Impacts of biological and procedural factors on semiquantification uptake value of liver in fluorine-18 fluorodeoxyglucose positron emission tomography/computed tomography imaging
Introduction
Integrated positron emission tomography/computed tomography (PET/CT) is becoming an important tool for clinical investigation with increase clinical utilization. The utilisation of this integrated imaging modality in cancer diagnosis has been well documented (1) as it offers several advantages over conventional scintigraphic techniques in terms of functional and morphological interpretation in a single imaging session, faster examination, greater topographic resolution and more accurate lesion localization (2-5). Both systems complement each other in improving diagnostic capabilities by enabling accurate lesion localization unlike standing alone for PET or CT system.
The radiotracer most frequently used in PET for oncology application is fluorine-18 fluoro-2-deoxy-D-glucose (18F-FDG). 18F-FDG PET is a functional imaging modality which reflects cellular glucose metabolism (6,7). In clinical practice, 18F-FDG PET images are analysed either qualitatively using visual comparison of the glucose metabolism in lesions in relative to normal tissues, or semiquantitatively using standardized uptake values (SUV) (8). Accumulation and trapping of fluorodeoxyglucose (FDG) allow the visualization of increased uptake in most malignant cells compared to normal cells. However, increased FDG uptake is not limited to malignant tissue alone (9-13) but in inflammatory lesions as well (14,15).
The FDG uptake as manifested by SUV could be affected by multiple factors such as weight, plasma glucose level, length of uptake period, partial volume effects and recovery coefficient (16,17). In PET/CT imaging, liver FDG uptake is commonly used as the reference standard for diagnosis (18,19), therapy assessment (20), prognosis (21) and quality control (22). It has been reported that age (23), blood glucose level (24), body mass index (BMI) (25), incubation time (26) and hepatic steatosis (27) influence the liver FDG uptake. The association between states of overweight and obesity with non-alcoholic fatty liver has been reported (28-30) in which alteration in normal glucose metabolism was found in metabolic syndrome. The intensity of physiological FDG uptake in the liver varies. It is important to be familiar with the varying degree of FDG accumulation that represents normal distribution and physiological changes, before attempting to interpret whole-body PET imaging for abnormality detection.
The aim of this study was to evaluate the impacts of biological and procedural-related factors that influence the semiquantification value of liver involving patients undergoing whole body 18F-FDG PET/CT for oncological disease using maximum SUV (SUVmax). Then the study was extended to determine the difference of liver SUVmax among four BMI groups of patients.
Materials and methods
Patients
This study includes the analysis of 18F-FDG PET/CT images from 51 patients who referred for 18F-FDG PET/CT whole body imaging in our centre from January 2012 to September 2013. Patients presenting metabolically FDG-avid in the liver in the context of primary or metastatic involvement were excluded from the study. Demographic data and biological parameters including age (years), weight (kg), height (cm), fasting blood glucose (mmol/L) and injected dose of FDG (millicurie), were collected from patient records. Patient consents were acquired as they were undergoing the PET/CT scan. Ethics approval was obtained from our local Medical Research Ethics Committee.
The BMI was calculated according to the formula: weight/height squared, and the nutritional status of the patients was classified according to the classification of World Health Organization (31). According to the value of BMI obtained, the patients were grouped into four categories of nutritional status; patients were considered to have underweight with a BMI less than 18.5, normal or adequate weight was considered in patients with BMI values between 18.5 to 25, overweight was considered with BMI values between 25 to 30, and patients with BMI more than 30 was considered as obese.
Patient preparation
All patients were instructed to fast for at least 6 hours prior to scanning session and only oral hydration with glucose-free water was allowed. Fasting blood glucose was checked in all patients. Dilute gastrografin solution (sodium meglumine diatrizoate; BerliMed S.A., Madrid, Spain) was given orally to the patients in three divided doses before administration of the radiopharmaceutical agent. Mean of 327.69±35.63 megabecquerel (MBq) of FDG was injected intravenously. All patients were put to rest in a special uptake room for an average of 84.05±59.57 (ranging from 30-282) minutes and empty the bladder before underwent the PET/CT imaging session.
