Clinical and imaging predictors of impaired myocardial perfusion in symptomatic patients after percutaneous coronary intervention: insights from dynamic CT myocardial perfusion imaging
Introduction
Percutaneous coronary intervention (PCI) is a common procedure in treating obstructive coronary artery disease (CAD) (1,2). The main treatment aim of this invasive procedure is to restore the myocardial perfusion of the ischemic myocardium, which is subtended by coronary stenosis. According to a previous largescale observational study, patients with ischemic but viable myocardia were more likely to benefit from early revascularization (3). However, complete recovery of blood flow to the ischemic myocardium cannot be achieved in all patients and reduced myocardial perfusion with patent stents has been previously reported (4,5). To date, the clinical factors, which are associated with postprocedural myocardial perfusion recovery, remain unknown.
Dynamic computed tomography myocardial perfusion imaging (CT-MPI) is an emerging technique that allows full quantification of myocardial blood flow (MBF) and incorporates both functional and anatomical evaluation of CAD (6,7). The accuracy of dynamic CT-MPI derived parameters for diagnosing ischemic coronary stenosis or acute myocardial injury has been validated on the latest platform of dual source CT (8-10). However, only one previous study has employed this novel technique to detect reduced MBF after PCI without correlating this perfusion abnormality to preprocedural clinical and imaging characteristics (4). We hypothesized that different baseline clinical characteristics may contribute to the myocardial perfusion recovery after PCI and that this blood flow improvement can be quantified by dynamic CT-MPI. Therefore, the purpose of the current study was to investigate the relationship between baseline clinical characteristics and postprocedural myocardial perfusion as determined by dynamic CT-MPI.
Methods
Participant population
We reviewed the database in our institute between January 2017 and December 2019 for consecutive symptomatic post-PCI patients who underwent dynamic CT-MPI and coronary CT angiography (CCTA) for evaluation of suspected in-stent restenosis (ISR) or unrevascularized native lesions before staged PCI. Patients were retrospectively included if dynamic CT-MPI + CCTA revealed patent stents on previously revascularized lesions. The exclusion criteria were as follows: (I) dynamic CT-MPI + CCTA revealing the presence of ISR; or (II) patients with unassessable stents; or (III) patients with de novo obstructive lesions (defined as stenotic extent ≥50%) on stented vessels; (IV) patients with concomitant cardiomyopathy; (V) incomplete baseline clinical data; or (VI) a significantly impaired image quality on dynamic CT-MPI (Figure 1). The hospital ethics committee approved this study, and the need for written informed consent was waived due to its retrospective nature.
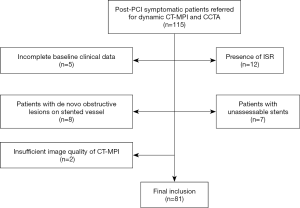
Scan protocol of dynamic CT-MPI + CCTA
A third-generation dual source CT (SOMATOM Force, Siemens Healthineers, Forchheim, Germany) was employed for all image acquisition. We used an integrated protocol which incorporated calcium score imaging, dynamic CT-MPI, and CCTA as previously reported (8). In brief, the calcium score was performed first to measure the calcification burden of epicardial vessels. A dynamic CT-MPI acquisition shuttle mode technique was triggered after 3 minutes of intravenous infusion of adenosine triphosphate (ATP) at 160 µg/kg/min. Nitroglycerin was given sublingually to all participants 5 minutes after dynamic CT-MPI, prior to the acquisition of CCTA which was performed using a prospective electrocardiogram (ECG)-triggered sequential technique. The details of scan parameters are given in the Appendix 1.
Image analysis of dynamic CT-MPI
The CT-MPI data were reconstructed using a dedicated kernel for reduction of iodine beam-hardening artifacts (Qr36) and analyzed using a commercially available CT-MPI software package (Myocardial perfusion analysis, VPCT body, Siemens Healthineers, Forchheim, Germany). Motion correction was applied for necessary CT studies to correct breathing-related misregistration of the left ventricle. Quantification of MBF was performed using a hybrid deconvolution algorithm (11,12).
For quantitative analysis, region of interest (ROI) was manually placed to sample the MBF on a segment base according to 17-segment model with exclusion of apical segments (13). The ROI was drawn to cover the whole area of suspected perfusion defect within the segment or to cover the whole segment when the perfusion defect was absent. The short axis view of the myocardial color-coded map was used for quantification of all segments.
