Daiichi Sankyo Propharma Co., Ltd.) and xylazine (2 mg/kg; Celactal 2% injection: Bayer Medical Co., Ltd.) was administered intramuscularly, and atropine sulfate (0.5 mg/head; atropine sulfate injection 0.5 mg “Tanabe”, Mitsubishi Tanabe Pharma Corporation) was administered intravenously for sedation and prevention of casting and pulse elimination.
The porcine models were anesthetized with inhaled isoflurane (5% concentration, 3% oxygen flow; Escaine: Mylan Pharmaceutical Co., Ltd.) until the jaw muscles relaxed and tracheal intubation was possible. Thereafter, sevoflurane (2–5%) was used for continued inhalation anesthesia, and an infusion of saline or extracellular fluid solution (Soldem 3A) was started. Intravenous rocuronium bromide (0.6 L/kg) or vecuronium bromide (masculate; 0.1 mg/kg) was administered as a muscle relaxant, and the models were attached to a ventilator.
Evaluation of rSO2 at the bronchial stump during a right lower lobectomy
Under general anesthesia, the right lung was collapsed by separate lung ventilation, followed by thoracoscopic surgery. Then, thoracoscopic surgery was performed as follows: The pulmonary artery, inferior pulmonary vein, and lower lobe bronchus were cut with a stapler (Powered Echelon 45 mm, Ethicon Endosurgery, Johnson and Johnson, Tokyo, Japan), and the lower lobe was resected.
Firstly, the rSO2 level was measured at multiple points in the right bronchus: The rSO2 level of the main bronchus (MB), intermediate bronchial trunk (IT), and bronchial stump (BS) were measured after the right lower lobectomy (BS1), when the middle bronchus was clamped with forceps (BS2) and when the clamp was released (BS3) (Figure 3). The rSO2 level of the bronchial stump was measured on the site 5 mm of the central side of the stump. The micro-TOS sensor was applied to the target area, and measurements were obtained every second for 10 seconds once the value had stabilized. When all bronchial arteries were preserved, the rSO2 level of the bronchial wall was used as the positive control, and when all bronchial arteries were completely blocked, the rSO2 level of the bronchial wall was used as the negative control.
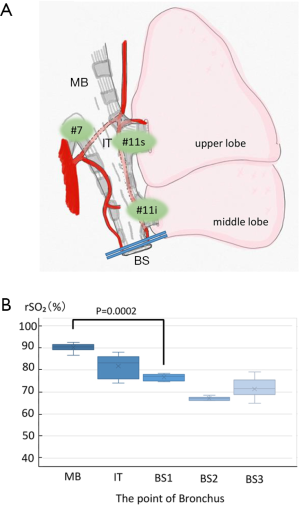
Secondly, the rSO2 level was measured at the fixed point of the bronchial stump. Multiple points of the bronchial arteries were then cut in stages as follows (Figure 4): Every bronchial artery was preserved before the lower lobe resection (i), the bronchial artery running from below to inside the trachea was ligated (ii), right lower lobe bronchotomy was performed (iii), a bronchial artery was ligated at the outside of the bronchi at the middle bronchial level (iv), and finally, a bronchial artery was ligated at the right main bronchial level (v).
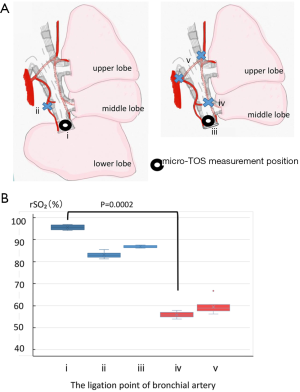
Statistical methods
The Wilcoxon or Kruskal-Wallis test was used to determine any significant differences between the two unpaired groups. Analysis was performed using the JMP®14.2 statistical analysis software (SAS Institute Inc., Cary, NC). The significance level was set at P<0.01.