PET/CT imaging protocol
Image acquisition was performed using an integrated Siemens Biograph 64 TruePoint PET/CT system (Siemens Medical Solutions USA Inc.) consisting of a PET scanner with lutetium oxyorthosilicate (LSO) crystals detector and a 64-multi detector CT scanner (MDCT). A scout view was performed in cranio-caudal direction to plan the study and then followed by non-contrast enhanced CT (NECT) protocol in caudo-cranial direction for the purpose of anatomical localisation and attenuation correction. Diagnostic protocol was then carried out with intravenous (IV) injection of non-ionic contrast, iohexol (omnipaque 350 mg/mL, GE Healthcare, Shanghai, China) [84.07 (mean; ranging from 50-110) mL] using dual head automatic pressure injector (Mallinckrodt, MO, USA) with flow rate at 2.5 mL per second and followed by 20 mL saline flush. Subsequently the contrast-enhanced CT scan (CECT) acquisition started in caudo-cranial direction with 80 seconds delay, ensuring optimal IV contrast in the circulation and tissue enhancement. Afterwards, a PET scan was acquired contemporaneously at 2 minutes per bed position using a three-dimensional acquisition mode. The total duration of PET/CT examination was about 23 minutes, with approximately 8 minutes to complete two CT scans and about 15 minutes to acquire PET emission data.
Image reconstruction
NECT and CECT data were reconstructed with 5 mm slice thickness in the axial plane and increment of 3 mm. PET images were reconstructed by using TrueX reconstruction algorithm with three iterations and 21 subsets using Gaussian filter with full width at half maximum (FWHM) of 4 mm. The CECT datasets were employed for attenuation correction with the same set of PET images. The NECT, CECT and attenuation corrected PET images dataset were copied into Apple Macbook Pro laptop (Apple Inc, California, USA) and displayed in transaxial, coronal and sagittal planes using OsiriX imaging software DICOM viewer 32-bit version (Pixmeo, Geneva, Switzerland) along with maximum intensity projection (MIP) images.
Image analysis
Three oval regions of interests (ROIs) with diameter of 1.00±0.010 cm2 were drawn over the right lobe of the liver at the segment VII to quantify the liver SUVmax (Figure 1). The intensity of FDG uptake in the tissues can be assessed visually using four points scale of intensity with reference to the liver uptake. This scale included grade 0= no uptake; grade 1= slight uptake (tissue uptake lower than liver uptake); grade 2= moderate uptake (tissue uptake similar to liver uptake); and grade 3= intense uptake (tissue uptake higher than liver uptake) (32). We have utilised the SUVmax values for quantification of FDG uptake because maximum pixel value is the most preference in PET/CT study (15). The average values from those three ROIs from each tissue were calculated.
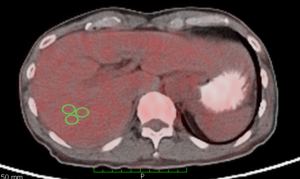
Statistical analysis
The descriptive statistics were presented as mean ± standard deviation (SD) for continuous variables including age, BMI, blood glucose level, incubation period and FDG dose. The differences between mean of FDG uptake among the BMI groups were evaluated using analysis of variance (ANOVA). The association between the SUVmax of liver and those factors were analysed by Pearson coefficients of correlation. Multivariate regression models were established to determine the best predictors of liver SUVmax among those factors. Multicollinearity between covariates was tested and none was identified. Then ANOVA was performed to determine the significance difference of the mean SUVmax of liver among the four categories of BMI. All hypothesis tests were two sided with a significant level of 0.05. The Statistical Package for Social Sciences program for Windows 21.0 (SPSS 21) (IBM Corp, Somers, New York, USA) was used for the statistical analysis.