The stented territories (supplied by stented vessel) and reference territories (supplied by native vessels without diameter stenosis ≥30%) were each determined according to the fusion images of coronary vasculature and perfusion map. The mean value of MBF was measured for each segment of both stented territories and reference territories. An MBF <100 mL/min/100 mL was used as the cutoff value to differentiate ischemic from nonischemic segments (8).
A pair of cardiovascular radiologists (with 10 and 6 years of experience in cardiac CT imaging) who were blinded to clinical histories independently analyzed all CT-MPI data. The mean values of MBF measured by the two observers were used for analysis.
Image analysis of CCTA
Axial images were reconstructed with smooth kennel (Bv 40, for evaluation of native coronary arteries) and sharp kernel (Bv 44, for evaluation of stents). Third-generation iterative reconstruction with different strength factors (strength 3 for smooth kernel data and strength 4 for sharp kernel data) was applied to all image datasets. The cardiac phase with the best image quality was manually determined and transferred to an offline workstation (SyngoVia, Siemens Healthineers, Forchheim, Germany) for further analyses.
Stent patency was assessed using sharp kernel image data and on reconstructed cross-sectional images. Binary ISR was considered as an in-stent neointimal proliferation with diameter stenosis ≥50% (14). Native coronary arteries with diameter ≥1.5 mm were also evaluated and obstructive lesions were defined as diameter stenosis ≥50%. The same two cardiovascular radiologists who were blinded to clinical histories independently analyzed all CCTA data. The presence of obstructive CAD or ISR was determined by consensus reading.
Baseline clinical characteristics
Baseline individual clinical characteristics were collected from the electronic medical record system by study investigators. Characteristics included patients’ symptoms [stable angina, unstable angina, and acute myocardial infarction (AMI)], conventional high-risk factors of CAD (hypertension, diabetes mellitus, hyperlipidemia, and smoking), and blood biochemical parameters, such as peak level of high-sensitivity cardiac troponin I (hs-cTnI), N-terminal pro-B-type natriuretic peptide (NT-pro-BNP), and high-sensitivity C-reactive protein (hs-CRP). The angiographic features of target lesion, including stenotic extent, thrombolysis in myocardial infarction (TIMI) flow grade, and lesion length, were also recorded.
Statistical analysis
Statistical analysis was performed with commercially available statistical software (MedCalc Statistical Software version 15.2.2, MedCalc Software BVBA, Ostend, Belgium). Qualitative or quantitative variables are expressed as mean ± standard deviation (SD), percentages, or median and interquartile range. Differences between normally distributed data [age, body mass index (BMI), apolipoprotein A to apolipoprotein B ratio (APOA/APOB ratio), total cholesterol, lesion length, and preprocedural TIMI flow grade] were compared using the Student’s t-test, whereas differences between data that were not normally distributed (hs-cTnI, NT-pro-BNP, NLR ratio, triglyceride, stenotic extent, systolic blood pressure, and diastolic blood pressure) were analyzed by the Mann-Whitney U test. The differences between categorical baseline variables (AMI and stent location) were analyzed by either chi-square test or Fisher’s exact test. Interobserver agreement of MBF measurement was assessed by intraclass correlation coefficient (ICC). We investigated the effects of different variables on post-PCI myocardial perfusion by univariate and multivariate logistic regression analyses. The variables that were predictors for reduced post-PCI myocardial perfusion were included in the multivariate logistic regression model. Receiver operating characteristic (ROC) curve analysis was performed by the DeLong method on baseline clinical and angiographic characteristics for identifying patients with reduced myocardial perfusion after revascularization. The area under curve (AUC) of each parameter was calculated. A two-tailed P value <0.05 was considered statistically significant.
Results
Clinical characteristics
A total of 115 post-PCI patients with dynamic CT-MPI and CCTA between 1 January 2017 and 31 December 2019 were retrospectively reviewed. A total of 12 patients were excluded due to the presence of ISR, and 5 patients were excluded because of incomplete preprocedural clinical data. A further 8 patients were excluded because of the presence of de novo obstructive lesions on stented vessels. In addition, 7 patients with unassessable stents and 2 patients with insufficient image quality of CT-MPI were also excluded (Figure 1). Finally, 81 participants with 96 stented vessels were included in the analysis. The mean effective doses of radiation for the whole integrated CT protocol (calcium score + dynamic CT-MPI + CCTA) was 4.89±1.14 (2.58–6.93) mSv when using 0.014 as the conversion factor. The mean interval between CT examinations and previous PCI was 11.2±7.4 months [6–30]. Clinical demographic details are displayed in Table 1.