Results
The rSO2 level was measured at each point in the right bronchus (Figure 3), and the rSO2 level near the bronchial stump was semi-quantified. The rSO2 level of the preserved bronchial wall was 90.5%±1.8% in the right main bronchus, which was defined as the upper limit positive control (Figure 3, MB). In the preserved bronchial artery, the rSO2 level at every point was semi-quantified with the micro-TOS sensor. The rSO2 level at the central end of the middle bronchial trunk (near #11, inter-lobar nodes) was 83.2%±5.1% (Figure 3, IT), and the rSO2 level in the bronchial stump was 77.1%±1.4% (Figure 3, BS1). The rSO2 level at the center of the bronchus was significantly higher than that of the bronchial stump (P=0.0002). When the bronchial stump was centrally clamped, the rSO2 level in the bronchial stump decreased to 67.1%±0.8% (Figure 3, BS2). This value was defined as the negative control. When the clamp was released, the rSO2 level in the bronchial stump increased to 71.5%±4.6% (Figure 3, BS3).
The rSO2 level was then evaluated at a fixed point in the bronchial stump when the bronchial artery was cut at different levels (Figure 4). When the bronchial artery was preserved, the rSO2 level was 95.5%±1.0% (Figure 4, i). The bronchi and bronchial arteries were then sequentially dissected from the periphery toward the center. First, the descending bronchial artery on the medial side of the bronchial bifurcation was cut, and the rSO2 level of the bronchial wall decreased slightly to 82.8%±1.3% (Figure 4, ii). Then, the bronchi were cut, and the rSO2 level remained at a similar level at 86.7%±0.5% (Figure 4, iii). The descending bronchial artery on the outside of the middle bronchus was then cut, and the rSO2 level abruptly decreased to 55.7%±1.2% (Figure 4, iv). Finally, the right bronchial artery was cut at the level of the right main bronchus, and the rSO2 level remained relatively constant at 58.9%±2.9% (Figure 4, v).
Discussion
To the best of our knowledge, this study is the first to semi-quantitatively measure blood flow at the bronchial stump during the transection of the bronchial artery. The bronchial arteries of the right lower lobe are distributed from the lateral side (middle bronchial trunk or outside) and medial side (tracheal bifurcation or inside). The rSO2 level significantly reduced to <60% (P=0.0002) when the bronchial artery branch, running at the high level on the lateral side of the bronchus, and the bronchial artery branch, running on the medial side, were severed.
A previous study examined extracorporeal circulation in the brain region in a cardiovascular surgery setting and showed that postoperative brain injury, such as cerebral infarction and intellectual impairment, occurred if the rSO2 level measured with a forehead probe dropped to ≤55–60% for longer than 5 min or decreased to an initial range of 76–86% (19,20).
The lower limit of the TOS required to prevent hypoxic tissue damage in the bronchus is currently unknown; however, with reference to other tissues, the TOS of bronchial tissues may need to be kept >60%. In this study, the central transection of the bronchial artery reduced the rSO2 level to <60%, indicating that cutting the bronchial artery at the central level may increase the risk of suture failure at the bronchial stump.
A BPF does not always occur when the bronchial artery is completely cut because blood flow to the bronchi is supplied from the bronchial artery and pulmonary circulation (8). For example, in lung transplantation, the bronchial artery is not anastomosed; however, the donor lung is still able to successfully engraft in the recipient.
In this study, we demonstrated that maintaining the blood flow of the bronchial artery into the middle lobe kept the rSO2 of the bronchial stump at an adequate level. Blood possibly flows into the lower lobe bronchus through the capillaries of the bronchial mucosa and submucosa between the middle and lower lobe bronchi. Individual differences can be observed in the degree of development of the bronchial arteries that flow into the remaining right middle lobe and the quality of oxygenation of the pulmonary circulation system in the right lung. Therefore, there may be differences in the amount of oxygen supplied to the lower lobe bronchial stump via the mucosa and submucosa. In this study, when at least one of the two inner or outer bronchial arteries were preserved, the TOS at the bronchial stump was maintained with an rSO2 level of ≥80%. When both arteries were cut, the rSO2 level dropped to <60%, and the risk of developing a bronchial stump fistula may have increased.
In clinical cases, additional reinforcement, such as covering the bronchial stump with a pericardial fat-tissue flap or intercostal muscle flap (21-23), may be considered to further reduce the risk of a bronchofistula. However, it is unclear whether strengthening the anastomotic site by covering it with other tissues may prevent suture failure.
Limitations
To adhere to the bioethical considerations for large animals, this pilot study used a minimum number of animals. In addition, pigs were chosen as our animal model because their physical size is closest to humans; however, the micro-TOS system has yet to be validated for use in human lobectomies in clinical studies.