Results
The analysis included 51 patients with 28 males and 23 females. The demographic data of patients are shown in Table 1. A statistically significant positive association was found with the SUVmax of liver and BMI, however, statistically significant negative association was observed between the semiquantification value and incubation period (Table 2). After adjusting for all other covariates in the final model of multivariate regression analysis, it was demonstrated that BMI and incubation period were significantly associated with SUVmax of liver (Table 3). These covariates were accounted for 29.6% of the liver SUVmax variance. BMI had the strongest association as marked by higher value of adjusted standardised beta coefficient as compared to the incubation period (Table 3). The mean SUVmax of liver in four BMI groups are shown in Table 4 and they were significantly different among those groups (P<0.05).

Full table
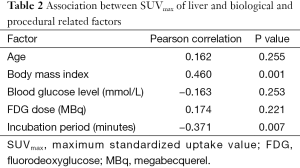
Full table
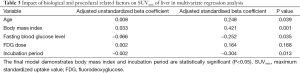
Full table
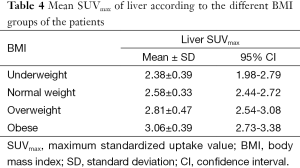
Full table
Discussion
Some physiological FDG uptake can cause misinterpretation of a PET scan; as a result it may lead to false-positive or false-negative interpretation, hence reducing the accuracy of the technique (33-36). Several factors contributing to physiological variation in FDG distribution have been reported (37-39). The variations in liver concentration of 18F-FDG in relation to BMI (25) and age (23) have been documented previously. A correlation study between liver and different BMI groups was reported, but it was limited to fatty liver as study population and only involved three BMI groups (40).
Our investigation demonstrated that 18F-FDG accumulation in liver was significantly higher in patients with increased BMI. This is likely due to very little fat accumulation in a fasting state and thus higher FDG uptake is characterized by non-fatty tissue (41). In the final model of regression analysis, BMI showed significant effect on the SUVmax of liver when adjusted for all other covariates. Moreover, the mean SUV of liver for each BMI group of patients was lower than those obtained by Batallés and his co-workers (42). This discrepancy might be due to variations in shape and size of the patients, method of ROI measurement and different type of scanner and workstation setting used. In contrast to the prior studies, we adjusted for age, blood glucose level, FDG dose and incubation period when we established the impact of BMI on the FDG uptake of liver. Other clinical and biological factors which were excluded from this study such as blood lipid profile (25), hepatic steatosis (43), diabetic status (44) and insulin (44) have been reported to have a significant effect on liver FDG uptake.
Obesity is associated with an increase in plasma levels of inflammatory cytokines such as tumour necrosis factor alpha (TNF-α) and interleukins-6 (IL-6) (45). Kupffer cells are residents macrophages distributed along the liver sinusoid (46). The similar cytokines including TNF-α and IL-6 are secreted by these Kupffer cells (47). Bioactive molecules generated by Kupffer cells and liver endothelial cells in response to varied stimuli have the capacity to contribute to the regulation of hepatic metabolism. Altered long-term expression of liver metabolic enzymes by TNF-α and IL-6 may be critical in the transition to the chronic inflammatory state (46). The sites of accumulation of 18F-FDG in infectious lesions are considered as secretory macrophages of these proinflammatory substances (48,49). It is thought that the areas of greatest FDG-avid usually observed in obese patients are due to inflammatory response of this chronically altered parenchyma which results to increase in the hepatic SUV (43). This present findings might explain strong correlation of physiological FDG uptake of liver with BMI is likely due to inflammatory state of the liver present in obese patients.
Incubation or uptake period is another factor which significantly affects the physiological uptake of liver. Longer incubation period tends to reduce the physiological FDG uptake of liver as signified by negative correlation in the Pearson correlation analysis and negative value of standardized beta coefficient in the multilinear regression analysis. It is likely increased FDG uptake of tumour and decreased uptake of background with longer FDG uptake time in PET/CT study (41). Application of dual time point imaging has made use the benefits of comparison between two time points study for assessment of tumour uptake with relative uptake period (50,51). It has been reported by a recent study that FDG uptake period could affect the liver SUV corrected for lean body mass at dual-phase FDG PET/CT (52).