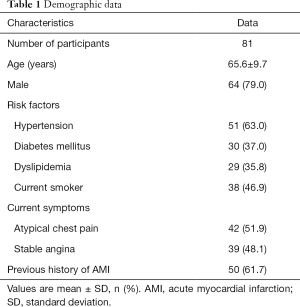
Full table
MBF of stented segments and reference segments
The interobserver agreement for measurement of MBF was good, as evidenced by the ICC of 0.987 (P<0.001). The mean MBF of all stented segments was 112.3±40.6 mL/100 mL/min, of which 187 segments were revealed to be ischemic (75.3±17.2 mL/100 mL/min) and 367 to be nonischemic (138.6±20.5 mL/100 mL/min). The mean MBF of all reference segments was 140.7±19.8 mL/100 mL/min. Overall, 49 stented vessels had reduced MBF within related territories, whereas 47 stented vessels had normal MBF.
Baseline clinical and invasive angiographic characteristics between stented territories with normal and reduced perfusion
Various baseline clinical characteristics were compared between participants with normal and reduced MBF of stented territories. According to the current results, peak levels of hs-cTnI, NT-Pro-BNP, hs-CRP, and glucose were significantly higher in participants with reduced MBF of stented territories (Table 2). Representative cases are displayed in Figures 2,3. The presence of AMI was also predominant in patients with decreased MBF after revascularization (77% vs. 39%, P=0.001). Other clinical parameters were not significantly different between the two subgroups (Table 2).
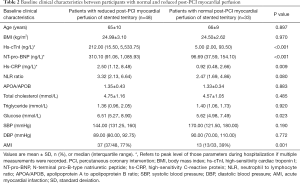
Full table
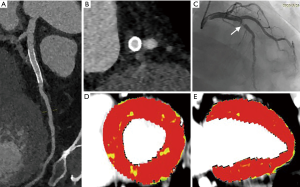
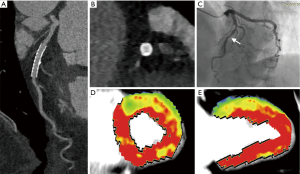
As for invasive angiographic characteristics, baseline stenotic extent was markedly higher in lesions with decreased post-PCI MBF while preprocedural TIMI flow grade was significantly lower. The lesion location and length were similarly distributed between two subgroups (Table 3).
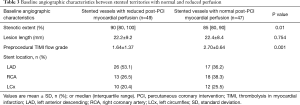
Full table
Univariate and multivariate analysis of predictors for decreased myocardial perfusion
Multiple clinical and angiographic parameters were included in the univariate analysis. As revealed by the current study, hs-cTnI, diabetes, NT-pro-BNP, hs-CRP, AMI, stenotic extent, and preprocedural TIMI flow grade were all associated with decreased myocardial perfusion after PCI in univariate analysis (Table 4). When these parameters were input into multivariate analysis, peak hs-cTnI level was the strongest predictor [adjusted hazard ratio (HR): 4.548, P=0.003] for decreased myocardial perfusion, followed by TIMI flow grade, AMI, stenotic extent, and NT-pro-BNP (Table 4). According to ROC curve analysis, stenotic extent and peak hs-cTnI level were the two best parameters for identifying patients with reduced myocardial perfusion after revascularization (Figure 4). When using the best cutoff values derived from ROC curve analysis, hs-cTnI, preprocedural stenotic extent, and TIMI flow grade were found to have high diagnostic accuracy for identifying patients with reduced MBF (Table 5).
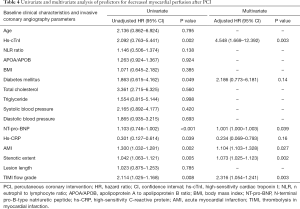
Full table
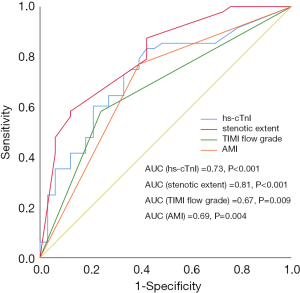

Full table
Discussion
The current study revealed that the baseline hs-cTnI peak level was the strongest predictor for decreased myocardial perfusion after revascularization, followed by preprocedural TIMI flow grade. In addition, a large proportion of patients with AMI did not have normal MBF even after successful revascularization.