Conclusions
The relationship between the surgical ligation level of the bronchial artery and the desaturation of the bronchial stump during a lobectomy was clarified in this porcine model study. During hilar lymph node dissection around the bronchus in the right lower lobe, central ligation of the bronchial artery may cause severe hypoxia at the bronchial stump. By preserving either the inside or outside of the bronchial artery running through the bronchial wall to the periphery, TOS at the bronchial stump may be maintained above the level at which hypoxia occurs.
Acknowledgments
We appreciated Fujita Medical Instruments Co., Ltd. (Tokyo, Japan) for kindly providing the TOS system for this research. We would like to especially thank Mr. Haruo Yamamura (TOSTEC Co., Ltd., Tokyo, Japan) for the development of the rod-shaped micro-TOS sensor. We would also like to thank Ms. Ami Yamanaka for her assistance in animal experiments. We thank Mr. Satoshi Haneda, Olympus Medical (Tokyo, Japan), for his technical assistance in handling the optical instruments. In addition, we thank Ms. Satoko Kimura and Mr. Toshiaki Ishibashi, MeDIP: Medical Device Innovation Platform, Foundation for Kobe International Medical Alliance (Hyogo, Japan), for providing the instruments necessary for the animal experiments.
Funding: The institutional research support grant of Kochi Medical School Hospital (Incentive grant #51010643), 2019.
Footnote
Conflicts of Interest: All authors have completed the ICMJE uniform disclosure form (available at http://dx.doi.org/10.21037/qims-20-1057). The authors have no conflicts of interest to declare.
Ethical Statement: Experiments were performed under a project license (No. 015-2019) granted by the institutional ethics board of the Foundation for Kobe International Medical Alliance, Kobe, Japan, in compliance with the institutional guidelines for the care and use of animals.
Open Access Statement: This is an Open Access article distributed in accordance with the Creative Commons Attribution-NonCommercial-NoDerivs 4.0 International License (CC BY-NC-ND 4.0), which permits the non-commercial replication and distribution of the article with the strict proviso that no changes or edits are made and the original work is properly cited (including links to both the formal publication through the relevant DOI and the license). See: https://creativecommons.org/licenses/by-nc-nd/4.0/.
References
- Buchs NC, Gervaz P, Secic M, Bucher P, Mugnier-Konrad B, Morel P. Incidence, consequences, and risk factors for anastomotic dehiscence after colorectal surgery: a prospective monocentric study. Int J Colorectal Dis 2008;23:265-270. [Crossref] [PubMed]
- Allen MS, Darling GE, Pechet TTV, Mitchell JD, Herndon JE 2nd, Landreneau RJ, Inculet RI, Jones DR, Meyers BF, Harpole DH, Putnam JB Jr, Rusch VW. ACOSOG Z0030 Study Group. Morbidity and mortality of major pulmonary resections in patients with early-stage lung cancer: initial results of the randomized, prospective ACOSOG Z0030 trial. Ann Thorac Surg 2006;81:1013-1019; discussion 1019-1020. [Crossref] [PubMed]
- Cerfolio RJ. The incidence, etiology, and prevention of postresectional bronchopleural fistula. Semin Thorac Cardiovasc Surg 2001;13:3-7. [Crossref] [PubMed]
- Sirbu H, Busch T, Aleksic I, Schreiner W, Oster O, Dalichau H. Bronchopleural fistula in the surgery of non-small cell lung cancer: incidence, risk factors, and management. Ann Thorac Cardiovasc Surg 2001;7:330-6. [PubMed]
- Asamura H, Naruke T, Tsuchiya R, Goya T, Kondo H, Suemasu K. Bronchopleural fistulas associated with lung cancer operations: univariate and multivariate analysis of risk factors, management, and outcome. J Thorac Cardiovasc Surg 1992;104:1456-64. [Crossref] [PubMed]
- Vester SR, Faber LP, Kittle CF, Warren WH, Jensik RJ. Bronchopleural fistula after stapled closure of bronchus. Ann Thorac Surg 1991;52:1253-1257; discussion 1257-1258. [Crossref] [PubMed]
- Cauldwell EW, Siekert RG. The bronchial arteries; an anatomic study of 150 human cadavers. Surg Gynecol Obstet 1948;86:395-412. [PubMed]
- Barman SA, Ardell JL, Parker JC, Perry ML, Taylor AE. Pulmonary and systemic blood flow contributions to upper airways in canine lung. Am J Physiol 1988;255:H1130-5. [PubMed]
- Nathan H, Orda R, Barkay M. The right bronchial artery. Anatomical considerations and surgical approach. Thorax 1970;25:328-333. [Crossref] [PubMed]
- Salassa JR, Pearson BW, Payne WS. Gross and microscopical blood supply of the trachea. Ann Thorac Surg 1977;24:100-7. [Crossref] [PubMed]
- Ginsberg RJ, Rubinstein LV. Randomized trial of lobectomy versus limited resection for T1 N0 non-small cell lung cancer. Ann Thorac Surg 1995;60:615-22; discussion 622-3. [Crossref] [PubMed]
- Novotny HR, Alvis D. A method of photographing fluorescence in circulating blood of the human eye. Tech Doc Rep SAMTDR USAF Sch Aerosp Med 1960;60-82:1-4.