On the contrary, this study showed fasting blood glucose level had no significant impacts on the liver SUVmax when adjusted for all other covariates. Our findings are contradicted with previous studies on the impact of blood glucose factor (24,41). Contrary to the prior studies, we adjusted for age, BMI, FDG dose and incubation period when we established the effect of fasting blood glucose and liver FDG uptake. We noted that mean SUV normalised to lean body mass was utilized in the study by Malladi et al. (41), otherwise maximum SUV normalised to body weight was employed in this study. However, our findings are in accordance with a study by Büsing et al. (44) and Kuruva et al. (53). Busing and his co-workers reported a significant impact of blood glucose level on 18F-FDG uptake was only observed in the organ consuming high glucose metabolism such as brain, whereas organs less consuming glucose such as liver and spleen showed insignificant effect (44).
Furthermore, this study also found the liver FDG uptake was not affected by age and FDG dose as well when adjusted for all other covariates. These findings are contradicted with the study previously which reported significant effect of age on the liver FDG uptake (23,41). FDG dose can affect the FDG uptake of liver, but it has only minimal effect compared with the other covariates, as it was applied within the range of injected doses for clinical studies (41).
This study did have some limitations. Only a small number of patients were available in the underweight and obese groups as compared to the other two BMI groups. Additionally, we did not investigate all the possible factors that could affect the liver FDG uptake such as hepatic steatosis, diabetic status, abnormal lipid profile and liver function abnormalities which some were excluded and not available in this study.
Conclusions
BMI and incubation period significantly affect the physiological FDG uptake of liver which accounted for 29.6% of the liver SUVmax variance. These impacts demonstrated a progressive increase in the semiquantification value with increasing BMI but progressive decrease with increasing incubation period. Higher impact was observed in BMI as compared to incubation period after adjustment for all other covariates. As physiological FDG uptake of liver was significantly different among varied BMI groups, it would be recommended to use different cut-off value of liver SUVmax as a reference standard for different BMI of patients in interpretation of whole body PET imaging.
Acknowledgements
The authors would like to acknowledge the Research Management Centre of Universiti Putra Malaysia for the funding of Research University Grant Scheme (RUGS) grant (04-02-12-1748RU).
Footnote
Conflicts of Interest: The authors have no conflicts of interest to declare.
References
- Townsend DW. Positron emission tomography/computed tomography. Semin Nucl Med 2008;38:152-66. [PubMed]
- Beyer T, Antoch G, Müller S, Egelhof T, Freudenberg LS, Debatin J, Bockisch A. Acquisition protocol considerations for combined PET/CT imaging. J Nucl Med 2004;45 Suppl 1:25S-35S. [PubMed]
- Nakamoto Y, Chin BB, Kraitchman DL, Lawler LP, Marshall LT, Wahl RL. Effects of nonionic intravenous contrast agents at PET/CT imaging: phantom and canine studies. Radiology 2003;227:817-24. [PubMed]
- Kinahan PE, Townsend DW, Beyer T, Sashin D. Attenuation correction for a combined 3D PET/CT scanner. Med Phys 1998;25:2046-53. [PubMed]