Dynamic CT-MPI combined with CCTA is a novel noninvasive imaging modality for detection of reduced myocardial perfusion after revascularization. So far, only 1 previous study with 37 participants had investigated the prevalence of impaired perfusion in symptomatic patients with patent stents (4). Compared to that study, the current study focused more on the relationship between baseline characteristics and reduced MBF after revascularization. Our study confirmed a previous finding in which the incidence of myocardial ischemia with patent stents was relatively high in symptomatic patients. Using a significantly larger sample size, we further identified several baseline clinical and imaging characteristics which were able to predict impaired myocardial perfusion after PCI.
hs-cTnI is a routinely used laboratory biomarker which is highly associated with myocardial injury following AMI (15,16). In addition to AMI, the peak level of hs-cTnI also rises after PCI in patients without myocardial infarction if periprocedural myocardial injury occurs. This is not uncommon and typically caused by distal embolization during procedures (17). In these circumstances, despite the patent coronary stents, microvascular perfusion may be reduced in a substantial portion of the perfusion territory of the treated coronary artery because distal embolization could can abnormalities at the level of the microvasculature (18). Therefore, it is conceivable that among all baseline clinical characteristics, the peak level of hs-cTnI was the strongest predictor for decreased myocardial perfusion after revascularization.
As for angiographic features, the stenotic extent and preprocedural TIMI flow grade were also two independent predictors for decreased MBF of the stented territory after revascularization. This is in line with the finding of a previous 15O-water positron emission tomography study that indicated lower lesion complexity and lesser stenotic extent were significantly associated with better regional MBF recovery after revascularization (19). Moreover, pre-PCI TIMI flow grade has been proven to be inversely associated with myocardial injury and microvascular obstruction. It is therefore within expectations that TIMI flow grade was an independent predictor for reduced myocardial perfusion after revascularization.
The current study also demonstrated the potential clinical value of dynamic CT-MPI + CCTA as a “one-stop shop” imaging modality for the comprehensive evaluation of symptomatic post-PCI patients. Anatomical assessment of stent patency by either CCTA or invasive coronary angiography (ICA) is currently the standard method in post-PCI patients with recurrent symptoms (20). However, as revealed by the present study, more than half of the cohort with patent stents had reduced MBF within stented territory. This finding indicates that lumen evaluation might be inadequate for accurate diagnosis in symptomatic patients after revascularization. In contrast to CCTA, dynamic CT-MPI + CCTA is able to detect both anatomical and functional abnormality in one examination at a relatively low radiation dose (4.89 mSv), which makes it suitable in the current clinical scenario. Moreover, the baseline hs-cTnI peak level was found to be the strongest predictor for decreased myocardial perfusion after PCI. Thus, in clinical practice, patients with high baseline hs-cTnI peak level might be considered a high-risk population of impaired microvascular dysfunction after revascularization. Follow-up CT-MPI + CCTA is therefore preferred as the first-tier imaging modality for such patients with recurrent symptoms.
In spite of the above findings, the current study also had several limitations. First, only symptomatic post-PCI patients with patent stents were included due to the retrospective design. Asymptomatic post-PCI patients were not referred for dynamic CT-MPI + CCTA in our institute because of improper indications. Thus, it remains unclear whether similar findings can be revealed in the cohort of asymptomatic post-PCI patients. Also, the retrospective design of the current study led to heterogeneity of the population (patients with and without a history of AMI) and a limited sample size. Future prospective studies with larger sample sizes are warranted to eliminate the impact of confounding factors. In addition, the period range between PCI and follow-up CT scan was relatively wide in our study. This nonuniform time interval was another confounding factor that might have influenced the recovery of blood flow after PCI. Finally, cardiac MRI with late gadolinium enhancement was not available after PCI. Hence, it is not feasible to differentiate myocardial ischemia from scarring secondary to myocardial infarction. Finally, the baseline plaque features of target lesions were not evaluated, as preprocedural CCTA was not available in all participants. The CCTA has been recognized as a useful tool for high-risk plaque analysis (21). It may bear valuable information regarding the association between plaque characteristics and post-PCI myocardial perfusion.
In conclusion, the baseline hs-cTnI peak level was the strongest predictor for decreased myocardial perfusion after revascularization, followed by preprocedural TIMI flow grade. In addition, a large proportion of patients with AMI did not have fully recovered MBF even after successful revascularization.