- Mitsuhashi N, Kimura F, Shimizu H, Imamaki M, Yoshidome H, Ohtsuka M, Kato A, Yoshitomi H, Nozawa S, Furukawa K, Takeuchi D, Takayashiki T, Suda K, Igarashi T, Miyazaki M. Usefulness of intraoperative fluorescence imaging to evaluate local anatomy in hepatobiliary surgery. J Hepatobiliary Pancreat Surg 2008;15:508-514. [Crossref] [PubMed]
- Takahashi M, Ishikawa T, Higashidani K, Katoh H. SPY: an innovative intra-operative imaging system to evaluate graft patency during off-pump coronary artery bypass grafting. Interact Cardiovasc Thorac Surg 2004;3:479-483. [Crossref] [PubMed]
- Suzuki A, Fujiwara M, Mizukami T, Fukamizu H. Delayed distally-based super sural flap: evaluation by indocyanine green fluorescence angiography. J Plast Reconstr Aesthet Surg 2008;61:467-9. [Crossref] [PubMed]
- Yano A, Orihashi D, Yoshida Y, Kuriyama M. Near-infrared spectroscopy for monitoring free jejunal flap. J Plast Reconstr Aesthet Surg 2021;74:108-115. [Crossref] [PubMed]
- Kim MB, Ward DS, Cartwright CR, Kolano J, Chlebowski S, Henson LC. Estimation of jugular venous O2 saturation from cerebral oximetry or arterial O2 saturation during isocapnic hypoxia. J Clin Monit Comput 2000;16:191-9. [Crossref] [PubMed]
- Hongo K, Kobayashi S, Okudera H, Hokama M, Nakagawa F. Noninvasive cerebral optical spectroscopy: depth-resolved measurements of cerebral haemodynamics using indocyanine green. Neurol Res 1995;17:89-93. [Crossref] [PubMed]
- Orihashi K, Sueda T, Okada K, Imai K. Near-infrared spectroscopy for monitoring cerebral ischemia during selective cerebral perfusion. Eur J Cardiothorac Surg 2004;26:907-911. [Crossref] [PubMed]
- Olsson C, Thelin S. Regional cerebral saturation monitoring with near-infrared spectroscopy during selective antegrade cerebral perfusion: diagnostic performance and relationship to postoperative stroke. J Thorac Cardiovasc Surg 2006;131:371-9. [Crossref] [PubMed]
- Llewellyn-Bennett R, Wotton R, West D. Prophylactic flap coverage and the incidence of bronchopleural fistulae after pneumonectomy. Interact Cardiovasc Thorac Surg 2013;16:681-5. [Crossref] [PubMed]
- Di Maio M, Perrone F, Deschamps C, Rocco G. A meta-analysis of the impact of bronchial stump coverage on the risk of bronchopleural fistula after pneumonectomy. Eur J Cardiothorac Surg 2015;48:196-200. [Crossref] [PubMed]
- Taghavi S, Marta GM, Lang G, Seebacher G, Winkler G, Schmid K, Klepetko W. Bronchial stump coverage with a pedicled pericardial flap: an effective method for prevention of postpneumonectomy bronchopleural fistula. Ann Thorac Surg 2005;79:284-8. [Crossref] [PubMed]