- An YS, Sheen SS, Oh YJ, Hwang SC, Yoon JK. Nonionic intravenous contrast agent does not cause clinically significant artifacts to 18F-FDG PET/CT in patients with lung cancer. Ann Nucl Med 2007;21:585-92. [PubMed]
- Răileanu I, Rusu V, Stefănescu C, Cinotti L, Hountis D. 18F FDG PET-Applications in Oncology. Rev Med Chir Soc Med Nat Iasi 2002;106:14-23. [PubMed]
- Son HB, Han CJ, Kim BI, Kim J, Jeong SH, Kim YC, Lee JO, Choi CY, Im SM. Evaluation of various hepatic lesions with positron emission tomography. Taehan Kan Hakhoe Chi 2002;8:472-80. [PubMed]
- Hadiprodjo D, Ryan T, Truong MT, Mercier G, Subramaniam RM. Parotid gland tumors: preliminary data for the value of FDG PET/CT diagnostic parameters. AJR Am J Roentgenol 2012;198:W185-90. [PubMed]
- Truong MT, Pan T, Erasmus JJ. Pitfalls in integrated CT-PET of the thorax: implications in oncologic imaging. J Thorac Imaging 2006;21:111-22. [PubMed]
- Gorospe L, Raman S, Echeveste J, Avril N, Herrero Y, Herna Ndez S. Whole-body PET/CT: spectrum of physiological variants, artifacts and interpretative pitfalls in cancer patients. Nucl Med Commun 2005;26:671-87. [PubMed]
- Cook GJ. Pitfalls in PET/CT interpretation. Q J Nucl Med Mol Imaging 2007;51:235-43. [PubMed]
- Cook GJ, Wegner EA, Fogelman I. Pitfalls and artifacts in 18FDG PET and PET/CT oncologic imaging. Semin Nucl Med 2004;34:122-33. [PubMed]
- Lin CY, Ding HJ, Liu CS, Chen YK, Lin CC, Kao CH. Correlation between the intensity of breast FDG uptake and menstrual cycle. Acad Radiol 2007;14:940-4. [PubMed]
- Cook GJ, Fogelman I, Maisey MN. Normal physiological and benign pathological variants of 18-fluoro-2-deoxyglucose positron-emission tomography scanning: potential for error in interpretation. Semin Nucl Med 1996;26:308-14. [PubMed]
- Ichiya Y, Kuwabara Y, Sasaki M, Yoshida T, Akashi Y, Murayama S, Nakamura K, Fukumura T, Masuda K. FDG-PET in infectious lesions: The detection and assessment of lesion activity. Ann Nucl Med 1996;10:185-91. [PubMed]
- Boellaard R, Krak NC, Hoekstra OS, Lammertsma AA. Effects of noise, image resolution, and ROI definition on the accuracy of standard uptake values: a simulation study. J Nucl Med 2004;45:1519-27. [PubMed]
- Boellaard R. Standards for PET image acquisition and quantitative data analysis. J Nucl Med 2009;50 Suppl 1:11S-20S. [PubMed]
- Perri M, Erba P, Volterrani D, Guidoccio F, Lazzeri E, Caramella D, Mariani G. Adrenal masses in patients with cancer: PET/CT characterization with combined CT histogram and standardized uptake value PET analysis. AJR Am J Roentgenol 2011;197:209-16. [PubMed]
- Ozcan Kara P, Kara T, Kara Gedik G, Kara F, Sahin O, Ceylan Gunay E, Sari O. The role of fluorodeoxyglucose-positron emission tomography/computed tomography in differentiating between benign and malignant adrenal lesions. Nucl Med Commun 2011;32:106-12. [PubMed]
- Juweid ME, Stroobants S, Hoekstra OS, Mottaghy FM, Dietlein M, Guermazi A, Wiseman GA, Kostakoglu L, Scheidhauer K, Buck A, Naumann R, Spaepen K, Hicks RJ, Weber WA, Reske SN, Schwaiger M, Schwartz LH, Zijlstra JM, Siegel BA, Cheson BD; Imaging Subcommittee of International Harmonization Project in Lymphoma. Use of positron emission tomography for response assessment of lymphoma: consensus of the Imaging Subcommittee of International Harmonization Project in Lymphoma. J Clin Oncol 2007;25:571-8. [PubMed]