Acknowledgments
Funding: This study was supported by the Science and Technology Foundation of the Health Commission of Guizhou province (gzwjkj 2020-1-176), International Exemplary Cooperation Base of Precision Imaging for Diagnosis and Treatment {QKHPTRC[2019]5803}, Medical Guidance Scientific Research Support Project of Shanghai Science and Technology Commission (19411965100) and Shanghai Municipal Education Commission-Gaofeng Clinical Medicine Grant Support (20161428).
Footnote
Conflicts of Interest: All authors have completed the ICMJE uniform disclosure form (available at http://dx.doi.org/10.21037/qims-20-977). Dr. HM received grant from Science and Technology Foundation of the Health Commission of Guizhou province (gzwjkj 2020-1-176). Dr. RW received grant from International Exemplary Cooperation Base of Precision Imaging for Diagnosis and Treatment {QKHPTRC[2019]5803}. Dr. JZ received grants from Medical Guidance Scientific Research Support Project of Shanghai Science and Technology Commission (Grant No. 19411965100) and Shanghai Municipal Education Commission-Gaofeng Clinical Medicine Grant Support (Grant No. 20161428). The other authors have no conflicts of interest to declare.
Ethical Statement: The hospital ethics committee approved this study, and the need for written informed consent was waived due to its retrospective nature.
Open Access Statement: This is an Open Access article distributed in accordance with the Creative Commons Attribution-NonCommercial-NoDerivs 4.0 International License (CC BY-NC-ND 4.0), which permits the non-commercial replication and distribution of the article with the strict proviso that no changes or edits are made and the original work is properly cited (including links to both the formal publication through the relevant DOI and the license). See: https://creativecommons.org/licenses/by-nc-nd/4.0/.
References
- Sousa JE, Serruys PW, Costa MA. New frontiers in cardiology: drug-eluting stents: Part I. Circulation 2003;107:2274-9. [Crossref] [PubMed]
- Sousa JE, Serruys PW, Costa MA. New frontiers in cardiology: drug-eluting stents: Part II. Circulation 2003;107:2383-9. [Crossref] [PubMed]
- Hachamovitch R, Rozanski A, Shaw LJ, Stone GW, Thomson LE, Friedman JD, Hayes SW, Cohen I, Germano G, Berman DS. Impact of ischaemia and scar on the therapeutic benefit derived from myocardial revascularization vs. medical therapy among patients undergoing stress-rest myocardial perfusion scintigraphy. Eur Heart J 2011;32:1012-24. [Crossref] [PubMed]
- Li Y, Yuan M, Yu M, Lu Z, Shen C, Wang Y, Lu B, Zhang J. Prevalence of decreased myocardial blood flow in symptomatic patients with patent coronary stents: insights from low-dose dynamic CT myocardial perfusion imaging. Korean J Radiol 2019;20:621-30. [Crossref] [PubMed]
- Schumacher SP, Stuijfzand WJ, Driessen RS, van Diemen PA, Bom MJ, Everaars H, Kockx M, Raijmakers PG, Boellaard R, van de Ven PM, van Rossum AC, Opolski MP, Nap A, Knaapen P. Impact of specific crossing techniques in chronic total occlusion percutaneous coronary intervention on recovery of absolute myocardial perfusion. Circ Cardiovasc Interv 2019;12:e008064 [Crossref] [PubMed]
- Alessio AM, Bindschadler M, Busey JM, Shuman WP, Caldwell JH, Branch KR. Accuracy of myocardial blood flow estimation from dynamic contrast-enhanced cardiac CT compared with PET. Circ Cardiovasc Imaging 2019;12:e008323 [Crossref] [PubMed]
- Yu M, Shen C, Dai X, Lu Z, Wang Y, Lu B, Zhang J. Clinical outcomes of dynamic computed tomography myocardial perfusion imaging combined with coronary computed tomography angiography versus coronary computed tomography angiography-guided strategy. Circ Cardiovasc Imaging 2020;13:e009775 [Crossref] [PubMed]
- Li Y, Yu M, Dai X, Lu Z, Shen C, Wang Y, Lu B, Zhang J. Detection of hemodynamically significant coronary stenosis: CT myocardial perfusion versus machine learning CT fractional flow reserve. Radiology 2019;293:305-14. [Crossref] [PubMed]