- Shiono S, Abiko M, Okazaki T, Chiba M, Yabuki H, Sato T. Positron emission tomography for predicting recurrence in stage I lung adenocarcinoma: standardized uptake value corrected by mean liver standardized uptake value. Eur J Cardiothorac Surg 2011;40:1165-9. [PubMed]
- Wahl RL, Jacene H, Kasamon Y, Lodge MA. From RECIST to PERCIST: Evolving Considerations for PET response criteria in solid tumors. J Nucl Med 2009;50 Suppl 1:122S-50S. [PubMed]
- Lin CY, Ding HJ, Lin CC, Chen CC, Sun SS, Kao CH. Impact of age on FDG uptake in the liver on PET scan. Clin Imaging 2010;34:348-50. [PubMed]
- Kubota K, Watanabe H, Murata Y, Yukihiro M, Ito K, Morooka M, Minamimoto R, Hori A, Shibuya H. Effects of blood glucose level on FDG uptake by liver: a FDG-PET/CT study. Nucl Med Biol 2011;38:347-51. [PubMed]
- Kamimura K, Nagamachi S, Wakamatsu H, Higashi R, Ogita M, Ueno S, Fujita S, Umemura Y, Fujimoto T, Nakajo M. Associations between liver (18)F fluoro-2-deoxy-D-glucose accumulation and various clinical parameters in a Japanese population: influence of the metabolic syndrome. Ann Nucl Med 2010;24:157-61. [PubMed]
- Basu S, Kung J, Houseni M, Zhuang H, Tidmarsh GF, Alavi A. Temporal profile of fluorodeoxyglucose uptake in malignant lesions and normal organs over extended time periods in patients with lung carcinoma: implications for its utilization in assessing malignant lesions. Q J Nucl Med Mol Imaging 2009;53:9-19. [PubMed]
- Abikhzer G, Alabed YZ, Azoulay L, Assayag J, Rush C. Altered hepatic metabolic activity in patients with hepatic steatosis on FDG PET/CT. AJR Am J Roentgenol 2011;196:176-80. [PubMed]
- Kahn R, Buse J, Ferrannini E, Stern M; American Diabetes Association; European Association for the Study of Diabetes. The metabolic syndrome: time for a critical appraisal: joint statement from the American Diabetes Association and the European Association for the Study of Diabetes. Diabetes Care 2005;28:2289-304. [PubMed]
- Hamaguchi M, Kojima T, Takeda N, Nakagawa T, Taniguchi H, Fujii K, Omatsu T, Nakajima T, Sarui H, Shimazaki M, Kato T, Okuda J, Ida K. The metabolic syndrome as a predictor of nonalcoholic fatty liver disease. Ann Intern Med 2005;143:722-8. [PubMed]
- Kim YH, Kim JY, Jang SJ, Chung HW, Jang KS, Paik SS, Song SY, Choi YY. F-18 FDG uptake in focal fatty infiltration of liver mimicking hepatic malignancy on PET/CT images. Clin Nucl Med 2011;36:1146-8. [PubMed]
- Obesity: preventing and managing the global epidemic. Report of a WHO Consultation (WHO Technical Report Series 894). World Health Organization 2000:252.
- Arnaud L, Haroche J, Malek Z, Archambaud F, Gambotti L, Grimon G, Kas A, Costedoat-Chalumeau N, Cacoub P, Toledano D, Cluzel P, Piette JC, Amoura Z. Is (18)F-fluorodeoxyglucose positron emission tomography scanning a reliable way to assess disease activity in Takayasu arteritis? Arthritis Rheum 2009;60:1193-200. [PubMed]
- Dong MJ, Lin XT, Zhao J, Guan YH, Zuo CT, Chen X, Dai JZ, Jiang BD. Malignant tumor with false negative 18F-FDG PET image. Zhonghua Zhong Liu Za Zhi 2006;28:713-7. [PubMed]
- Mahmood S, Martinez de Llano SR. Paget disease of the humerus mimicking metastatic disease in a patient with metastatic malignant mesothelioma on whole body F-18 FDG PET/CT. Clin Nucl Med 2008;33:510-2. [PubMed]
- Acar C, Akkas BE, Sen I, Sozen S, Kitapci MT. False positive 18F-FDG PET scan in adrenal oncocytoma. Urol Int 2008;80:444-7. [PubMed]
- Roarke MC, Nguyen BD, Pockaj BA. Desmoplastic melanoma: true positive and false negative findings on F-18 FDG-PET/CT. Clin Nucl Med 2008;33:562-4. [PubMed]
- Thie JA, Hubner KF, Isidoro FP, Smith GT. A weight index for the standardized uptake value in 2-deoxy-2-[F-18]fluoro-D-glucose-positron emission tomography. Mol Imaging Biol 2007;9:91-8. [PubMed]
- Kitajima K, Murakami K, Yamasaki E, Kaji Y, Sugimura K. Standardized uptake values of uterine leiomyoma with 18F-FDG PET/CT: variation with age, size, degeneration, and contrast enhancement on MRI. Ann Nucl Med 2008;22:505-12. [PubMed]
- Chin BB, Green ED, Turkington TG, Hawk TC, Coleman RE. Increasing uptake time in FDG-PET: standardized uptake values in normal tissues at 1 versus 3 h. Mol Imaging Biol 2009;11:118-22. [PubMed]
- Lin CY, Lin WY, Lin CC, Shih CM, Jeng LB, Kao CH. The negative impact of fatty liver on maximum standard uptake value of liver on FDG PET. Clin Imaging 2011;35:437-41. [PubMed]
- Malladi A, Viner M, Jackson T, Mercier G, Subramaniam RM. PET/CT mediastinal and liver FDG uptake: effects of biological and procedural factors. J Med Imaging Radiat Oncol 2013;57:169-75. [PubMed]
- Batallés SM, Villavicencio RL, Quaranta A, Burgos L, Trezzo S, Staffieri R, Pezzotto SM. Variations of the hepatic SUV in relation to the body mass index in whole body PET-CT studies. Rev Esp Med Nucl Imagen Mol 2013;32:26-32. [PubMed]
- Abele JT, Fung CI. Effect of hepatic steatosis on liver FDG uptake measured in mean standard uptake values. Radiology 2010;254:917-24. [PubMed]
- Büsing KA, Schönberg SO, Brade J, Wasser K. Impact of blood glucose, diabetes, insulin, and obesity on standardized uptake values in tumors and healthy organs on 18F-FDG PET/CT. Nucl Med Biol 2013;40:206-13. [PubMed]
- Smith SR, Ravussin E. Emerging paradigms for understanding fatness and diabetes risk. Curr Diab Rep 2002;2:223-30. [PubMed]
- Wardle EN. Kupffer cells and their function. Liver 1987;7:63-75. [PubMed]
- Brass EP, Vetter WH. Interleukin-6, but not tumour necrosis factor-alpha, increases lipogenesis in rat hepatocyte primary cultures. Biochem J 1994;301:193-7. [PubMed]
- West J, Morton DJ, Esmann V, Stjernholm RL. Carbohydrate metabolism in leukocytes. 8. Metabolic activities of the macrophage. Arch Biochem Biophys 1968;124:85-90. [PubMed]
- Kubota R, Yamada S, Kubota K, Ishiwata K, Tamahashi N, Ido T. Intratumoral distribution of fluorine-18-fluorodeoxyglucose in vivo: high accumulation in macrophages and granulation tissues studied by microautoradiography. J Nucl Med 1992;33:1972-80. [PubMed]
- Dirisamer A, Halpern BS, Schima W, Heinisch M, Wolf F, Beheshti M, Dirisamer F, Weber M, Langsteger W. Dual-time-point FDG-PET/CT for the detection of hepatic metastases. Mol Imaging Biol 2008;10:335-40. [PubMed]
- Hu M, Han A, Xing L, Yang W, Fu Z, Huang C, Zhang P, Kong L, Yu J. Value of dual-time-point FDG PET/CT for mediastinal nodal staging in non-small-cell lung cancer patients with lung comorbidity. Clin Nucl Med 2011;36:429-33. [PubMed]
- Chirindel A, Alluri KC, Tahari AK, Chaudhry M, Wahl RL, Lodge MA, Subramaniam RM. Liver standardized uptake value corrected for lean body mass at FDG PET/CT: effect of FDG uptake time. Clin Nucl Med 2015;40:e17-22. [PubMed]
- Kuruva M, Mittal BR, Abrar ML, Kashyap R, Bhattacharya A. Multivariate analysis of various factors affecting background liver and mediastinal standardized uptake values. Indian J Nucl Med 2012;27:20-3. [PubMed]