- Yu M, Chen X, Dai X, Pan J, Wang Y, Lu B, Zhang J. The value of low-dose dynamic myocardial perfusion CT for accurate evaluation of microvascular obstruction in patients with acute myocardial infarction. AJR Am J Roentgenol 2019;213:798-806. [Crossref] [PubMed]
- Pan J, Yuan M, Yu M, Gao Y, Shen C, Wang Y, Lu B, Zhang J. Myocardial blood flow quantified by low-dose dynamic CT myocardial perfusion imaging is associated with peak troponin level and impaired left ventricle function in patients with ST-elevated myocardial infarction. Korean J Radiol 2019;20:709-18. [Crossref] [PubMed]
- Bamberg F, Klotz E, Flohr T, Becker A, Becker CR, Schmidt B, Wintersperger BJ, Reiser MF, Nikolaou K. Dynamic myocardial stress perfusion imaging using fast dual-source CT with alternating table positions: initial experience. Eur Radiol 2010;20:1168-73. [Crossref] [PubMed]
- Rossi A, Merkus D, Klotz E, Mollet N, de Feyter PJ, Krestin GP. Stress myocardial perfusion: imaging with multidetector CT. Radiology 2014;270:25-46. [Crossref] [PubMed]
- Cerqueira MD, Weissman NJ, Dilsizian V, Jacobs AK, Kaul S, Laskey WK, Pennell DJ, Rumberger JA, Ryan T, Verani MSAmerican Heart Association Writing Group on Myocardial Segmentation and Registration for Cardiac Imaging. Standardized myocardial segmentation and nomenclature for tomographic imaging of the heart. A statement for healthcare professionals from the Cardiac Imaging Committee of the Council on Clinical Cardiology of the American Heart Association. Circulation 2002;105:539-42. [Crossref] [PubMed]
- Li Y, Yu M, Li W, Lu Z, Wei M, Zhang J. Third generation dual-source CT enables accurate diagnosis of coronary restenosis in all size stents with low radiation dose and preserved image quality. Eur Radiol 2018;28:2647-54. [Crossref] [PubMed]
- Chia S, Senatore F, Raffel OC, Lee H, Wackers FJ, Jang IK. Utility of cardiac biomarkers in predicting infarct size, left ventricular function, and clinical outcome after primary percutaneous coronary intervention for ST-segment elevation myocardial infarction. JACC Cardiovasc Interv 2008;1:415-23. [Crossref] [PubMed]
- Hassan AK, Bergheanu SC, Hasan-Ali H, Liem SS, van der Laarse A, Wolterbeek R, Atsma DE, Schalij MJ, Jukema JW. Usefulness of peak troponin-T to predict infarct size and long-term outcome in patients with first acute myocardial infarction after primary percutaneous coronary intervention. Am J Cardiol 2009;103:779-84. [Crossref] [PubMed]
- Balian V, Galli M, Marcassa C, Cecchin G, Child M, Barlocco F, Petrucci E, Filippini G, Michi R, Onofri M. Intracoronary ST-segment shift soon after elective percutaneous coronary intervention accurately predicts periprocedural myocardial injury. Circulation 2006;114:1948-54. [Crossref] [PubMed]
- Jaffe R, Dick A, Strauss BH. Prevention and treatment of microvascular obstruction-related myocardial injury and coronary no-reflow following percutaneous coronary intervention: a systematic approach. JACC Cardiovasc Interv 2010;3:695-704. [Crossref] [PubMed]
- Aikawa T, Naya M, Koyanagawa K, Manabe O, Obara M, Magota K, Oyama-Manabe N, Tamaki N, Anzai T. Improved regional myocardial blood flow and flow reserve after coronary revascularization as assessed by serial 15O-water positron emission tomography/computed tomography. Eur Heart J Cardiovasc Imaging 2020;21:36-46. [Crossref] [PubMed]
- Hickethier T, Wenning J, Bratke G, Maintz D, Michels G, Bunck AC. Evaluation of soft-plaque stenoses in coronary artery stents using conventional and monoenergetic images: first in-vitro experience and comparison of two different dual-energy techniques. Quant Imaging Med Surg 2020;10:612-23. [Crossref] [PubMed]
- Yuan M, Wu H, Li R, Yu M, Dai X, Zhang J. The value of quantified plaque analysis by dual-source coronary CT angiography to detect vulnerable plaques: a comparison study with intravascular ultrasound. Quant Imaging Med Surg 2020;10:668-77. [Crossref] [PubMed]